- Type 2 Diabetes
- Heart Disease
- Digestive Health
- Multiple Sclerosis
- COVID-19 Vaccines
- Occupational Therapy
- Healthy Aging
- Health Insurance
- Public Health
- Patient Rights
- Caregivers & Loved Ones
- End of Life Concerns
- Health News
- Thyroid Test Analyzer
- Doctor Discussion Guides
- Hemoglobin A1c Test Analyzer
- Lipid Test Analyzer
- Complete Blood Count (CBC) Analyzer
- What to Buy
- Editorial Process
- Meet Our Medical Expert Board
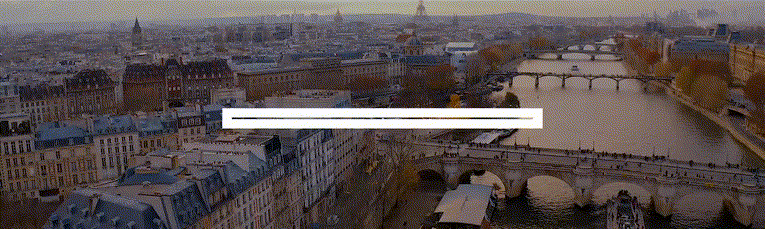
The Anatomy of the Spinal Cord
It forms the nervous system connection between the brain and the body
Associated Conditions
Rehabilitation.
The spinal cord is part of the central nervous system. This long structure runs down the center of your back, