MIT Technology Review
- Newsletters
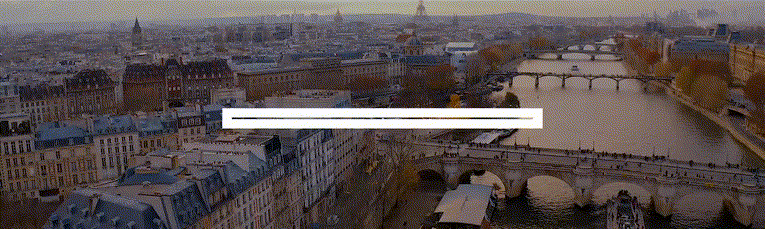
NASA wants to use the sun to power future deep space missions
Solar energy can make space travel more fuel-efficient.
- Tatyana Woodall archive page
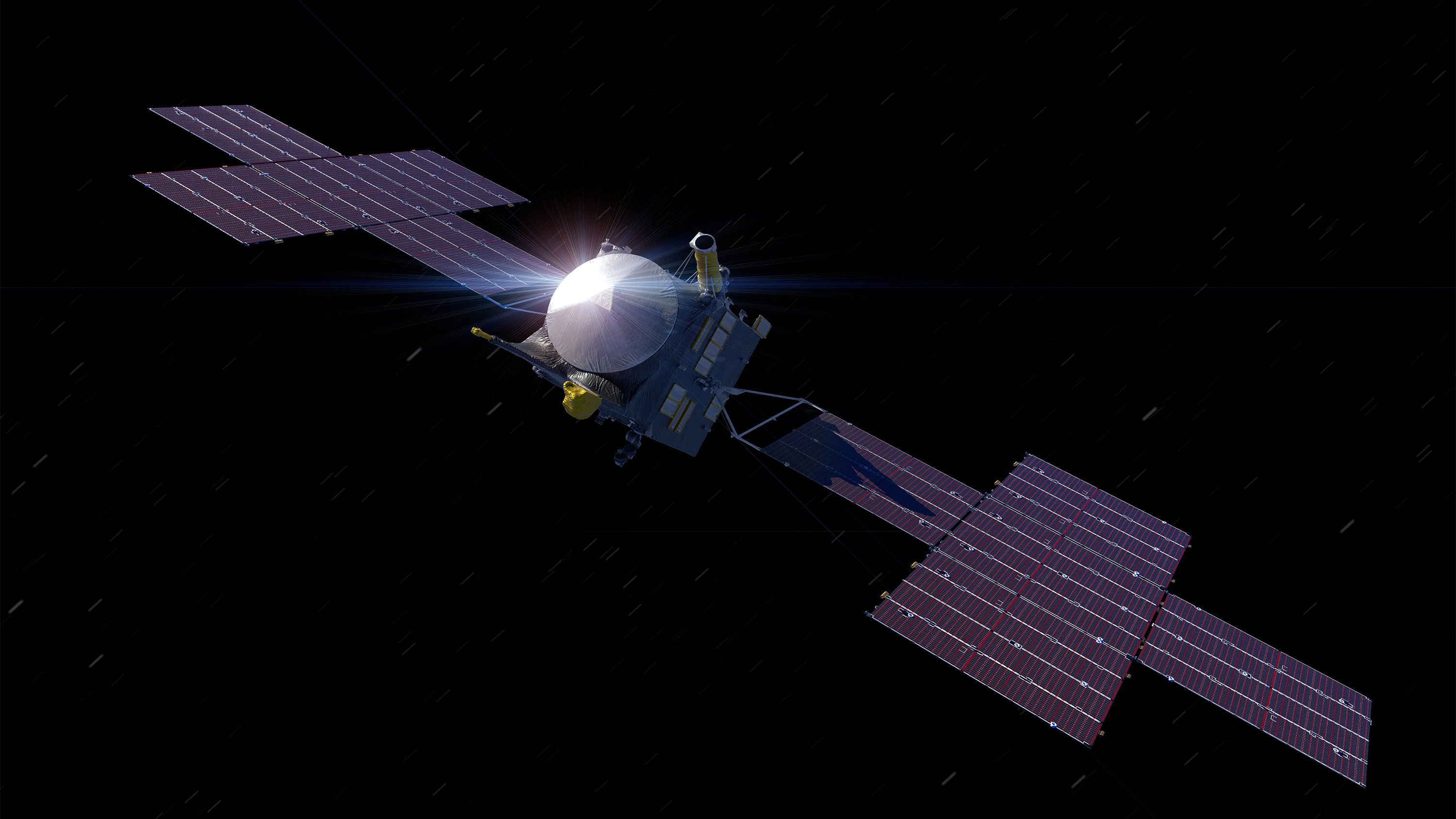
In August 2022, a NASA probe called Psyche will set out to explore a giant metallic asteroid called Psyche 16, to help scientists learn more about how planets form. The way Psyche reaches its target, though, will be different from typical NASA missions.
Building on technology used in previous missions, including Dawn and Deep Space 1 , solar power will help propel Psyche into deep space. If that proves successful, it could be the start of a new era of using more fuel-efficient probes for both space exploration and commercial missions.
Traditional spacecraft rely on chemical reactions between a combination of liquid fuels to get around rather than electricity. Psyche will use two giant solar arrays to convert solar energy into electricity that will power four ion thrusters. That electricity will turn tanks of xenon gas (the same kind used in car headlights) into xenon ions, which Psyche’s four thrusters will eject to gently propel the spacecraft toward the asteroid, which orbits between Mars and Jupiter, more than 1.5 billion miles from Earth.
While other spacecraft, like Lucy , have used solar energy to operate instruments, Psyche will be among the first of NASA’s deep-space missions to use solar energy for both onboard operations and propulsion.
Paulo Lozano , director of MIT’s space propulsion laboratory, says Psyche could lay the groundwork for more solar-powered space exploration. Eventually, the technology could help us investigate multiple celestial objects for longer periods and potentially make human-crewed missions outside of Earth’s orbit more affordable and feasible.
“It actually opens up the possibility to explore and to commercialize space in a way that we haven’t seen before,” Lozano says.
Because a spacecraft that uses solar-electric propulsion requires less propellant than a chemically powered one, it has more space on board for cargo, scientific instruments, and, someday, astronauts. One company, Accion Systems , is developing more efficient ion thrusters for Cubesats as well as larger satellites and other spacecraft.
Solar propulsion technology is already common in satellites that orbit Earth, but until now it has not been a powerful enough alternative to chemically powered engines to be used as often in spacecraft headed to deep space. Advances in solar electric propulsion will change that.
The technology behind Psyche had its first major test in Dawn, an exploration spacecraft that used solar power and ion thrusters. Dawn eventually went silent while orbiting the dwarf planet Ceres (where it will remain in orbit for decades) in 2018, three years after the mission was supposed to end. These thrusters can operate for years without running out of fuel, but they provide relatively low thrust compared with conventional propulsion.
Psyche’s thrusters will be able to generate three times as much thrust as its predecessors, and about a year after launch, it will get some help from Mars’s gravitational pull to change its trajectory before eventually reaching its target in 2026.
After that, Psyche will spend just under two years orbiting the asteroid. Its mission will be to examine the asteroid’s iron core to determine whether it has the same elements that have been discovered in Earth’s high-pressure core, which can help researchers learn more about how planets form.
Although we can’t directly view Earth’s core, Psyche will use a multispectral imager, an instrument that uses filters and two cameras to obtain high-resolution geologic, compositional, and topographical data from the asteroid. If its core proves similar to those of small, rocky planets, scientists could determine whether the two have similar origins. Because Psyche 16 (the asteroid) is thought to be the core of a planet that failed to form, an up-close look could provide details about the formation of the inner solar system.
Back in 2017, Psyche was chosen as one of two missions for NASA’s Discovery Program, a series of low-cost missions to targets around the solar system. Led in part by Arizona State University, Psyche had about a $450 million development cap to ensure it could make it all the way to deep space. But the farther a spacecraft goes from the sun, the more difficult it becomes for its solar arrays to capture sunlight and power its ion thrusters. That’s why once Psyche is past Mars, it will have to slow down.
Keep Reading
Most popular.

What is AI?
Everyone thinks they know but no one can agree. And that’s a problem.
- Will Douglas Heaven archive page

How to fix a Windows PC affected by the global outage
There is a known workaround for the blue screen CrowdStrike error that many Windows computers are currently experiencing. Here’s how to do it.
- Rhiannon Williams archive page

A controversial Chinese CRISPR scientist is still hopeful about embryo gene editing. Here’s why.
He Jiankui, who went to prison for three years for making the world’s first gene-edited babies, talked to MIT Technology Review about his new research plans.
- Zeyi Yang archive page

Google DeepMind’s new AI systems can now solve complex math problems
AlphaProof and AlphaGeometry 2 are steps toward building systems that can reason, which could unlock exciting new capabilities.
Stay connected
Get the latest updates from mit technology review.
Discover special offers, top stories, upcoming events, and more.
Thank you for submitting your email!
It looks like something went wrong.
We’re having trouble saving your preferences. Try refreshing this page and updating them one more time. If you continue to get this message, reach out to us at [email protected] with a list of newsletters you’d like to receive.

Energy Resources for Space Missions
By Göktuğ Karacalıoğlu on January 16, 2014 in Nuclear Propulsion
Today, energy is possibly one of the most critical sources for humanity. On Earth, we make use of different energy resources such as fossil fuels (coal, oil, etc.), nuclear power, and renewable energy (solar, wind, geothermal, etc.). Similarly, space missions require power at different stages of their life cycle, and they require their own power sources.
For the satellites orbiting Earth, the state-of-the-art technology is solar power, where electricity is generated by the photovoltaic effect of sunlight on certain substrates, notably silicon and germanium. Missions to study Sun and the inner-solar system planets like Soho, Mercury Messenger, and Venus Express, also make use of solar power, employing solar panels to generate the necessary electric power to keep them running.
However, because the intensity of sunlight decreases with the square of the distance from the Sun, solar power becomes too weak beyond a certain distance and the spacecraft that are sent off to the outer solar system and beyond need a different source of energy to power up their systems. (The Juno spacecraft, launched in 2011, will be the first mission to observe Jupiter using solar power; however it will need to employ three huge solar panels of 2.7 m x 8.9 m size to meet its power requirements.) Also for landers and rovers that must operate on dusty surfaces or on the dark side of planets in long-duration shadows, solar power is not an option.
Illustration of a Radioisotope Thermoelectric Generator (Credits: US Department of Energy).
For such missions, nuclear power systems have been used for more than five decades, enabling us to construct a knowledge base about distant planets and interstellar space. The two basic types of nuclear power supply used in space are “nuclear reactors” and “radioisotope sources.” In a nuclear reactor system, the energy source is the heat generated by the controlled fission of uranium. This heat is then transferred by a heat-exchange coolant to either a static or dynamic conversion system, which transforms it into electricity. In a radioisotope system, unstable chemical isotopes (radioisotopes) are used as the heat source; but unlike nuclear reactors, no fission or fusion processes are used. Rather, this technology relies on thermoelectric materials—special kinds of semiconductors that generate an electric current when one end is kept hotter than the other end. The greater the temperature difference, the more electricity is produced. These systems, generally referred to as Radioisotope Thermoelectric Generators (RTGs), are known to be simple and very reliable since they do not involve any moving parts. They are also long-lasting power sources since they operate as long as the isotope produces a useful level of heat.
The US only tested one nuclear reactor in space to date, and mostly used RTGs in its missions. Russia, in contrast, has launched about 40 spacecraft with nuclear plants aboard, as well as some with RTGs. However, if we exclude Earth orbiting spacecraft, it would be correct state that RTGs has been the dominant nuclear power system for space exploration missions.
The first nuclear-powered spacecraft, the navigational satellite Transit 4A, was launched more than 50 years ago, in 1961. Since then, dozens of spacecraft have been launched carrying RTGs and have explored the Moon and almost every planet in the solar system. In many of these cases, the radioisotope was not only used for power generation, but also to keep the spacecraft warm in frigid environments on planetary surfaces or in deep space, which is quite critical for efficient operations.
The figure below from Space.com shows the US space probes that have carried RTGs. It is worth mentioning that, after 37 years of operation, Voyager-1 is now officially the first man-made object to enter interstellar space, and its RTGs are expected to keep on working until 2025!
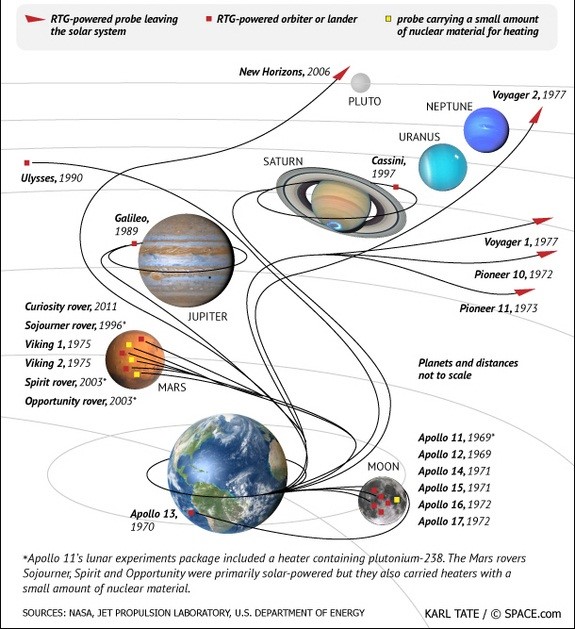
US Space Missions with RTGs (Credits: Space.com).
What Fuels an RTG?
Plutonium-238, with a half-life of 88 years, is the most widely used radioisotope in RTGs. With its high heat capacity, it allows the heat sources to be compact while resulting in useful levels of energy after the heat to electric conversion process. One kilogram of Pu-238 can generate approximately 560 watts of thermal power.
Another advantage of Pu-238 is its emission characteristics. In contrast to several other radioisotopes that release nasty gamma or beta radiation, Pu-238 decays by an alpha emission process and has exceptionally low gamma and neutron radiation levels. Since alpha particles can be stopped by material as thin as a sheet of paper, using Pu-238 eliminates any heavy shielding requirements to protect both humans and the spacecraft from the radioactive effects of the isotope.
As a summary, Pu-238 is a special material with several unique features which have made it the preferred choice to power space missions for decades. However, there is a problem: we don’t have any more of it!
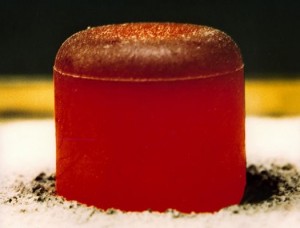
An Pu-238 pellet (Credits: Los Alamos Natıonal Laboratory).
Of course, this situation did not pop out suddenly. Naturally, plutonium can be found in extremely small traces, but Pu-238 was discovered in 1940s by researchers. It was than produced in several laboratories around the world for several decades. However, The United States terminated Pu-238 production in 1988 with the end of the Cold War and augmented the demand by purchasing stock from Russia. However, Russia reneged on a contract to supply the US in 2009, and according to a Wired author Dave Mosher: “it’s not just the U.S. reserves that are in jeopardy. The entire planet’s stores are nearly depleted. The nuclear crisis is so bad that affected researchers know it simply as “The Problem.” Mosher estimates that NASA is left with 15-16 kilograms of Pu-238 in stocks, whose energy potential continuously depletes by around a percentage point each year. Considering that each of the previous RTG-powered space missions used 3 to 11 kg of Pu-238 aboard, this stock will only be enough to launch 2-3 small deep-space missions within the current decade.
Pu-238 Alternative?
Considering that Pu-238 is so scarce, are there not other radioisotopes that can replace or back it up? Actually, the European Space Agency (ESA) is considering alternative nuclear fuels to power its upcoming probes traveling into the deep space. “To see ourselves as a serious planetary science partner on the world stage with the United States, we’re building up our nuclear capability for European-built RTGs,” David Southwood, ESA’s director of science and robotic exploration, said in an interview with Spaceflight Now .
In order to be a decent RTG power source, an isotope needs to have:
1. Good power density, since the current energy conversion efficiencies are quite low
2. Good half-life, since it is aimed to operate the spacecraft as long as possible
3. Require little to no shielding, in order not to interfere with sensitive instruments aboard and also to protect humans.
4. High power/mass ratio, to minimize the propulsion requirements to launch it into space
Pu-238 is the ideal fuel because it is shows good performance in all four categories. Other possible solutions such as Strontium-90, Polonium-210 (which was used in older Russian RTGs), and Curium-242/244 lack at least one of the above properties. The only reasonable alternative seems to be Americium-241, which has a longer half-life (over 150 years), but around ¼ power density compared to Pu-238. Americium-241 exists rather in large quantities, since it is a by-product of regular power reactors. So, if more energy-efficient RTG designs offset its low power density, it might be a feasible alternative as a spacecraft fuel in the future. However, being a more aggressive neutron emitter than Pu-238, Americium is a greater radiation hazard for the scientists and engineers who handle it.
Currently, worldwide production of Am-241 is only a few kilograms per year, most of which is already used to fill demand for smoke detectors and moisture gauges. According to flightglobal.com , BNSC space science director David Parker says that Harwell (ESA’s new facility in the UK) will study Americium as an alternative to Pu-238, and Oxford University is researching infrared-related technology as an alternative conversion solution.
Researchers have also discussed the potential use of a rarer Americium isotope, Am242m, in space applications. This isotope can be created when Americium-241 absorbs a neutron and generates a very efficient fission reaction. However more studies have to be conducted before any real application can be attempted.
More Efficient RTG Designs
Current RTG systems in use, called the Multi-Mission Radioisotope Thermoelectric Generators (MMRTG), convert only around 8 percent of the heat energy released to electricity. Therefore, an increase of the conversion efficiency of RTGs is a current engineering requirement and challenge, whether they are operated with Pu-238, or any other alternative fuel. A new design concept called the Advanced Stirling Radioisotope Generator (ASRG) was studied over a decade at NASA, using a Stirling thermodynamic cycle to extract electricity out of Pu-238.
The Stirling engine works by heating and changing the pressure of the gas inside, causing a piston movement. However, although this concept generates almost four times more electricity for the same amount of plutonium compared to the current RTGs, it might set a limit to the useful life of the spacecraft since it involves moving parts which could affect the sensitive instruments aboard due to continuous vibration.
The Safety Aspect
Just as it has always been a point of discussion for terrestrial nuclear systems, the “safety aspect” of nuclear power in space should always be kept in mind and questioned as well. Compared to alternative isotopes, safety officials say Pu-238 is the safest fuel for nuclear-powered spacecraft. Within the available set of fuels, this statement might be correct for the nominal operation conditions in a mission, but what if the mission fails?
To minimize the risk of the radioactive material being released, Pu-238 is stored in individual units with their own heat shielding in RTGs. They are surrounded by a layer of iridium and encased in high-strength graphite blocks. These two materials are corrosion- and heat-resistant. An aeroshell surrounds the graphite blocks and protects the entire assembly against the heat of reentering the Earth’s atmosphere.
According to NASA reports , for use as fuel in an RTG, Pu-238 is processed with other materials into a ceramic form called plutonium dioxide. Since emissions from plutonium do not penetrate the skin, the primary hazard from Pu-238 dioxide occurs if it is somehow pulverized into very tiny particles that are inhaled, after which they can irradiate internal organs. Particularly at risk is the skeleton, the surface of which is likely to absorb the isotope, and the liver, where the isotope will collect and become concentrated. However, in the unlikely event of a launch or reentry accident that released the fuel, the ceramic form is expected to break primarily into large, non-inhalable pieces rather than fine particles that could be harmful to human health or the environment. This ceramic form also has a high melting temperature and a very low solubility in water, so these features greatly limit the movement of any material that might be released in the environment, further reducing the chance that anyone could be exposed to it, at least unwittingly.
As explained earlier, nuclear power systems can be used both for the Earth-orbiting satellites and for more distant planetary missions. However, both of these missions start with a launch phase, where most of the failures might be expected to occur. A good deal of effort has been invested to make today’s RTGs more or less impervious to the most likely launch accident scenarios, but NASA’s environmental impact statement still concludes that there is a 1-in-350 chance of a launch mishap in which plutonium could be released into the environment. Once the launch is successful, the rule of thumb for the satellites in low Earth orbit carrying nuclear reactor cores is to eject these cores into a higher disposal orbit at the end of the mission, where they stay a few hundred years and loose much of their nuclear power. But there were several failure incidents, some of which resulted in radioactive material reentering the Earth’s atmosphere, and even falling landside with possible human population exposure.
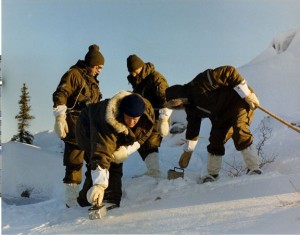
Department of Energy Nuclear Emergency Support Team members sweep for radiation following the crash of Cosmos 954 (Credits: Department of Energy).
Taking a step back and looking into history can provide a better understanding of the problem. The first nuclear accident in space is reported as the USA’s Transit 5BN-3 satellite mission, which failed to achieve orbit in 1964, releasing its Pu-238 into the Earth’s atmosphere at an altitude of 50 km. In 1968, US’s Nimbus B-1 weather satellite exploded when the launch vehicle had to be intentionally destroyed shortly after the lift-off. The RTG was recovered from the bottom of the Santa Barbara Channel in the Pacific Ocean off California five months later, so no radioactive material had been released. In 1969, the first Lunokhod rovers of USSR were launched from Baikonur with another isotope, polonium-210, on board. However, the rocket exploded and radioactivity was spread over a large area of Russia. A failure of another USSR launch vehicle caused a nuclear piece to fall into the Pacific Ocean, north of Japan, in 1973. Five years later, USSR’s Cosmos 954 satellite accidentally reentered Earth’s atmosphere, scattering around 50 kg of radioactive uranium-235 over northern Canada. Although this area was sparsely populated, several residents were accidentally exposed to radiation -none resulting in serious harm- before a major recovery campaign succeeded in sweeping a total area of 124,000 square kilometers over the course of almost one year. (This accident is still the first and only instance where the 1972 UN Liability Convention was invoked between two signatories.) Another failure was Cosmos 1402 of USSR, in 1983. At the end of that satellite’s operational period, the reactor core did not separate to be sent into higher orbit as planned, but reentered the atmosphere with the remainder of the spacecraft, landing somewhere in the South Atlantic Ocean.
One other important accident involving RTGs was the heroic Apollo 13 mission, which is known as the “successful failure” in space history. This mission fortunately ended up with return of the astronauts safely to Earth, however not only they but also their RTG, which was intended to be left behind on the Moon’s surface, made it back to Earth. The RTG and its 3.9 kilograms of plutonium dioxide plunged into the Tonga Trench in the Pacific Ocean, where it will remain radioactive for the next 2,000 years. Subsequent water testing has shown the RTG is not leaking radioactivity into the ocean.
A more recent failure was Mars 96, a Russian Mars mission, which failed to escape the Earth and broke up over Chile after its reentry in 1996. Although it was stated that the spacecraft had splashed down into the ocean initially, further analysis showed that it is reasonable that the impact was in fact on land. The spacecraft, which was equipped with 200 grams of Pu-238, was never recovered since the exact location(s) of the impact could not be determined.
The list above is definitely not complete, but it gives an idea of the results of possible accidental failures in nuclear-powered space missions. However, this is not the only danger. As mentioned before, the nuclear cores of RTGs are ejected to a higher orbit at the end of lifetime of a reentering satellite. This means that there are kilograms of nuclear waste spinning around the Earth at present. Although unlikely, any collision of these particles with other particles of space debris could possibly shift them to lower orbits resulting in their uncontrolled reentry.
Considering previous failures, scientific characteristics, and current design improvements, we may conclude that Pu-238 powered RTGs are safe systems unless there has been direct exposure to a person, locally. This is an unlikely situation, although NBC News Space Consultant James Oberg ’s account of one incident shows that it is not impossible: “After the explosion of a rocket near the Baikonur cosmodrome in 1970, Soviet soldiers found a nuclear battery in the wreckage. Later, investigators looking for the battery discovered that the shivering soldiers had secretly kept it as a hand-warmer in their poorly heated guardhouse.”
Therefore, while continuing to use the radioisotopes, as well as RTGs systems, it should always be kept in mind to consider all the possible failure modes, and take precautions accordingly for each of them.
Current Situation
During 2013, the space exploration community received both some good news as well as bad from NASA. The good news is that, after long years of debates to find the budget to reinitiate Pu-238 production, the US has restarted this radioisotope’s production in its High Flux Isotope Reactor at Oak Ridge. However the production is still at a lab scale and for very small quantities. NASA estimates a necessity of 2 kg/year of Pu-238 for its robotic planetary missions alone, which might be satisfied once production reaches its estimated full scale rate in 2018 or 2019. However, any manned mission in the future will definitely need much greater amounts of the isotope.
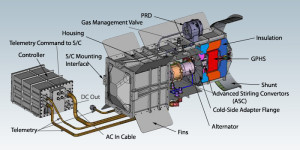
Diagram of NASA’s Advanced Stirling Radioisotope Generator, which has now been shelved (Credits: NASA/DoE).
In terms of new RTG designs, Lockheed Martin Space Systems was working on ASRGs for NASA, and it was expected that two flight-ready ASRGs will be delivered in 2016. However, before the end of 2013, NASA’s Planetary Science Division Director Jim Green announced that the ASRG development program was cancelled due to budget constraints: “With an adequate supply of Plutonium-238, and considering the current budget-constrained environment, NASA has decided to discontinue procurement of ASRG flight hardware. The hardware procured under this activity will be transferred to the Glenn Research Center to continue development and testing of the Stirling technology.”
Future Missions
According to the current plans, the next mission to use RTGs will be a copy of the Mars Curiosity rover. This mission is estimated to be launched in 2020, and is expected to include around 4 kg of Pu-238. Another mission planned in the 2020s is a flagship probe to study Jupiter’s moon Europa. Considering these plans, it is promising to know that the US has taken action to start Pu-238 production, although it is doubtful that the production rates will satisfy the scheduled demand. Europe is also known to be conducting studies on alternative radioisotopes and estimating solid results by the end of this decade, which might act as a back-up stock.
Every space mission contains some level of health and safety risks, as well as budget problems. Positioning ourselves in a completely risk-free zone would cease our exploration capabilities and constrain space science which aims to bring benefits to all humanity. So we should take up the challenge all together to successfully mitigate these risks, and all the space-faring nations should contribute to it both technically and financially.
Since the environmental hazards of nuclear power mostly vanish once a spacecraft escapes from Earth’s gravity, a policy to limit the use of these systems only to space missions travelling beyond Earth orbit would minimize any undesirable results. Engineering studies for designing safer and more efficient nuclear power systems -not necessarily RTGs- should also continue in parallel, in order to raise our readiness level for possible crewed missions to the Moon and Mars.
Since nuclear plants can be used not only as a source of electric power, but also a source of heat and propulsion, they might be our only choice to reach and support life and productive activities at bases beyond planet Earth.
2 Responses
Excellent article! Deserves an Insert in the SSM Quarterly
“One kilogram of Pu-238 can generate approximately 560 watts of thermal power.”… For the first X years after which it decays to Y Watts in so many years later??? Missed some Important detail there. And Watts is a proper noun,and thus, capitalized.
Leave a Reply
Your email address will not be published. Required fields are marked *

Inside Nuclear Propulsion

Browse The Space Shuttle Columbia Disaster

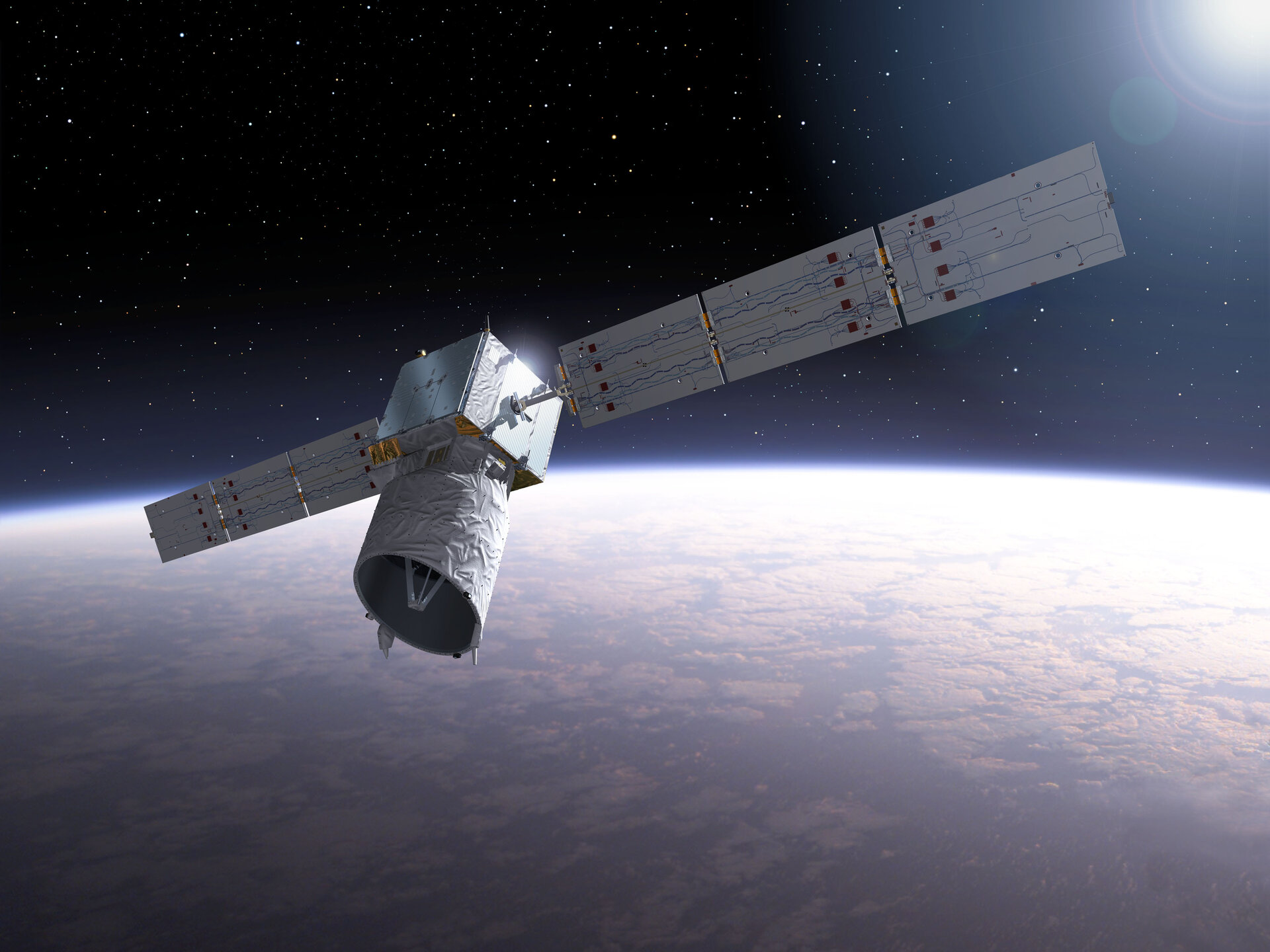
Renewable energy for spaceship Earth
Earth has never been more energy-hungry, but for supply to keep pace with demand, new technology is needed. Technologies for space missions – including power supply and management systems – are helping the terrestrial energy sector as it works to serve its customers, and also to reduce carbon emissions and other environmental impacts.
Wind power is one of the renewable sources of energy which is growing in importance. To maximise the amount of electricity from new wind turbines, the French company Leosphere developed a small instrument to measure wind speed and direction from the ground up to heights of 200 metres. The 'lidar' technology is similar to that on ESA's Aeolus satellite which has been developed to provide global observations of wind profiles from space.
ESA's expertise from the Aeolus mission was important for Leosphere and was used to improve their instrument during the company's start-up phase at ESA's Business Incubation Centre (BIC) in Noordwijk, the Netherlands. Leosphere's 'Windcubes' are used to help select the location and predict the yield of wind farms. Additional uses include climatology and environmental monitoring. More instruments based on the same technology have followed and these are now being used in more than 100 countries.
The output of solar energy plants has also been boosted by help from space. Solar power is generated in two different ways on Earth: solar thermal plants concentrate heat from the Sun using it to create steam to turn turbines, while photovoltaic plants convert sunlight into electricity. In both cases precise, long-term irradiance data is needed for choosing plant locations and estimates of likely energy yield for prospective investors.

By using data from weather satellites, 'SolarSAT' from Italian company Flyby can accurately predict the power output of photovoltaic power plants. This information is used to design improved systems and quickly identify faults in operating photovoltaic plants – faults that can reduce energy production by more than 10% a year. This system has already been installed on several photovoltaic systems in Italy. The company also helps those trying to avoid the damaging effects of the Sun by using satellite data to keep sunbathers safe!
'Sunshine maps', accurate and continent-wide measurements of ground radiances, are provided by the Meteosat Second Generation satellite. Companies like Meteocontrol use this information to identify the optimal locations for photovoltaic power plants. The data can also be used to assess how much power the plant should be generating - so managers can check that their facilities are making the most of the available sunshine.
Connecting the solar power industry with the best information from space was the task of the ENVISOLAR project (Environmental Information Services for Solar Energy Industries), funded by ESA. It streamlined the exploitation of Earth observation data for use in solar energy industries.
Thank you for liking
You have already liked this page, you can only like it once!
Related Links
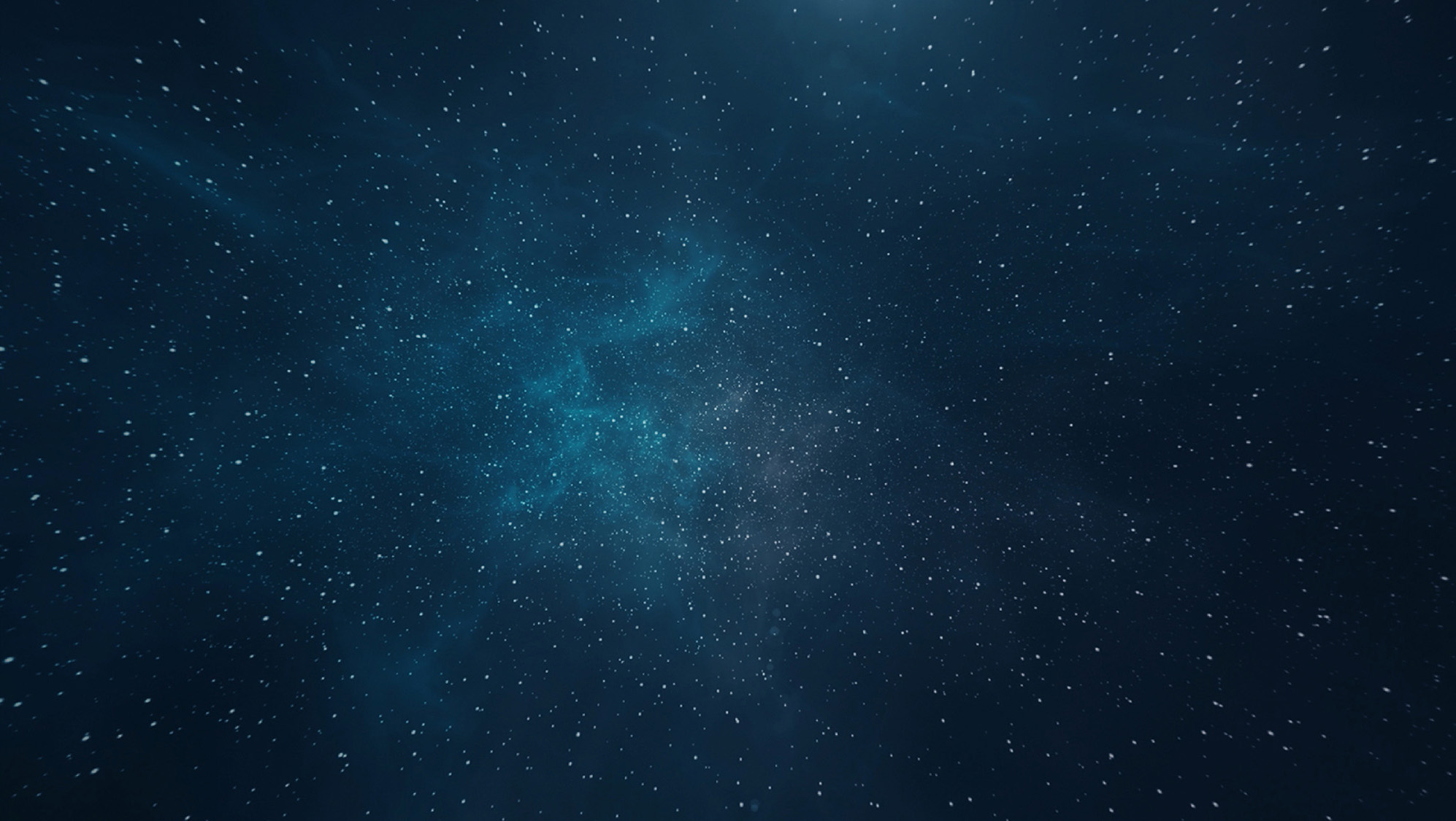
Space technology optimises windmill efficiency
Space technology helps mitigate climate change, sunshine mapping from space means brighter solar energy fut…, renewable energy development, flyby. applied research solutions., meteo control. energy and weather services., envisolar project.
Thank you for visiting nature.com. You are using a browser version with limited support for CSS. To obtain the best experience, we recommend you use a more up to date browser (or turn off compatibility mode in Internet Explorer). In the meantime, to ensure continued support, we are displaying the site without styles and JavaScript.
- View all journals
- Explore content
- About the journal
- Publish with us
- Sign up for alerts
- NEWS EXPLAINER
- 01 February 2023
Could solar panels in space supply Earth with clean energy?
- Elizabeth Gibney
You can also search for this author in PubMed Google Scholar
For 100 years, people have dreamed of sending vast arrays of solar panels into space and beaming their energy down to Earth. Unlike intermittent renewable-energy sources on the ground, these orbiting panels would always bask in bright sunlight and would potentially offer a continuous supply of power.
Access options
Access Nature and 54 other Nature Portfolio journals
Get Nature+, our best-value online-access subscription
24,99 € / 30 days
cancel any time
Subscribe to this journal
Receive 51 print issues and online access
185,98 € per year
only 3,65 € per issue
Rent or buy this article
Prices vary by article type
Prices may be subject to local taxes which are calculated during checkout
doi: https://doi.org/10.1038/d41586-023-00279-8
Reprints and permissions
Related Articles
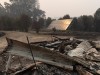
China sets sights on first solar power stations in space
Japan sets sights on solar power from space
- Engineering
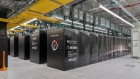
A day in the life of the world’s fastest supercomputer
News Feature 04 SEP 24
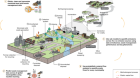
A local-to-global emissions inventory of macroplastic pollution
Article 04 SEP 24
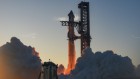
Huge SpaceX rocket explosion shredded the upper atmosphere
News 30 AUG 24
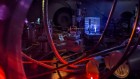
Progress on nuclear clocks shows the benefits of escaping from scientific silos
Editorial 04 SEP 24
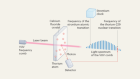
Countdown to a nuclear clock
News & Views 04 SEP 24
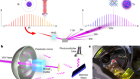
Frequency ratio of the 229mTh nuclear isomeric transition and the 87Sr atomic clock
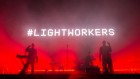
Live music is a major carbon sinner — but it could be a catalyst for change
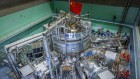
Inside China’s race to lead the world in nuclear fusion
News Feature 28 AUG 24
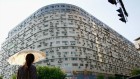
The cool technologies that could protect cities from dangerous heat
News Feature 27 AUG 24
13 PhD Positions at Heidelberg University
GRK2727/1 – InCheck Innate Immune Checkpoints in Cancer and Tissue Damage
Heidelberg, Baden-Württemberg (DE) and Mannheim, Baden-Württemberg (DE)
Medical Faculties Mannheim & Heidelberg and DKFZ, Germany
Postdoctoral Associate- Environmental Epidemiology
Houston, Texas (US)
Baylor College of Medicine (BCM)
Open Faculty Positions at the State Key Laboratory of Brain Cognition & Brain-inspired Intelligence
The laboratory focuses on understanding the mechanisms of brain intelligence and developing the theory and techniques of brain-inspired intelligence.
Shanghai, China
CAS Center for Excellence in Brain Science and Intelligence Technology (CEBSIT)
Research Associate - Good Manufacturing Practices (GMP)
Faculty position in pathology research.
Dallas, Texas (US)
The University of Texas Southwestern Medical Center (UT Southwestern Medical Center)
Sign up for the Nature Briefing newsletter — what matters in science, free to your inbox daily.
Quick links
- Explore articles by subject
- Guide to authors
- Editorial policies
Deep space travel energy sources
Ieee account.
- Change Username/Password
- Update Address
Purchase Details
- Payment Options
- Order History
- View Purchased Documents
Profile Information
- Communications Preferences
- Profession and Education
- Technical Interests
- US & Canada: +1 800 678 4333
- Worldwide: +1 732 981 0060
- Contact & Support
- About IEEE Xplore
- Accessibility
- Terms of Use
- Nondiscrimination Policy
- Privacy & Opting Out of Cookies
A not-for-profit organization, IEEE is the world's largest technical professional organization dedicated to advancing technology for the benefit of humanity. © Copyright 2024 IEEE - All rights reserved. Use of this web site signifies your agreement to the terms and conditions.
Power Sources for Space Exploration
Ben johnson december 11, 2012, submitted as coursework for ph240 , stanford university, fall 2012, introduction.
Spaceflight presents unusual challenges to storing and collecting electrical power. Electrical power is required to operate instruments and support equipment. In addition to the modest power requirements of computing, communications, and sensing electronics, some spacecraft components require large amounts of electrical power. Large peak power levels allow for radars to achieve better range or penetration through materials. It can also be used for propulsion in the form of ion drives, which improve efficiency over regular rockets by separating the storage of energy from the storage of reaction mass.
Most satellites in Earth orbit are powered by solar arrays. These are large, but they can be folded up at launch and deployed on orbit. Photovoltaic technology has seen rapid development in recent years. For operation beyond Earth, the reduced intensity of light from the sun means that spacecraft must have larger solar arrays, which can introduce several disadvantages. The mass of solar panels is mass that cannot be used for payloads, and the increased vehicle mass requires more powerful maneuvering systems (jets and reaction wheels). Large solar arrays may be viable for operation in the outer solar system, but with significant disadvantages compared to RTGs (see below). [1] Solar panels can be made with safe, readily available materials, while RTGs require fuels that are difficult to safely handle and which are in limited and highly regulated supply. The Mars Exploration Rovers (Spirit and Opportunity) obtained all their electrical power from solar panels. There was concern early in the mission that the lifetime of the rovers would be limited by dust accumulating on the rovers, but the dust was periodically removed by wind, leaving mechanical failure as the only limiting factor in the rovers' lifetimes. [2]
Probes that travel a long distance from the sun often use radioisotope thermoelectric generators (RTGs). These devices contain pellets of a radioactive material, often Plutonium, which produces heat from radioactive decay. Thermocouples convert some of this heat into electric power. The radiation from the fuel is not used by RTGs, but there are designs for devices which can produce electricity by capturing beta particles. [3]
The principle advantage of an RTG over a solar array is that the RTG works in any environment for a very long time. The Voyager probes, currently the most distant artificial objects, are both powered by RTGs and are still operating, albeit with the loss of some instruments. [4] RTGs have no moving parts and are very simple: fundamentally, they consist of a fuel cell, thermocouples, and shielding. The output of an RTG decreases over time due to decay of the heat source and degradation of the semiconductor thermocouples from radiation.
In contrast to the earlier Mars Exploration Rovers, the Mars Curiosity rover usese an RTG to generate its electrical power. It also has a lithium-ion battery pack which is recharged by the RTG to support large transient loads. The rover can operate at night, or it can use large amounts of power during the day and recharge the battery at night.
RTGs are very inefficient: Curiosity's RTG produces 2000W of thermal power but only about 120W of electrical power. The extra heat is dissipated by fins. This large amount of waste heat adds engineering challenges that other power sources don't face: even when electrical power from the RTG is not being used, it still produces full thermal power which must be dumped into the environment. During transport and assembly on Earth, cooling systems must be provided to keep the RTG at an acceptable temperature.
The presence of radioactive material in an RTG means that safety is a major design consideration. Modern RTGs are designed to survive a launch failure or re-entry without dispersing their fuel. There is little radiation risk from an intact RTG. In fact, a few manned missions have included one. Apollo missions 12-17 carried an RTG as part of an experiment to be left on the moon, and one fell to earth when Apollo 13 returned with its lunar module descent stage. This RTG fell into a deep trench in the Pacific ocean and is currently believed to be intact. [5]
Apollo and the space shuttle both used hydrogen-oxygen fuel cells for electrical power. Fuel cells combine two chemical components at a controlled rate to produce heat, electricity, and some chemical waste product. In the case of hydrogen-oxygen fuel cells, this waste produce is water, which could be used by the crew.
Fuel cells require significant support equipment which adds significant weight and introduces potential failure modes. The two fuels must be stored in tanks, cryogenically for hydrogen and oxygen. The fuels are delivered to the fuel cells by plumbing and valves which could fail mechanically. The famous failure of Apollo 13 was caused by an explosion of an oxygen tank supplying fuel cells and breathable air to the crew. [6] This single point of failure severely reduced electrical power to the spacecraft and endangered the crew due to a limited supply of breathable oxygen.
Fuel cells share the advantage with RTGs of operating without the need for sunlight, but the fuel is consumed quickly. While an RTG may operate continuously for decades, a fuel cell may deplete its fuel supply in days under normal operation. Unlike RTGs, fuel cells use safe materials and their reaction rate can be controlled to limit waste heat. The poor energy-to-mass ratio of fuel cell systems may limit their applicability to near-Earth manned spacecraft, where a supply of oxygen is already needed and the waste water can be put to use.
Non-rechargeable batteries are not viable on most spacecraft as a primary power source, although some small amateur satellites have used primary cells for short missions. They have been used on some spacecraft for special purposes: Curiosity's descent stage used thermal batteries, and the Apollo missions used batteries to power re-entry systems after the service module containing the fuel cells was jettisoned.
Rechargeable batteries are important for load levelling. They are commonly used on satellites to provide continuous power while the satellite moves in and out of sunlight. Curiosity uses a rechargeable lithium-ion battery pack to power large temporary loads while the rover's RTG provides a lower, constant amount of power.
Research into more efficent solar panels is ongoing, primarily for providing power on Earth. The future of RTGs is in question due to the limited supply of fuel, but NASA is investigating producing small quantities of Pu-238 for space missions. Radioisotope-powered Stirling engines are a possible improvement to current RTGs. Like RTGs, such an engine uses a block of radioactive material as a heat source, but instead of thermocouples it uses a heat engine to operate a generator. It promises to be more efficient than an RTG, but with increased complexity. A prototype has been built, but none have been launched. [7]
© Ben Johnson. The author grants permission to copy, distribute and display this work in unaltered form, with attribution to the author, for noncommercial purposes only. All other rights, including commercial rights, are reserved to the author.
[1] M. F. Piszczor et al. , " Advanced Solar Cell and Array Technology For NASA Deep Space Missions ," Prof. 33rd IEEE Photovoltaic Specialists Conference, (PVSC '08), 11 May 08.
[2] P. M. Stella et al. , " Managing PV Power on Mars - MER Rovers ," Proc. 34th IEEE Photovoltaic Specialists Conference (PVSC '09), 7 Jun 09, p. 001073.
[3] R. Bao, P. J. Brand and D. B. Chrisey, "Performance of Radiation-Hardened High-Efficiency Si Space Solar Cells," IEEE Trans. Electron Devices 59 , 1286 (2012).
[4] R. P. Rudd, J. C. Hall, G. L. Spradlin, "The Voyager Interstellar Mission", Acta Astronautica 40 , 383 (1997).
[5] G. R. Schmidt, R. D. Abelson and R. L. Wiley, "Benefit of Small Radioisotope Power Systems for NASA Exploration Missions", AIP Conf. Proc. 746 , 295 (2005).
[6] " Report of the Apollo 13 Review Board ," U.S. National Aeronautics and Space Administration, June 1970.
[7] J. Chan, J. G. Wood and J. G. Schreiber, "Development of Advanced Stirling Radioisotope Generator for Space Exploration," U.S. National Aeronautics and Space Administration, NASA/TM-2007-214806 , May 2007.
[8] N. Nguyen, " Space Propulsion Technology and Energy Expenditures ", Physics 240, Stanford University, Fall 2011.
[9] S. Kumar, " Energy From Radioactivity ", Physics 240, Stanford University, Fall 2011.
SIGNALS FROM THE EDGE
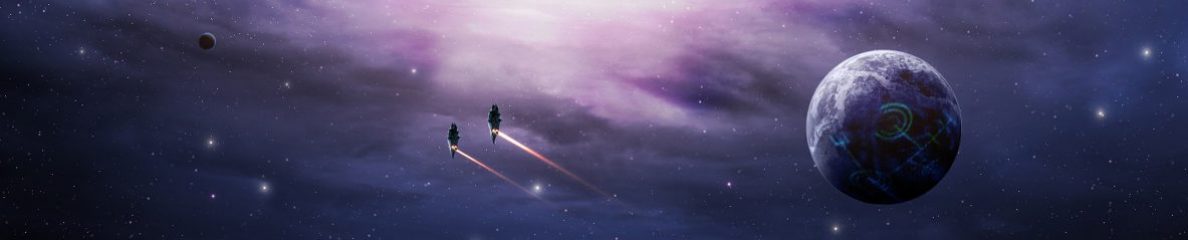
Can We Use Renewable Energy for Space Travel?

The Space Race of olden days is over, but the spirit lives on today with the growing desire to conquer Mars. Privatized space programs go toe-to-toe with NASA and CNSA while the mass public looks on and questions the future.
One of the questions that has always burned in me is “how will we power spacecraft in the future when we’re all out of fossil fuels? What happens if Earth can no longer sustain us, and we have to find alternative energy sources?”
With each passing day, SpaceX and Blue Origin work toward touching the stars, while things down here on Earth are approaching an important crossroads.
As the conversation around renewable energy grows louder in the face of rising fuel prices and economic inflation rates many of us have never seen, another, less urgent question rises up. We might be able to use renewable energy to power our vehicles, but can we use renewable energy sources for space travel?
Renewable Energy That’s Plausible For Space Travel
Right now, you might be running through the short list of all the renewable energy sources and weighing which ones could possibly work in space. Well, there are a slim few.
Wind power is obviously a bust, because, no wind. Geothermal is also a no go for obvious reasons, and hydroelectric power falls in the same boat.
So that leaves us with a few options. Solar power is perhaps the most viable option for powering space travel. It’s already being used in various space capacities, from powering satellites to beaming down solar power to Earth , as sci-fi as that sounds.
But one of the main problems with using solar power is that it doesn’t provide enough propulsion to exit Earth’s atmosphere. With jet fuel, combustion provides an immense amount of energy all at once, which is how we can send rockets into space and keep them traveling even after they exit our atmosphere.
With solar power, you need a conduit to harness the power. We might be able to turn solar energy into electricity, but without the right kind of motor or funnel, we won’t be able to use that electricity for anything useful.
However, when solar energy is thought of as a proponent of chemical propulsion methods, engineers can cut down on overall fuel costs associated with sending spacecraft into orbit.
Using Solar Power for Farther Travel
NASA has a program dedicated to research and development of solar-powered technologies for use in spacecraft propulsion. SEP, or Solar Electric Propulsion, is designed to “extend the length and capabilities of ambitious new exploration and science missions.”
The way SEP works is fairly straightforward.
- Onboard solar arrays collect solar rays and convert it into electricity, to be used for a number of spacecraft systems. Arrays are either in a fan or a window-shade formation.
- Energy from the solar arrays is used to power electrostatic Hall thrusters.
- Electrons trapped in the magnetic field created by the thrusters then ionize inert xenon gas to create a plasma propellant, which moves the spacecraft forward at a constant speed.
This technology has been in the works for a long time, but it’s finally starting to come to the forefront of space innovation. Researchers theorize that eventually, SEP will be one of the primary methods for space propulsion.
There’s still the question of leaving Earth’s atmosphere, and whether solar power can generate enough electricity to launch a craft into space. But, SEP makes it possible to travel longer distances in space without the need for heavy, onboard fuel sources like conventional rockets.
Futuristic Energy Sources for Space Travel
In science fiction, one of the most common themes is using some kind of nuclear power for space travel. It appears in Star Trek, The Expanse , and plenty of other popular sci-fi novels, shows, and games.
Nuclear fusion tends to be the technology that’s most used, and it’s different than fission, which is the process used to split atoms (like for the atomic bomb).
Great strides are being made toward making fusion energy a viable energy source , but because of the size constraints, it’s unlikely that we’ll see them used in spacecraft any time soon.
Whenever discussing the logistics of space propulsion, I like to go back to Warhammer 40k. The orks in 40k have such large numbers that their collective willpower is enough to psychically force junky spaceships to run flawlessly. If only our own space travel was as simple as that! Willpower is perhaps the most renewable energy source out there!
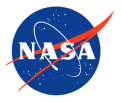
Suggested Searches
- Climate Change
- Expedition 64
- Mars perseverance
- SpaceX Crew-2
- International Space Station
- View All Topics A-Z
Humans in Space
Earth & climate, the solar system, the universe, aeronautics, learning resources, news & events.
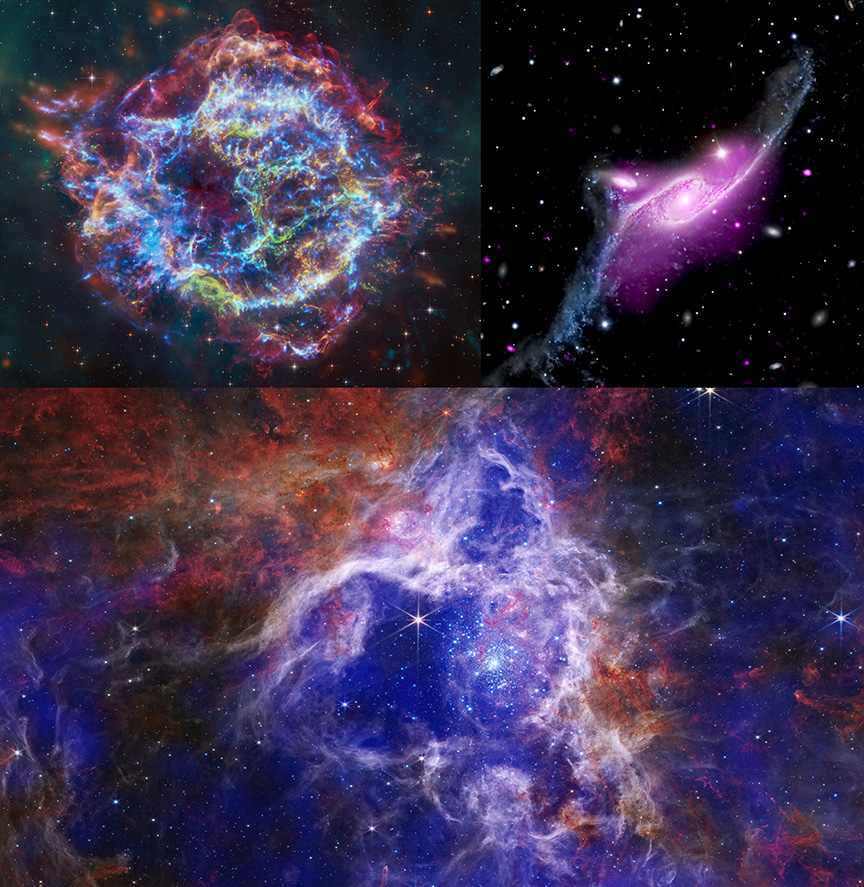
New NASA Sonifications Listen to the Universe’s Past

What’s Up: September 2024 Skywatching Tips from NASA

NASA Mission Gets Its First Snapshot of Polar Heat Emissions
- Search All NASA Missions
- A to Z List of Missions
- Upcoming Launches and Landings
- Spaceships and Rockets
- Communicating with Missions
- James Webb Space Telescope
- Hubble Space Telescope
- Why Go to Space
- Commercial Space
- Destinations
- Living in Space
- Explore Earth Science
- Earth, Our Planet
- Earth Science in Action
- Earth Multimedia
- Earth Science Researchers
- Pluto & Dwarf Planets
- Asteroids, Comets & Meteors
- The Kuiper Belt
- The Oort Cloud
- Skywatching
- The Search for Life in the Universe
- Black Holes
- The Big Bang
- Dark Energy & Dark Matter
- Earth Science
- Planetary Science
- Astrophysics & Space Science
- The Sun & Heliophysics
- Biological & Physical Sciences
- Lunar Science
- Citizen Science
- Astromaterials
- Aeronautics Research
- Human Space Travel Research
- Science in the Air
- NASA Aircraft
- Flight Innovation
- Supersonic Flight
- Air Traffic Solutions
- Green Aviation Tech
- Drones & You
Technology Transfer & Spinoffs
- Space Travel Technology
- Technology Living in Space
- Manufacturing and Materials
- Science Instruments
- For Kids and Students
- For Educators
- For Colleges and Universities
- For Professionals
- Science for Everyone
- Requests for Exhibits, Artifacts, or Speakers
- STEM Engagement at NASA
- NASA's Impacts
- Centers and Facilities
- Directorates
- Organizations
- People of NASA
- Internships
- Our History
- Doing Business with NASA
- Get Involved
NASA en Español
- Aeronáutica
- Ciencias Terrestres
- Sistema Solar
- All NASA News
- Video Series on NASA+
- Newsletters
Social Media
- Media Resources
- Upcoming Launches & Landings
- Virtual Guest Program
- Image of the Day
- Sounds and Ringtones
- Interactives
- STEM Multimedia

NASA Invites Social Creators to Experience Launch of Europa Clipper Mission
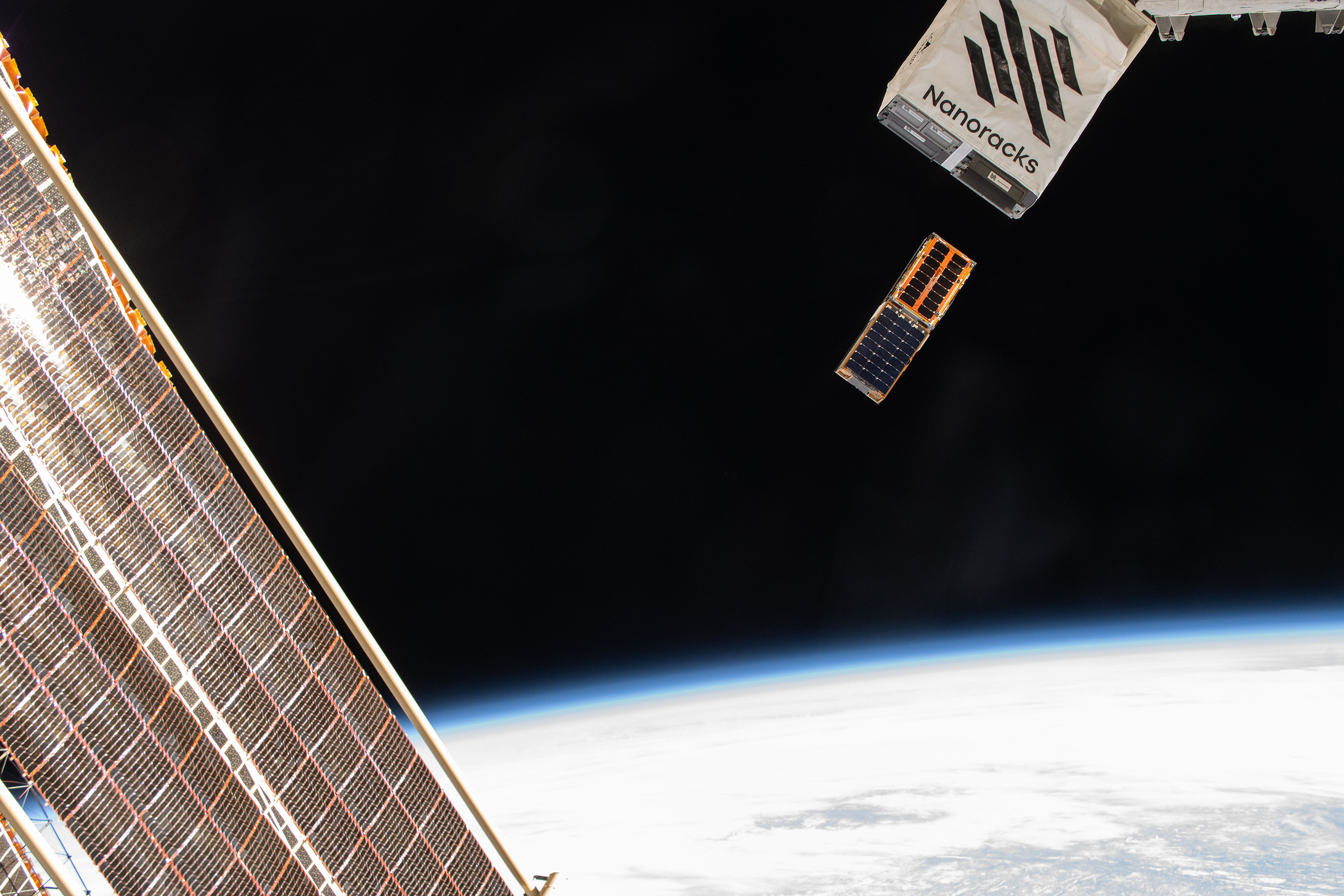
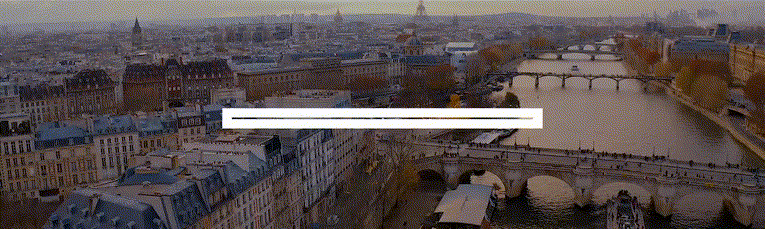
NASA’s Mini BurstCube Mission Detects Mega Blast

NASA Astronaut Don Pettit’s Science of Opportunity on Space Station

NASA, Boeing Optimizing Vehicle Assembly Building High Bay for Future SLS Stage Production

NASA Seeks Input for Astrobee Free-flying Space Robots
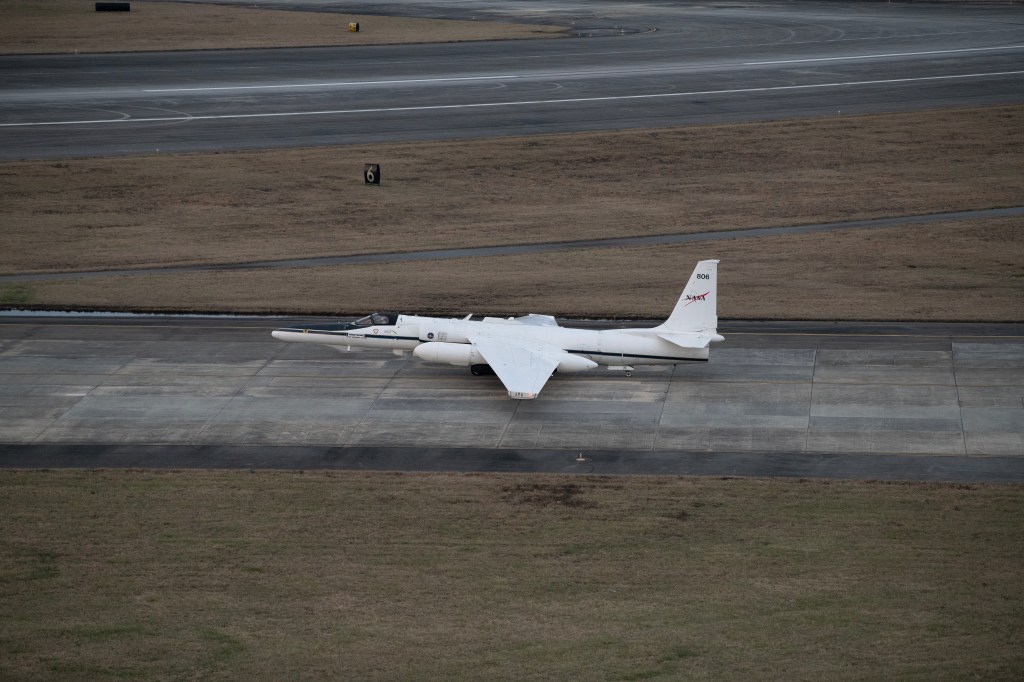
NASA Earth Scientists Take Flight, Set Sail to Verify PACE Satellite Data
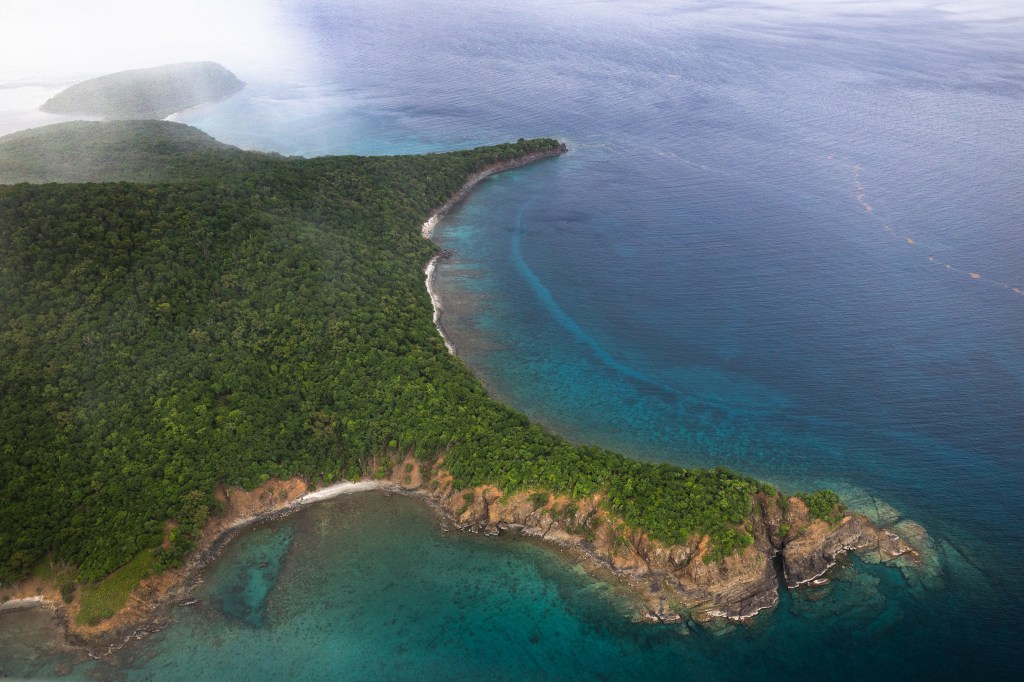
Proyecto de la NASA en Puerto Rico capacita a estudiantes en biología marina
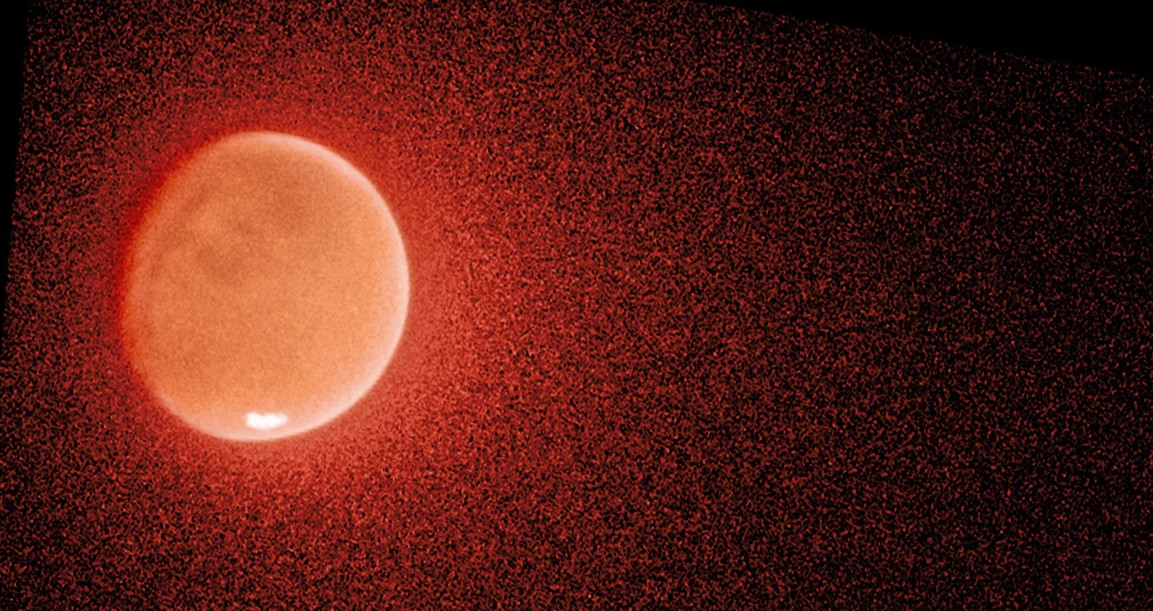
NASA’s Hubble, MAVEN Help Solve the Mystery of Mars’ Escaping Water
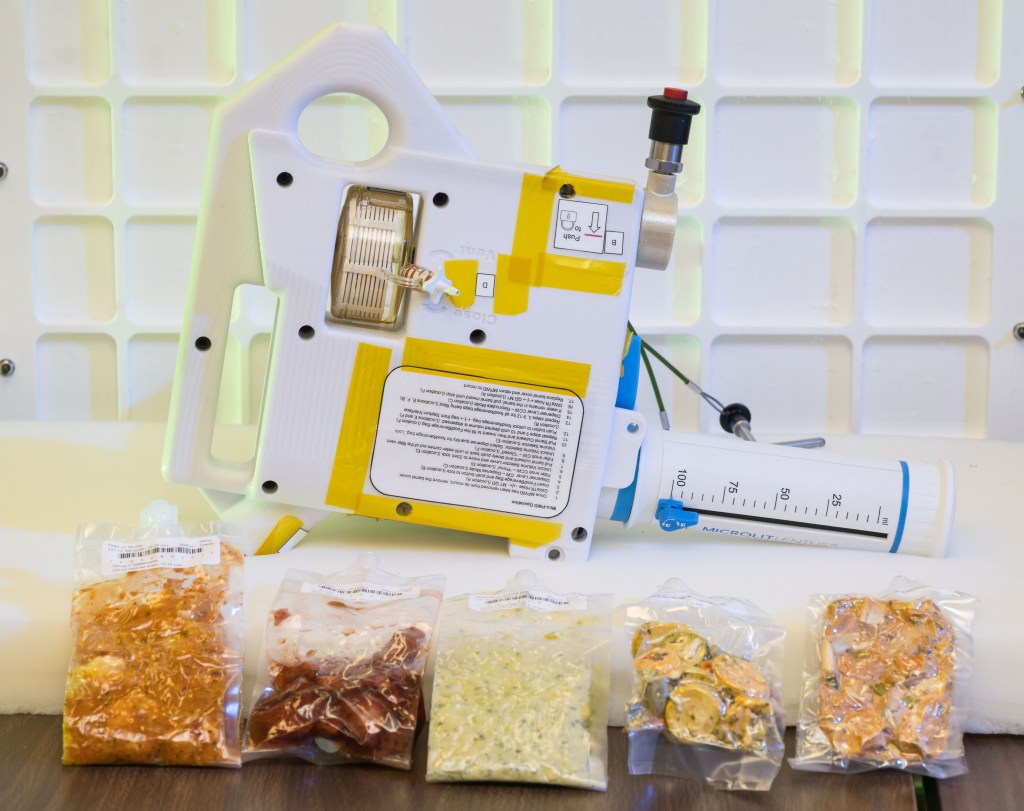
Artemis IV: Gateway Gadget Fuels Deep Space Dining
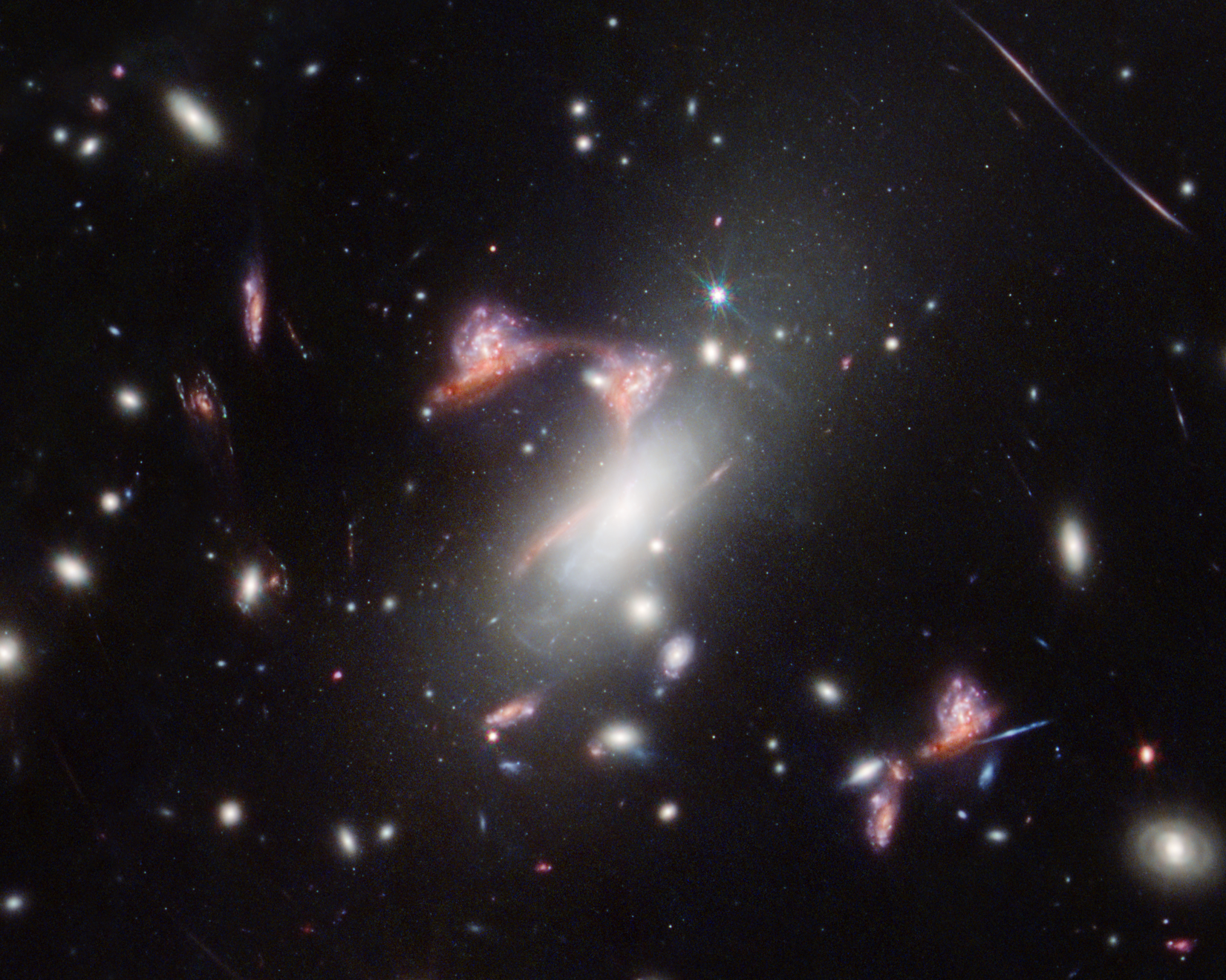
NASA’s Webb Reveals Distorted Galaxy Forming Cosmic Question Mark
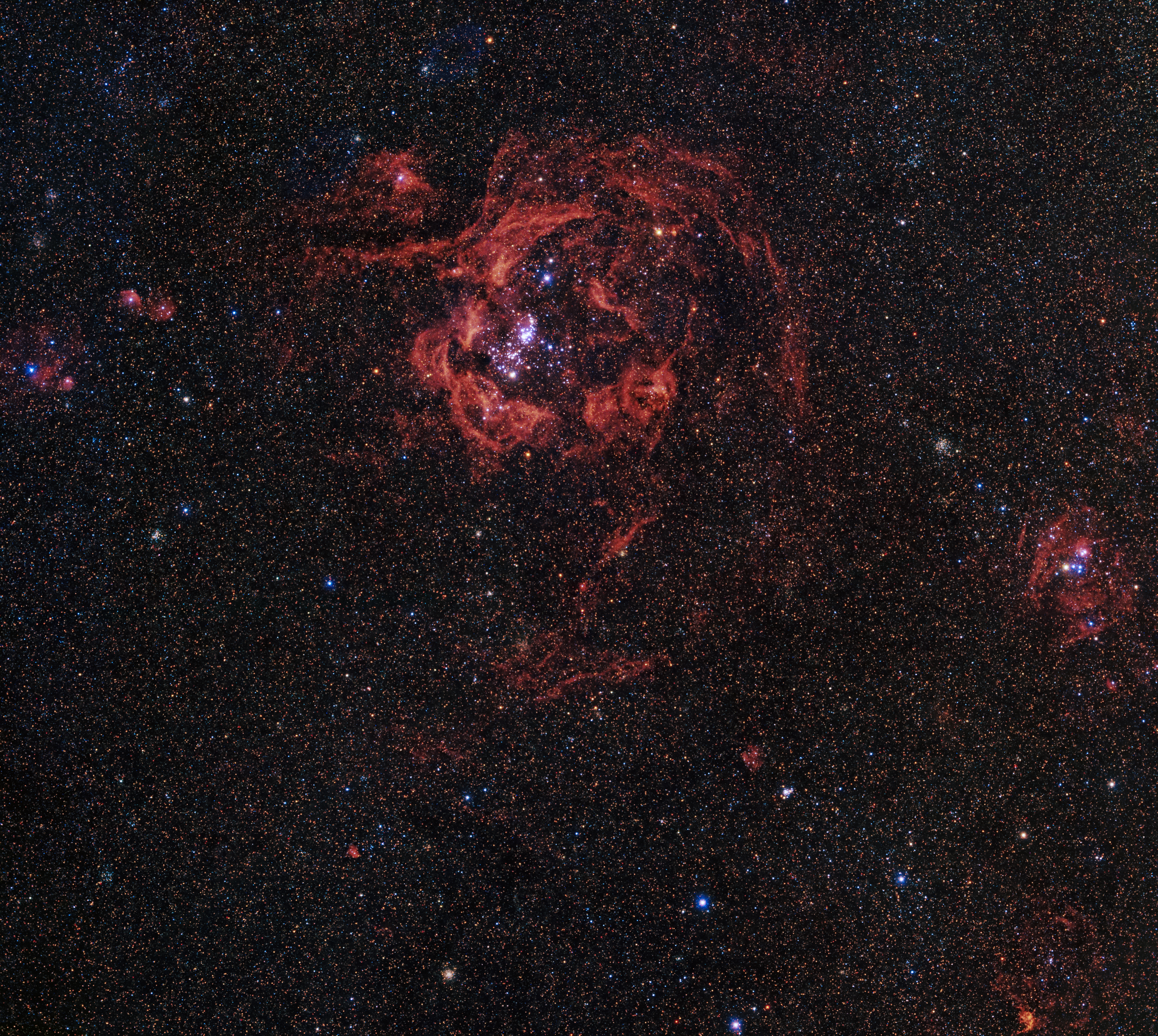
Hubble Zooms into the Rosy Tendrils of Andromeda
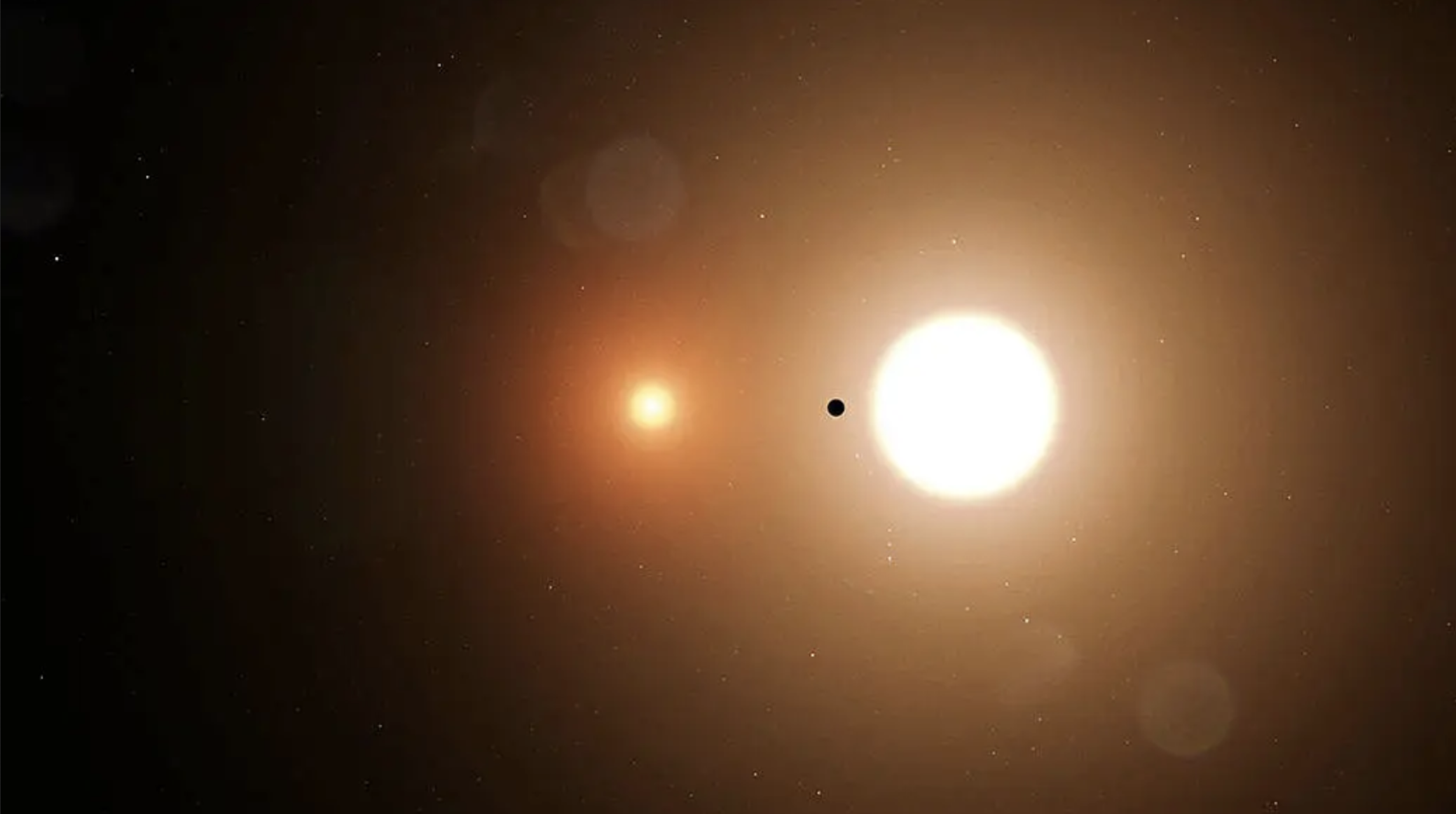
Join the Eclipsing Binary Patrol and Spot Rare Stellar Pairs!
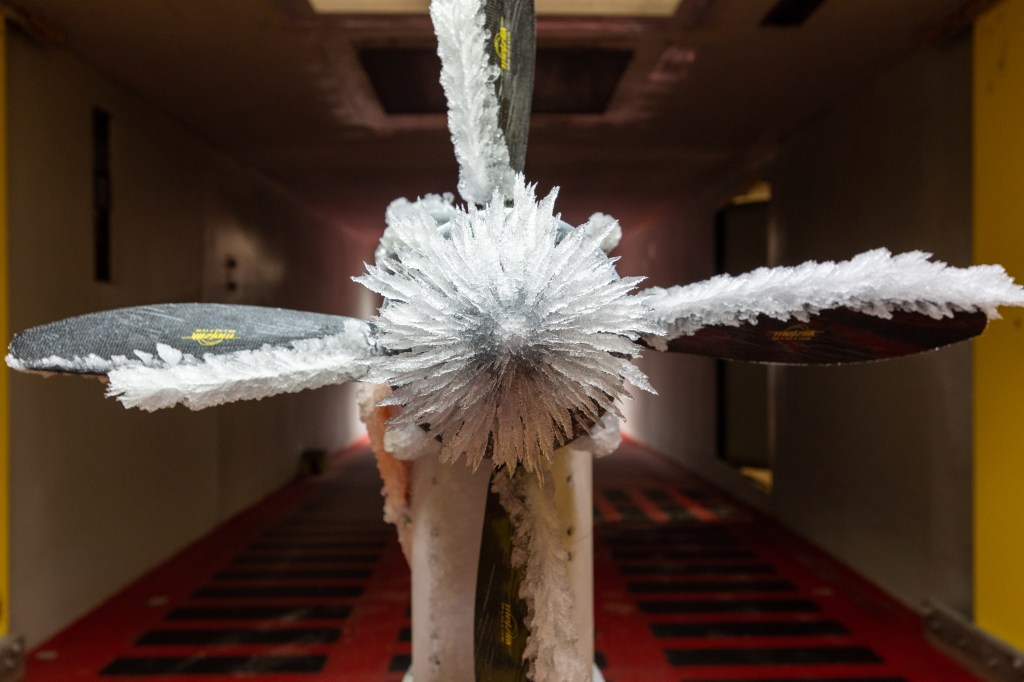
NASA Tunnel Generates Decades of Icy Aircraft Safety Data

Research Plane Dons New Colors for NASA Hybrid Electric Flight Tests
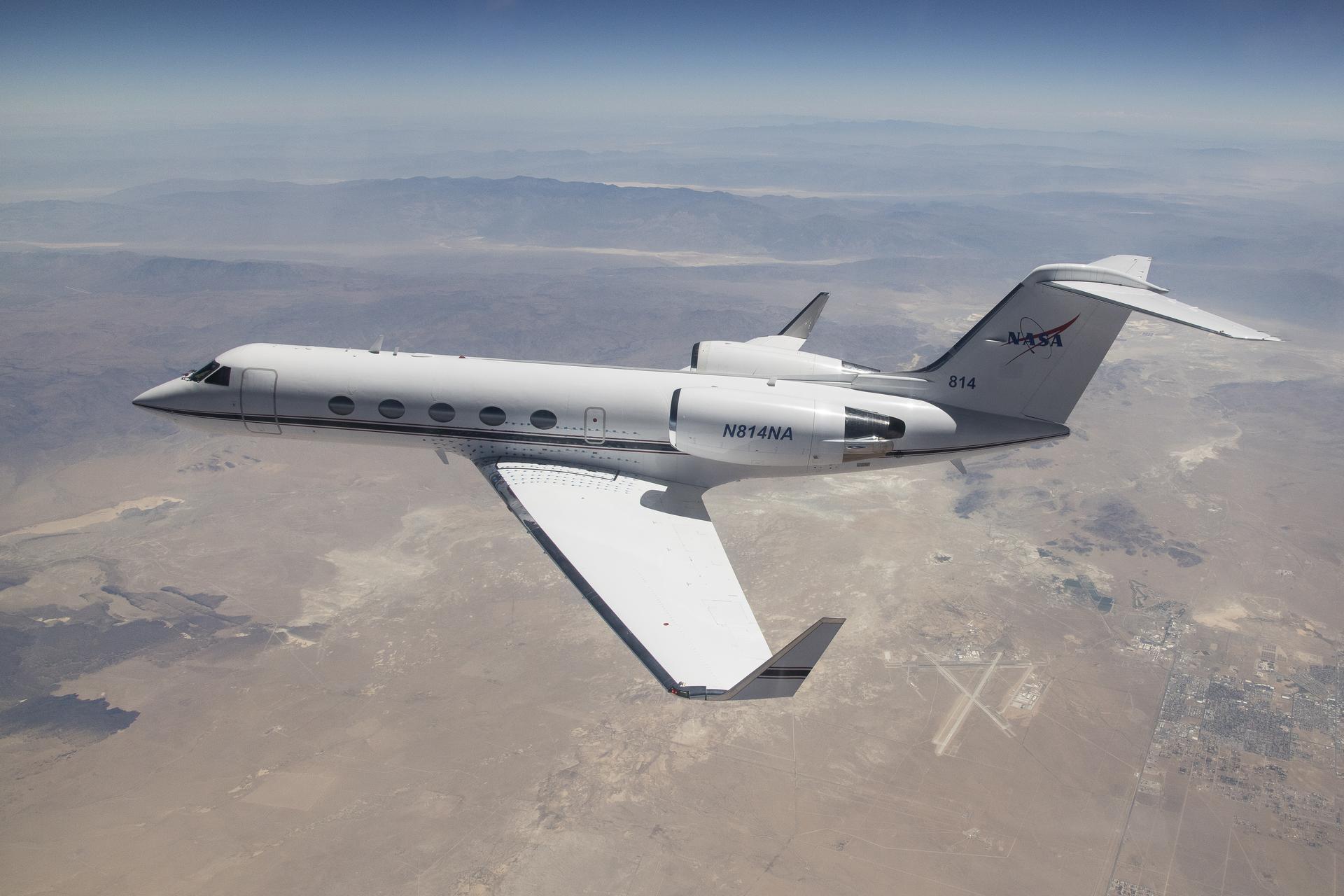
NASA G-IV Plane Will Carry Next-Generation Science Instrument

NASA to Support DARPA Robotic Satellite Servicing Program

NASA JPL Developing Underwater Robots to Venture Deep Below Polar Ice
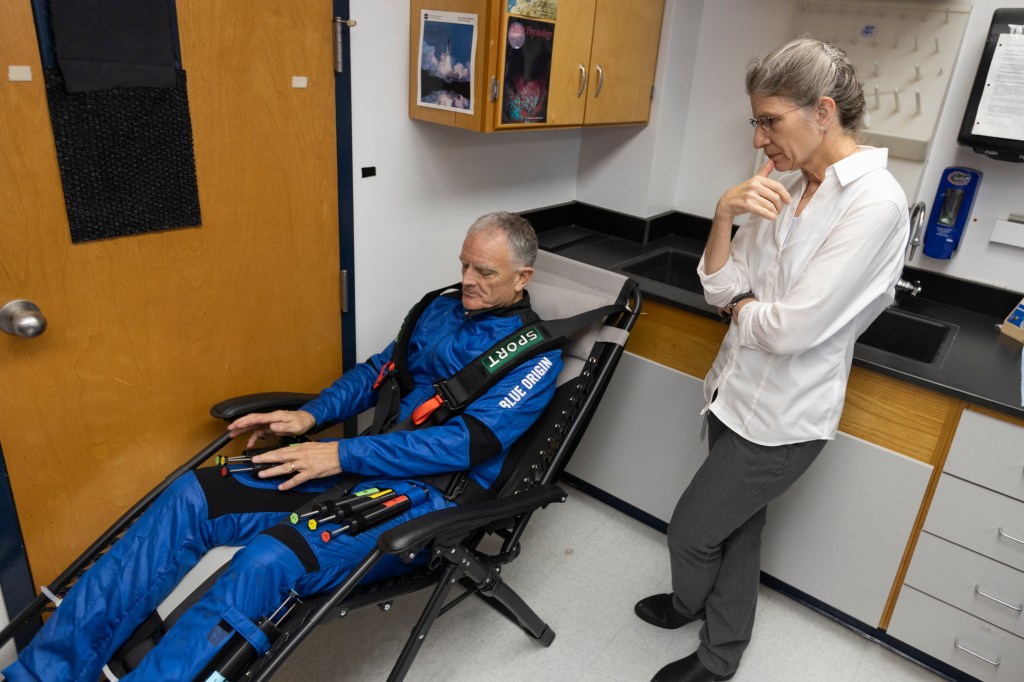
First NASA-Supported Researcher to Fly on Suborbital Rocket

Learn Math with NASA Science

Eclipses Create Atmospheric Gravity Waves, NASA Student Teams Confirm
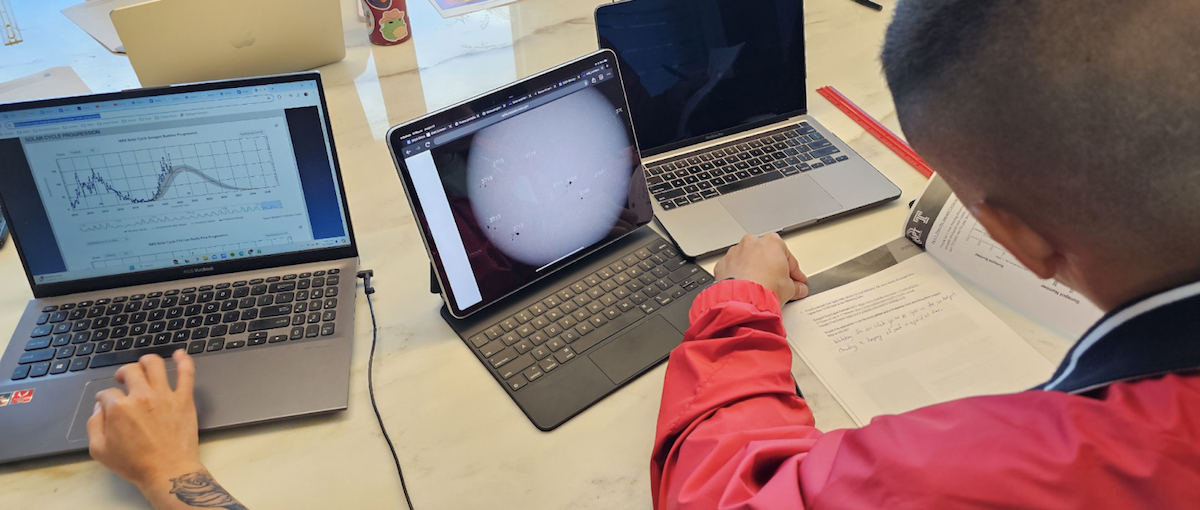
Leveraging Teacher Leaders to Share the Joy of NASA Heliophysics
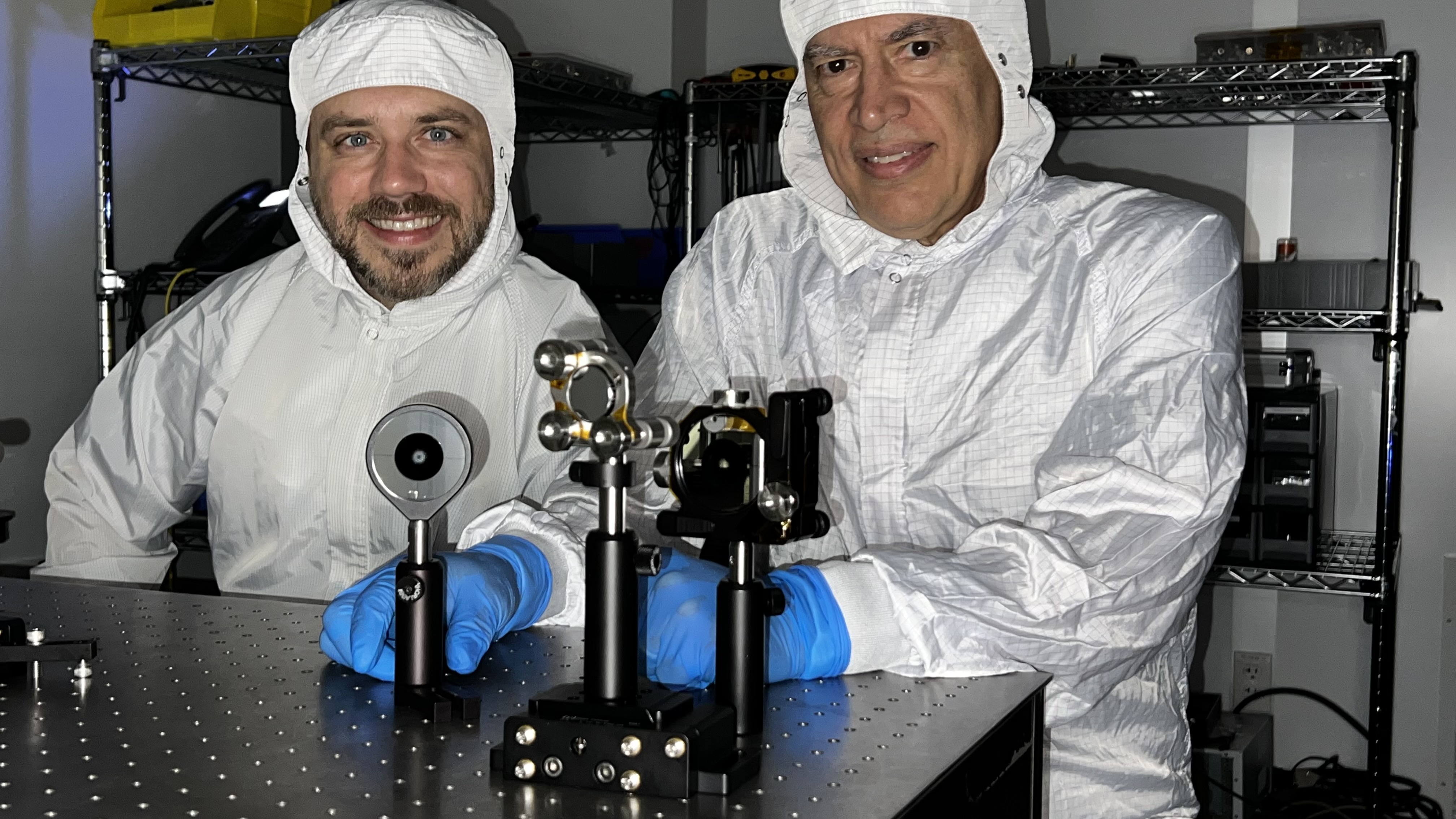
Carbon Nanotubes and the Search for Life on Other Planets
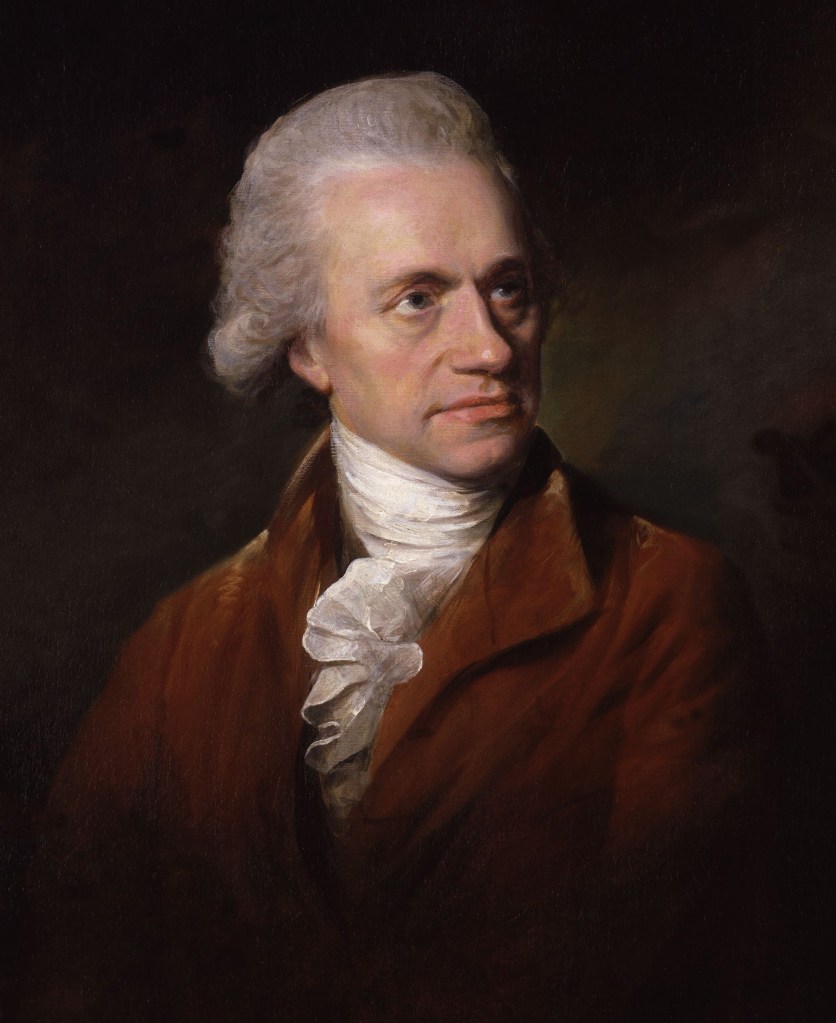
235 Years Ago: Herschel Discovers Saturn’s Moon Enceladus

La NASA invita a los medios al lanzamiento de Europa Clipper

El X-59 de la NASA avanza en las pruebas de preparación para volar

La NASA invita a creadores de las redes sociales al lanzamiento de la misión Europa Clipper
Space travel.
The path to the Moon, Mars, and beyond requires technologies to get us where we need to go quickly, safely and efficiently. Space travel includes launch and in-space propulsion systems, cryogenic fluid management, and thermal management, as well as navigation and landing systems to get our supplies, equipment, and robotic or human explorers to diverse surface destinations.
Quick Facts
NASA’s Space Launch System is 17 feet taller than the Statue of Liberty and produced 15% more thrust than the Saturn V at liftoff.
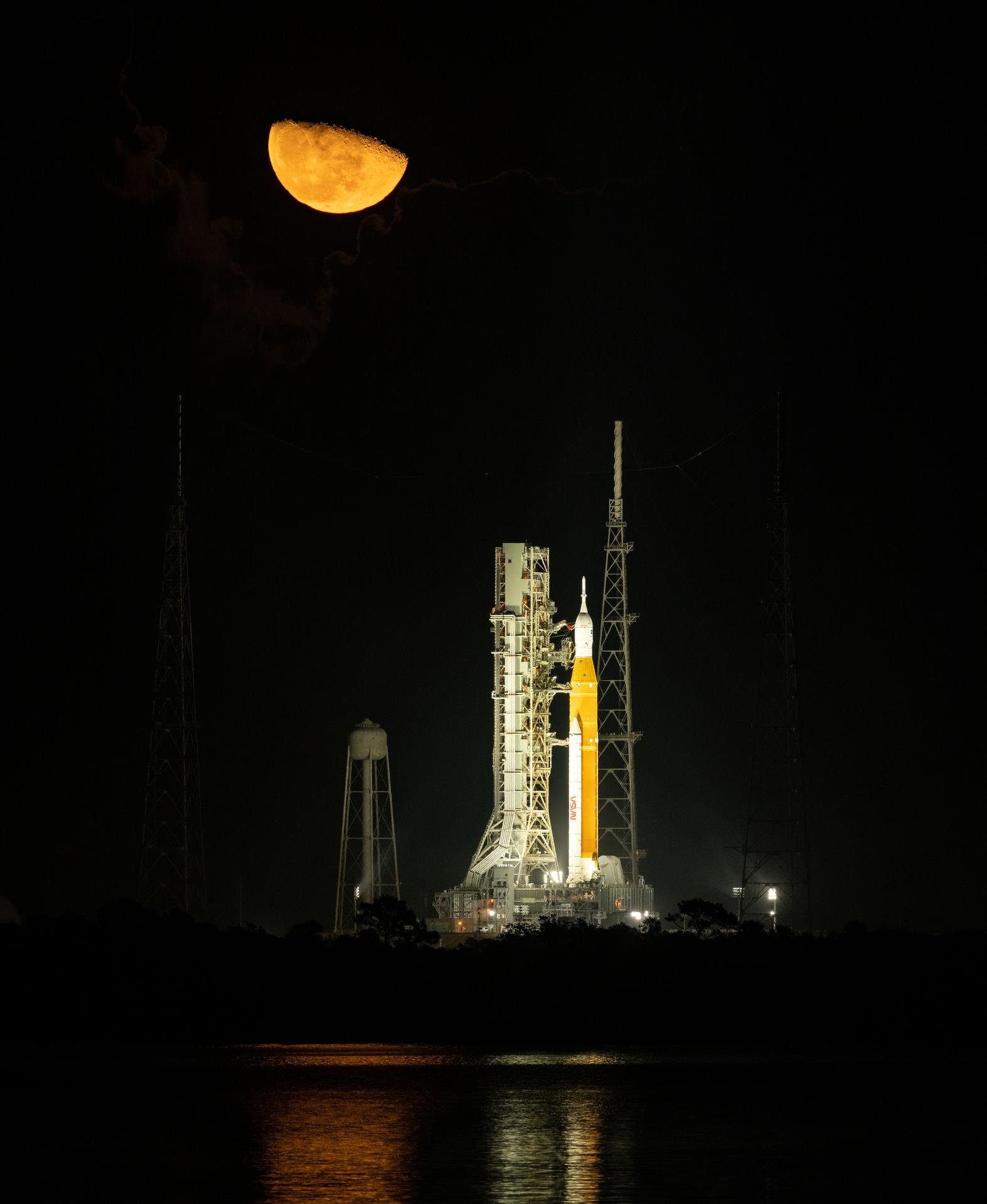
The last nuclear thermal rocket engine tests conducted by the United States occurred more than 50 years ago. NASA and DARPA are partnering on the Demonstration Rocket for Agile Cislunar Operations, or DRACO, program and together, we’ll develop and demonstrate advanced nuclear thermal propulsion technology as soon as 2027.
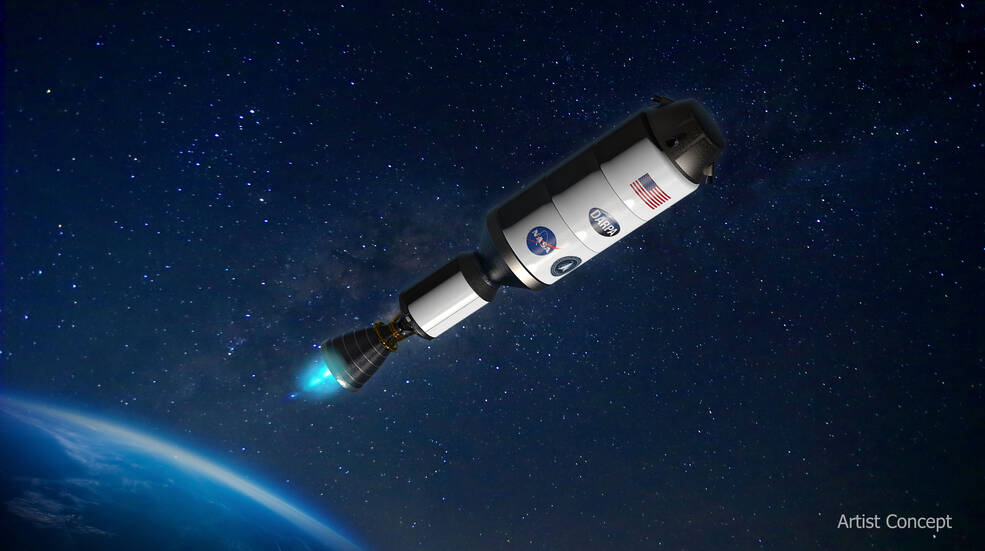
Mission and Impact
NASA seeks to improve our ability to access and travel through space; land more mass in more locations throughout the solar system; live and work in deep space and on planetary bodies; build next generation air vehicles, and transform the ability to observe the universe and answer profound questions in earth and space sciences.
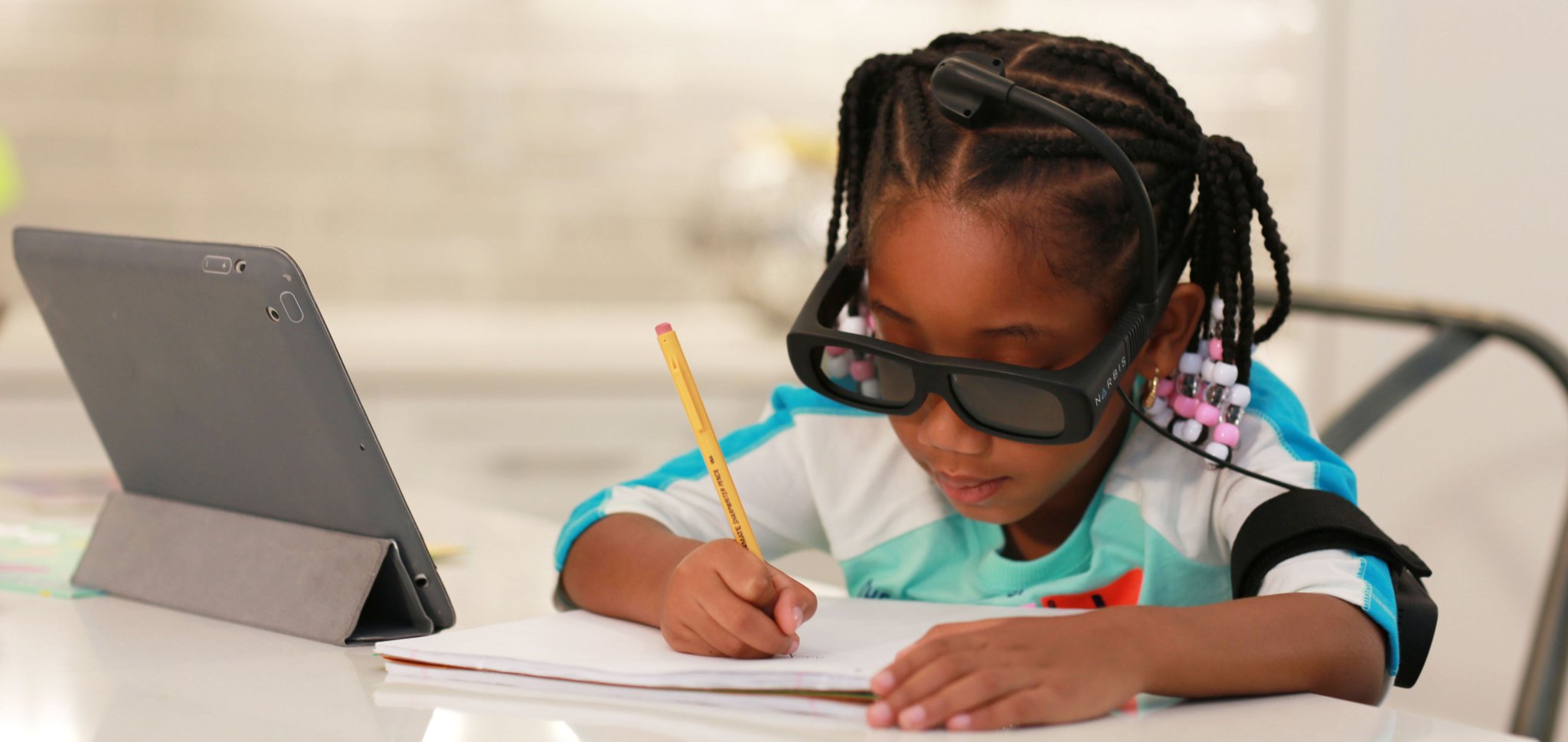
Latest Space Travel News
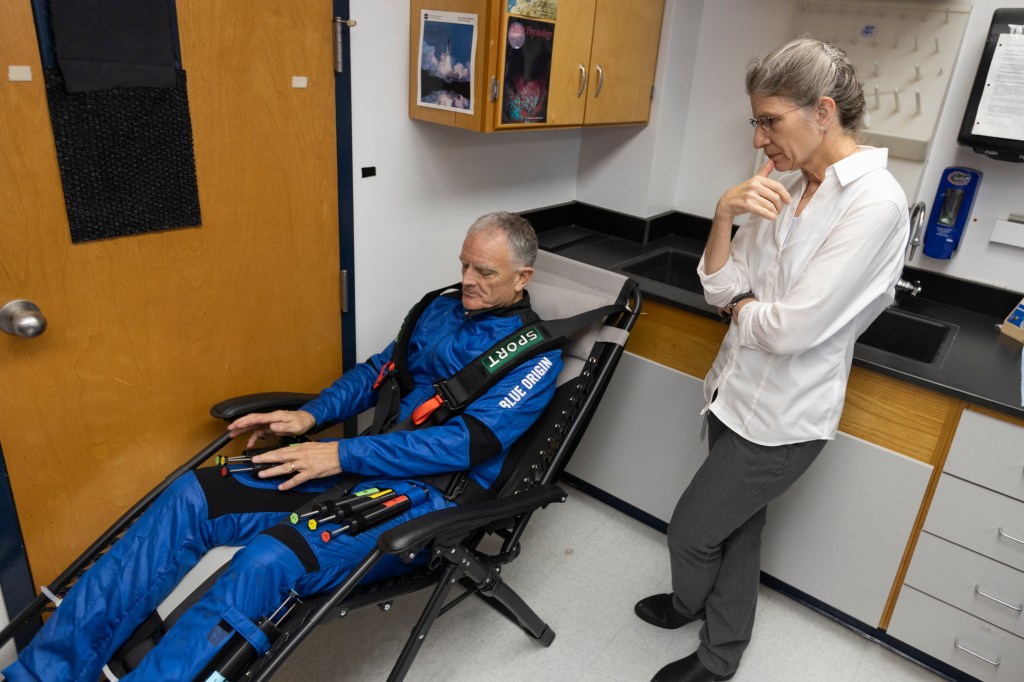
Station Science Top News: August 16, 2024
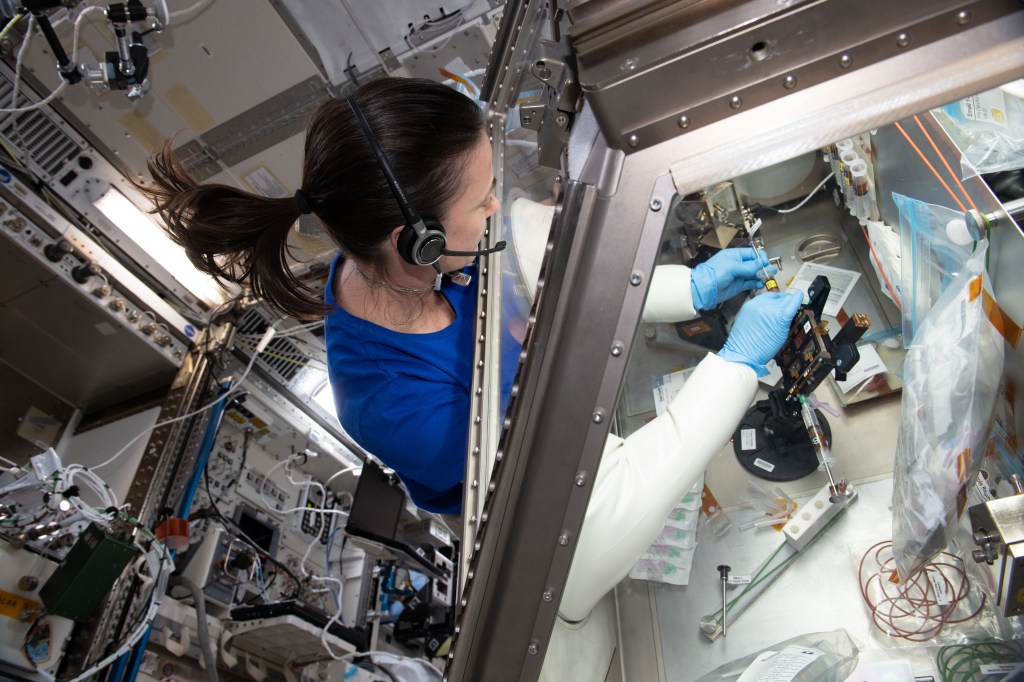
Perseverance Pays Off for Student Challenge Winners
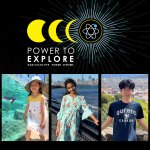
NASA Announces Winners of Power to Explore Challenge
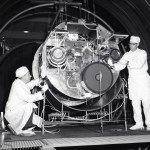
NASA’s SERT II: ‘A Genuine Space Success Story’
How Do Spacecraft Slow Down? We Asked a NASA Technologist
How do spacecraft slow down? Rigid heat shields and retropropulsion have been the favorites of engineers for years. Now NASA is testing a new inflatable heat shield technology that could allow us to carry even larger payloads to worlds with atmospheres.
NASA Technology
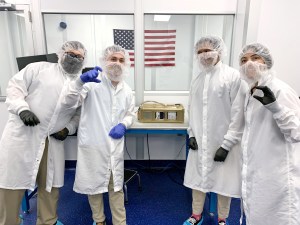
Explore Technology Areas
Space Technology Mission Directorate
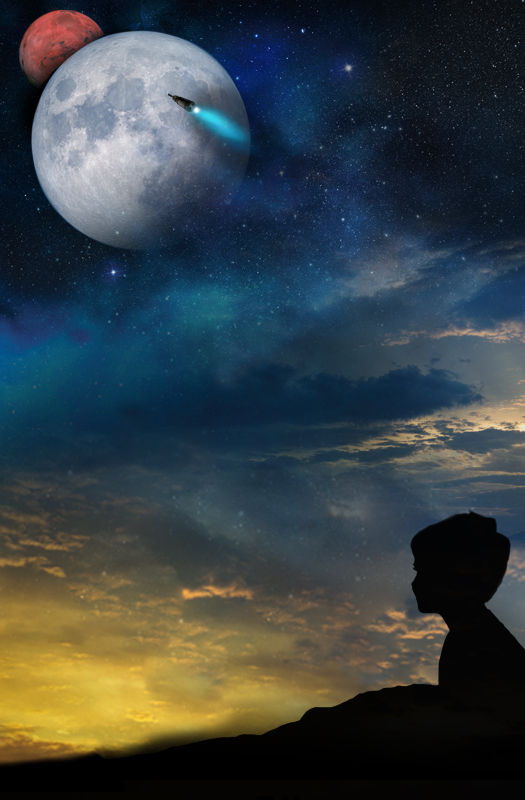
@NASA_Technology
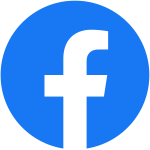
@NASATechnology
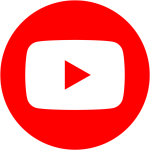
NASA Space Tech Channel
Who are you?* —Please choose an option— Large Corporation Future Entrepreneur Public Institution or Academia Investor SME or Start-up Other
First name*
Company / Institution
Country* Select Country Afghanistan Aland Islands Albania Algeria American Samoa Andorra Angola Anguilla Antarctica Antigua And Barbuda Argentina Armenia Aruba Australia Austria Azerbaijan Bahamas The Bahrain Bangladesh Barbados Belarus Belgium Belize Benin Bermuda Bhutan Bolivia Bosnia and Herzegovina Botswana Bouvet Island Brazil British Indian Ocean Territory Brunei Bulgaria Burkina Faso Burundi Cambodia Cameroon Canada Cape Verde Cayman Islands Central African Republic Chad Chile China Christmas Island Cocos (Keeling) Islands Colombia Comoros Congo Congo The Democratic Republic Of The Cook Islands Costa Rica Cote D'Ivoire (Ivory Coast) Croatia (Hrvatska) Cuba Cyprus Czech Republic Denmark Djibouti Dominica Dominican Republic East Timor Ecuador Egypt El Salvador Equatorial Guinea Eritrea Estonia Ethiopia Falkland Islands Faroe Islands Fiji Islands Finland France French Guiana French Polynesia French Southern Territories Gabon Gambia The Georgia Germany Ghana Gibraltar Greece Greenland Grenada Guadeloupe Guam Guatemala Guernsey and Alderney Guinea Guinea-Bissau Guyana Haiti Heard and McDonald Islands Honduras Hong Kong S.A.R. Hungary Iceland India Indonesia Iran Iraq Ireland Israel Italy Jamaica Japan Jersey Jordan Kazakhstan Kenya Kiribati Korea North Korea South Kuwait Kyrgyzstan Laos Latvia Lebanon Lesotho Liberia Libya Liechtenstein Lithuania Luxembourg Macau S.A.R. Macedonia Madagascar Malawi Malaysia Maldives Mali Malta Man (Isle of) Marshall Islands Martinique Mauritania Mauritius Mayotte Mexico Micronesia Moldova Monaco Mongolia Montenegro Montserrat Morocco Mozambique Myanmar Namibia Nauru Nepal Netherlands Antilles Netherlands The New Caledonia New Zealand Nicaragua Niger Nigeria Niue Norfolk Island Northern Mariana Islands Norway Oman Pakistan Palau Palestinian Territory Occupied Panama Papua new Guinea Paraguay Peru Philippines Pitcairn Island Poland Portugal Puerto Rico Qatar Reunion Romania Russia Rwanda Saint Helena Saint Kitts And Nevis Saint Lucia Saint Pierre and Miquelon Saint Vincent And The Grenadines Saint-Barthelemy Saint-Martin (French part) Samoa San Marino Sao Tome and Principe Saudi Arabia Senegal Serbia Seychelles Sierra Leone Singapore Slovakia Slovenia Solomon Islands Somalia South Africa South Georgia South Sudan Spain Sri Lanka Sudan Suriname Svalbard And Jan Mayen Islands Swaziland Sweden Switzerland Syria Taiwan Tajikistan Tanzania Thailand Togo Tokelau Tonga Trinidad And Tobago Tunisia Turkey Turkmenistan Turks And Caicos Islands Tuvalu Uganda Ukraine United Arab Emirates United Kingdom United States United States Minor Outlying Islands Uruguay Uzbekistan Vanuatu Vatican City State (Holy See) Venezuela Vietnam Virgin Islands (British) Virgin Islands (US) Wallis And Futuna Islands Western Sahara Yemen Zambia Zimbabwe
I consent to the processing of my personal data as specified in the Privacy Policy for the scope of the newsletter
* Mandatory field
How Space supports the Energy Transition
Market trends.
Insights in this article are partly drawn from the Space for Green Growth and Clean Energy Workshop.
The space and energy sectors have met for the first time during the development of solar panels more than 60 years ago.
Today, Space has a key role in monitoring greenhouse gas emissions and its climate impact. In fact, half of all Essential Climate Variables (ECVs) are measured by satellites. The recent findings on air quality changes during the COVID-19 pandemic and discussions on the identification and regulation of methane emission leaks have attracted major attention and continue to drive international and European policy initiatives.
Geostrategic considerations, the need for ensuring energy supply in times of growing energy consumption, energy price volatility, but also the increasing awareness and international commitments for climate change, have led to the creation of the most ambitious set of renewable energy targets in history. In fact, progressively building up a toolbox of policies, Europe is leading the worldwide clean energy revolution. The Green Deal further strengthened existing targets and complemented them with achieving climate neutrality by 2050 (in accordance with the Paris Climate Agreement commitments). It is a dedicated roadmap with a focus on renewable energies (especially off-shore energy), the renovation of buildings (double or triple current renovation rate of 1%), decarbonisation of transport (electrification and 1m public charging points), and increased support for R&D (35% of EU research funding should serve climate-friendly technologies).
The proportion of electricity generation from renewable sources in the EU reached a record high of nearly 40% in 2022. Especially wind and solar power are playing a crucial role, overtaking gas for the first time. The increasing shares of renewables were driven by economic competitiveness, national government support to reach European 2020 renewable targets (on utility-scale as well as self-consumption level) as well as increasing numbers of distributed energy sources.
While public policies, private investments, economies of scale and consumer preferences point all in the direction of more renewable energies, the energy transition creates numerous new challenges for all stakeholders involved: an energy system fed by high shares of variable renewable energies, which themselves depend on changing weather conditions, increases the needs for flexibility and balancing on system level. At the same time, small power producers are replacing large, inflexible power stations leading to a continuous decentralisation and regionalisation of markets. Active consumers not only buy energy any more, but produce, store, and eventually sell generated energy through the network. The increasing electrification and digitalisation of households, in combination with smart-meters and home storage, offers complete new possibilities, but also challenges for existing grids which had been designed to deliver energy from A to B unidirectional and with only limited fluctuations. Smart Grids are needed to ensure storage possibilities and multi-directional flows of energy and information across the European transmission grid (with its 300 000 km of power lines and 355 cross-border lines).
Space added value in supporting the energy transition
With the focus on renewables and an increasing digitisation and data reliability of the energy industry, the role of space technology and satellite derived data is increasing steadily. Energy-as-a-service as well as energy-data-as-a-service are driving forces of the transformation, uniting data from multiple sources, including space.
With the current focus on renewables, the role of space increases on single asset, company and system level. Satellites identify most promising locations for renewables mapping solar geometry and radiation, wind speeds or water flows. The current focus on offshore renewable energy generation including ocean energy and floating wind or solar photovoltaic calls for the strategic collection of remote data such as water temperatures, waves, tidal flows, wind speeds and salinity gradients. Satellites help to limit the environmental impact of new as well as better understand the impact of existing assets on fish, bird, or sea mammals populations.
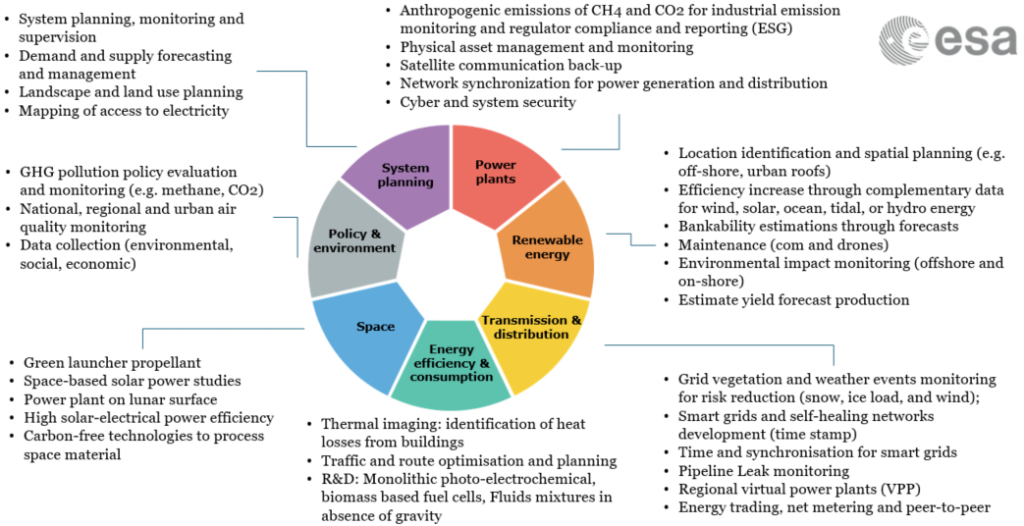
Ready to learn more about space added value for renewable energies? Dive into our recent in depth reports with promising use cases and how ESA programmes help up building European capabilities:
Improving energy generation and forecasts in the short- and long-term through accurate weather data is important for asset managers, banks and local authorities. By the use of real-time and historic data system planners can predict and react flexible to electricity fluctuations on the supply and demand side (generation and consumption peaks). This is especially relevant in remote areas or regions with no or limited access to relevant data and weak infrastructure.
The need for flexibility is heavily felt on grid level where ESA supports smart grids and the general resilience of power lines. Working together with Transmission System Operators and Distribution System Operators on national and European level, ESA supports the development of innovative solution to monitor grid pylon movements, vegetation across power lines, weather events impact (snow, ice load, and wind), grid conductivity and hotpots or man-made interference and constructions. ESA BASS has set up a specific Energy Task Force to follow latest trends and end-user needs. Two‐way communication between smart meters and grid operators and the set-up and maintenance of virtual power plants, net-metering or peer-to-peer networks are further improving system flexibility and balancing. More information can be found in the latest Transmission and Distribution of Electrical Power report .
Space activities are unthinkable without the efficient and responsible use of energy
Building on the progress made in the production of clean hydrogen, ESA is studying options to produce green hydrogen through water electrolysis for the Europe’s Spaceport in French Guiana for both, fuel cells feeding and liquid hydrogen (launcher propellant) production. It is planned to implement the project progressively, starting the production of green hydrogen by 2025, reducing electrical consumption and CO2 emissions while improving dependability and competitiveness.
But not only on the ground, also in space the energy transition is taking place: The International Space Station as well as satellites use solar cells to convert sunlight to electricity and a future moon base will most probably include solar power plants with regenerative systems to store energy for night survival. Feasibility studies include the sustainable use of available resources (e.g. lunar soil) and carbon free technologies to process space materials. The possibilities of re-creating an artificial environment allowing for 100% recycling of waste to produce oxygen, water and food are studied in the frame of MELiSSA (Micro-Ecological Life Support System Alternative). Sustainable and efficient off-the grid solutions are key enabler for human survival in extreme environments and long-range missions.
In the vacuum of space convection, conduction or combustion is not experienced. It is hence a unique environment to study processes related to thermal systems (and to manufacture those). Experiments carried out in the past years aboard the ISS have the potential to revolutionise cooling in electronics and data centres which are consuming approximately 2% of all energy on Earth. Also, fluids mixtures in absence of gravity (e.g. no sedimentation or phase separation) help the oil & gas industry improving their processes related to resource extraction and processing.
Currently, a fascinating concept is experiencing a revival: the study of Space-Based Solar Power harvesting clean energy from space. Solar power satellites benefit from higher solar illumination, unfiltered by atmospheres, and have the potential to mitigate climate change through the provision and transmission of clean energy, in a flexible way, to different remote users on earth, on the moon or on other planets. Studies have shown the potential for such systems to provide 100sMW to 100sGW of power to Earth at a build cost comparable with large terrestrial engineering projects. Benefits for European citizens, for the space sector, and for the planet could be enormous.
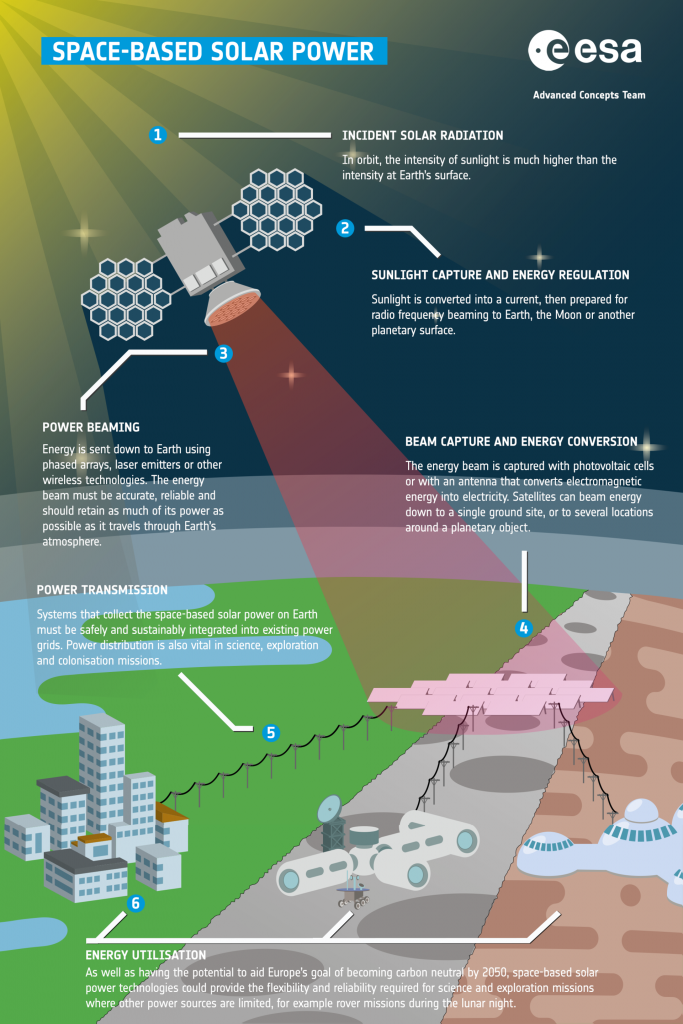
RELATED INDUSTRIES
Related tags, related pages, external page, discover the esa commercialisation days: space forum for green energy, join our newsletter.
And never miss a business opportunity
Don’t miss any news
Your country Select Country Afghanistan Aland Islands Albania Algeria American Samoa Andorra Angola Anguilla Antarctica Antigua And Barbuda Argentina Armenia Aruba Australia Austria Azerbaijan Bahamas The Bahrain Bangladesh Barbados Belarus Belgium Belize Benin Bermuda Bhutan Bolivia Bosnia and Herzegovina Botswana Bouvet Island Brazil British Indian Ocean Territory Brunei Bulgaria Burkina Faso Burundi Cambodia Cameroon Canada Cape Verde Cayman Islands Central African Republic Chad Chile China Christmas Island Cocos (Keeling) Islands Colombia Comoros Congo Congo The Democratic Republic Of The Cook Islands Costa Rica Cote D'Ivoire (Ivory Coast) Croatia (Hrvatska) Cuba Cyprus Czech Republic Denmark Djibouti Dominica Dominican Republic East Timor Ecuador Egypt El Salvador Equatorial Guinea Eritrea Estonia Ethiopia Falkland Islands Faroe Islands Fiji Islands Finland France French Guiana French Polynesia French Southern Territories Gabon Gambia The Georgia Germany Ghana Gibraltar Greece Greenland Grenada Guadeloupe Guam Guatemala Guernsey and Alderney Guinea Guinea-Bissau Guyana Haiti Heard and McDonald Islands Honduras Hong Kong S.A.R. Hungary Iceland India Indonesia Iran Iraq Ireland Israel Italy Jamaica Japan Jersey Jordan Kazakhstan Kenya Kiribati Korea North Korea South Kuwait Kyrgyzstan Laos Latvia Lebanon Lesotho Liberia Libya Liechtenstein Lithuania Luxembourg Macau S.A.R. Macedonia Madagascar Malawi Malaysia Maldives Mali Malta Man (Isle of) Marshall Islands Martinique Mauritania Mauritius Mayotte Mexico Micronesia Moldova Monaco Mongolia Montenegro Montserrat Morocco Mozambique Myanmar Namibia Nauru Nepal Netherlands Antilles Netherlands The New Caledonia New Zealand Nicaragua Niger Nigeria Niue Norfolk Island Northern Mariana Islands Norway Oman Pakistan Palau Palestinian Territory Occupied Panama Papua new Guinea Paraguay Peru Philippines Pitcairn Island Poland Portugal Puerto Rico Qatar Reunion Romania Russia Rwanda Saint Helena Saint Kitts And Nevis Saint Lucia Saint Pierre and Miquelon Saint Vincent And The Grenadines Saint-Barthelemy Saint-Martin (French part) Samoa San Marino Sao Tome and Principe Saudi Arabia Senegal Serbia Seychelles Sierra Leone Singapore Slovakia Slovenia Solomon Islands Somalia South Africa South Georgia South Sudan Spain Sri Lanka Sudan Suriname Svalbard And Jan Mayen Islands Swaziland Sweden Switzerland Syria Taiwan Tajikistan Tanzania Thailand Togo Tokelau Tonga Trinidad And Tobago Tunisia Turkey Turkmenistan Turks And Caicos Islands Tuvalu Uganda Ukraine United Arab Emirates United Kingdom United States United States Minor Outlying Islands Uruguay Uzbekistan Vanuatu Vatican City State (Holy See) Venezuela Vietnam Virgin Islands (British) Virgin Islands (US) Wallis And Futuna Islands Western Sahara Yemen Zambia Zimbabwe
I consent to the processing of my personal data as specified in Privacy Policy for the newsletter scope.

Is Interstellar Travel Really Possible?
Interstellar flight is a real pain in the neck.
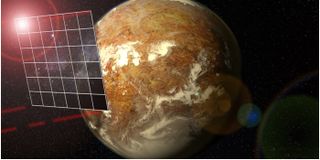
Paul M. Sutter is an astrophysicist at The Ohio State University , host of Ask a Spaceman and Space Radio , and author of " Your Place in the Universe. " Sutter contributed this article to Space.com's Expert Voices: Op-Ed & Insights .
Interstellar space travel . Fantasy of every five-year-old kid within us. Staple of science fiction serials. Boldly going where nobody has gone before in a really fantastic way. As we grow ever more advanced with our rockets and space probes, the question arises: could we ever hope to colonize the stars? Or, barring that far-flung dream, can we at least send space probes to alien planets, letting them tell us what they see?
The truth is that interstellar travel and exploration is technically possible . There's no law of physics that outright forbids it. But that doesn't necessarily make it easy, and it certainly doesn't mean we'll achieve it in our lifetimes, let alone this century. Interstellar space travel is a real pain in the neck.
Related: Gallery: Visions of Interstellar Starship Travel
Voyage outward
If you're sufficiently patient, then we've already achieved interstellar exploration status. We have several spacecraft on escape trajectories, meaning they're leaving the solar system and they are never coming back. NASA's Pioneer missions, the Voyager missions , and most recently New Horizons have all started their long outward journeys. The Voyagers especially are now considered outside the solar system, as defined as the region where the solar wind emanating from the sun gives way to general galactic background particles and dust.
So, great; we have interstellar space probes currently in operation. Except the problem is that they're going nowhere really fast. Each one of these intrepid interstellar explorers is traveling at tens of thousands of miles per hour, which sounds pretty fast. They're not headed in the direction of any particular star, because their missions were designed to explore planets inside the solar system. But if any of these spacecraft were headed to our nearest neighbor, Proxima Centauri , just barely 4 light-years away, they would reach it in about 80,000 years.
I don't know about you, but I don't think NASA budgets for those kinds of timelines. Also, by the time these probes reach anywhere halfway interesting, their nuclear batteries will be long dead, and just be useless hunks of metal hurtling through the void. Which is a sort of success, if you think about it: It's not like our ancestors were able to accomplish such feats as tossing random junk between the stars, but it's probably also not exactly what you imagined interstellar space travel to be like.
Get the Space.com Newsletter
Breaking space news, the latest updates on rocket launches, skywatching events and more!
Related: Superfast Spacecraft Propulsion Concepts (Images)
Speed racer
To make interstellar spaceflight more reasonable, a probe has to go really fast. On the order of at least one-tenth the speed of light. At that speed, spacecraft could reach Proxima Centauri in a handful of decades, and send back pictures a few years later, well within a human lifetime. Is it really so unreasonable to ask that the same person who starts the mission gets to finish it?
Going these speeds requires a tremendous amount of energy. One option is to contain that energy onboard the spacecraft as fuel. But if that's the case, the extra fuel adds mass, which makes it even harder to propel it up to those speeds. There are designs and sketches for nuclear-powered spacecraft that try to accomplish just this, but unless we want to start building thousands upon thousands of nuclear bombs just to put inside a rocket, we need to come up with other ideas.
Perhaps one of the most promising ideas is to keep the energy source of the spacecraft fixed and somehow transport that energy to the spacecraft as it travels. One way to do this is with lasers. Radiation is good at transporting energy from one place to another, especially over the vast distances of space. The spacecraft can then capture this energy and propel itself forward.
This is the basic idea behind the Breakthrough Starshot project , which aims to design a spacecraft capable of reaching the nearest stars in a matter of decades. In the simplest outline of this project, a giant laser on the order of 100 gigawatts shoots at an Earth-orbiting spacecraft. That spacecraft has a large solar sail that is incredibly reflective. The laser bounces off of that sail, giving momentum to the spacecraft. The thing is, a 100-gigawatt laser only has the force of a heavy backpack. You didn't read that incorrectly. If we were to shoot this laser at the spacecraft for about 10 minutes, in order to reach one-tenth the speed of light, the spacecraft can weigh no more than a gram.
That's the mass of a paper clip.
Related: Breakthrough Starshot in Pictures: Laser-Sailing Nanocraft to Study Alien Planets
A spaceship for ants
This is where the rubber meets the interstellar road when it comes to making spacecraft travel the required speeds. The laser itself, at 100 gigawatts, is more powerful than any laser we've ever designed by many orders of magnitude. To give you a sense of scale, 100 gigawatts is the entire capacity of every single nuclear power plant operating in the United States combined.
And the spacecraft, which has to have a mass no more than a paper clip, must include a camera, computer, power source, circuitry, a shell, an antenna for communicating back home and the entire lightsail itself.
That lightsail must be almost perfectly reflective. If it absorbs even a tiny fraction of that incoming laser radiation it will convert that energy to heat instead of momentum. At 100 gigawatts, that means straight-up melting, which is generally considered not good for spacecraft.
Once accelerated to one-tenth the speed of light, the real journey begins. For 40 years, this little spacecraft will have to withstand the trials and travails of interstellar space. It will be impacted by dust grains at that enormous velocity. And while the dust is very tiny, at those speeds motes can do incredible damage. Cosmic rays, which are high-energy particles emitted by everything from the sun to distant supernova, can mess with the delicate circuitry inside. The spacecraft will be bombarded by these cosmic rays non-stop as soon as the journey begins.
Is Breakthrough Starshot possible? In principle, yes. Like I said above, there's no law of physics that prevents any of this from becoming reality. But that doesn't make it easy or even probable or plausible or even feasible using our current levels of technology (or reasonable projections into the near future of our technology). Can we really make a spacecraft that small and light? Can we really make a laser that powerful? Can a mission like this actually survive the challenges of deep space?
The answer isn't yes or no. The real question is this: are we willing to spend enough money to find out if it's possible?
- Building Sails for Tiny Interstellar Probes Will Be Tough — But Not Impossible
- 10 Exoplanets That Could Host Alien Life
- Interstellar Space Travel: 7 Futuristic Spacecraft to Explore the Cosmos
Learn more by listening to the episode "Is interstellar travel possible?" on the Ask A Spaceman podcast, available on iTunes and on the Web at http://www.askaspaceman.com . Thanks to @infirmus, Amber D., neo, and Alex V. for the questions that led to this piece! Ask your own question on Twitter using #AskASpaceman or by following Paul @PaulMattSutter and facebook.com/PaulMattSutter .
Follow us on Twitter @Spacedotcom or Facebook .
Join our Space Forums to keep talking space on the latest missions, night sky and more! And if you have a news tip, correction or comment, let us know at: [email protected].
Paul M. Sutter is an astrophysicist at SUNY Stony Brook and the Flatiron Institute in New York City. Paul received his PhD in Physics from the University of Illinois at Urbana-Champaign in 2011, and spent three years at the Paris Institute of Astrophysics, followed by a research fellowship in Trieste, Italy, His research focuses on many diverse topics, from the emptiest regions of the universe to the earliest moments of the Big Bang to the hunt for the first stars. As an "Agent to the Stars," Paul has passionately engaged the public in science outreach for several years. He is the host of the popular "Ask a Spaceman!" podcast, author of "Your Place in the Universe" and "How to Die in Space" and he frequently appears on TV — including on The Weather Channel, for which he serves as Official Space Specialist.
Blue Origin droneship arrives in Florida ahead of 1st New Glenn launch (video, photos)
Lego City Modular Space Station review
Hubble Telescope spies a very sparkly mini-galaxy (image)
Most Popular
- 2 Celestron Origin Intelligent Home Observatory smart telescope review
- 3 Blue Origin droneship arrives in Florida ahead of 1st New Glenn launch (video, photos)
- 4 Lego City Modular Space Station review
- 5 'There was some tension in the room', NASA says of decision to bring Boeing's Starliner spacecraft home without astronauts
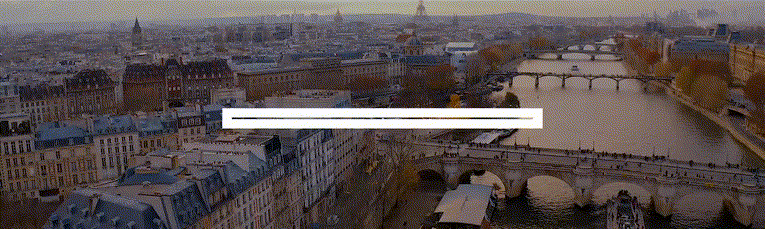
IMAGES
VIDEO
COMMENTS
A space solar power prototype has demonstrated its ability to wirelessly beam power through space and direct a detectable amount of energy toward Earth for the first time. The experiment proves ...
Deep space travel energy sources. Abstract: Exploration of the planets beyond Mars and their surroundings is already planned. Astronomy researchers are citing important information that can be obtained with instrumented spacecraft that fly beyond the planets of our solar system. Spacecraft flying these missions need power for performing their ...
NASA. NASA announced a new style of nuclear generator last week, one that may become a permanent fixture on lunar outposts or deep-space missions in the coming decades. A dependable power source is the name of the game in solar-system exploration. Here among the inner planets, there's ample power to be had in the form of solar radiation.
Countries around the world are investing in space-based solar power research and development, and international organizations are focused on reducing carbon emissions to net-zero by 2050. NASA is considering how best to support space-based solar power development. " Space-Based Solar Power," a new report from the NASA's Office of ...
NASA wants to use the sun to power future deep space missions. Solar energy can make space travel more fuel-efficient. By. Tatyana Woodall. November 25, 2021. NASA/JPL-Caltech/ASU. In August 2022 ...
Newton's Third Law in Space. Chemical propulsion uses a fuel and an oxidizer, converting energy stored in the chemical bonds of the propellants, to produce a short, powerful thrust, or what we see as fire. It's loud and exciting, but not all that efficient. An electric propulsion system uses energy collected by either solar arrays (solar ...
2. Today, energy is possibly one of the most critical sources for humanity. On Earth, we make use of different energy resources such as fossil fuels (coal, oil, etc.), nuclear power, and renewable energy (solar, wind, geothermal, etc.). Similarly, space missions require power at different stages of their life cycle, and they require their own ...
Renewable energy for spaceship Earth. 11545 views 37 likes. ESA / Enabling & Support / Preparing for the Future / Space for Earth / Energy. Earth has never been more energy-hungry, but for supply to keep pace with demand, new technology is needed. Technologies for space missions - including power supply and management systems - are helping ...
By. Elizabeth Gibney. The European Space Agency is investigating whether orbiting solar arrays could beam renewable energy to Earth, as shown in this artist's illustration. Credit: European SPS ...
Deep space travel energy sources ... Sunlight in these zones is so weak that alternative energy sources are needed. An alternative power source for deep-space missions is radioisotope heated energy converters.. The choice of heat-to-electric power conversion is narrowing to: 1) the Stirling engine; and 2) a combined cycle with thermionic and ...
v Energy for Space: Department of Energy's Strategy to Advance American Space Leadership SNPP Space Nuclear Power and Propulsion SPD Space Policy Directive SPP Strategic Partnership Projects SSA Space Situational Awareness STEM Science, Technology, Engineering and Mathematics S&T Science and Technology TRISO Tristructural-Isotropic (Nuclear Fuel) USAF U.S. Air Force
The nuclear fusion feat has broad implications, fueling hopes of clean, limitless energy. As for space exploration, one upshot from the landmark research is attaining the long-held dream of future ...
Fuel Cells. Apollo and the space shuttle both used hydrogen-oxygen fuel cells for electrical power. Fuel cells combine two chemical components at a controlled rate to produce heat, electricity, and some chemical waste product. In the case of hydrogen-oxygen fuel cells, this waste produce is water, which could be used by the crew.
energy demands of space missions, offering advantages over t raditional energy sources [Slough et al., 2012]. The devel opment of fusi on ene rgy for space travel has long be en
Energy storage for space travel is the next frontier for exploring the cosmos. Meet some of the companies innovating batteries, capacitors, and fuel cells. ... The system also includes a pyrotechnic, thermal energy source for "igniting" the battery. After activation, the battery quickly reaches peak voltage and functions until a critical ...
Well, at least not on Earth. Since it's Space Week, we thought it'd be appropriate to look at one promising, but futuristic, idea that could change the face of solar power generation: Space-Based Solar Power (SBSP). While the Energy Department is not actively researching SBSP, we hope you'll take a moment to learn about this far out concept.
An alternative power source for deep-space missions is radioisotope heated energy converters.. The choice of heat-to-electric power conversion is narrowing to: 1) the Stirling engine; and 2) a ...
Renewable Energy That's Plausible For Space Travel. Right now, you might be running through the short list of all the renewable energy sources and weighing which ones could possibly work in space. Well, there are a slim few. Wind power is obviously a bust, because, no wind. Geothermal is also a no go for obvious reasons, and hydroelectric ...
Sunlight in these zones is so weak that alternative energy sources are needed. An alternative power source for deep-space missions is radioisotope heated energy converters.. ... Deep space travel energy sources @article{Oman2003DeepST, title={Deep space travel energy sources}, author={Henry Oman}, journal={IEEE Aerospace and Electronic Systems ...
Space Travel. The path to the Moon, Mars, and beyond requires technologies to get us where we need to go quickly, safely and efficiently. Space travel includes launch and in-space propulsion systems, cryogenic fluid management, and thermal management, as well as navigation and landing systems to get our supplies, equipment, and robotic or human ...
Energy-as-a-service as well as energy-data-as-a-service are driving forces of the transformation, uniting data from multiple sources, including space. With the current focus on renewables, the role of space increases on single asset, company and system level. Satellites identify most promising locations for renewables mapping solar geometry and ...
Perhaps one of the most promising ideas is to keep the energy source of the spacecraft fixed and somehow transport that energy to the spacecraft as it travels. One way to do this is with lasers.
Fusion is renewable if you are going fast enough. The Bussard Ram Jet can collect interstellar hydrogen as you travel. From here:. Bussard1 proposed a ramjet variant of a fusion rocket capable of reasonable interstellar travel, using enormous electromagnetic fields (ranging from kilometers to many thousands of kilometers in diameter) as a ram scoop to collect and compress hydrogen from the ...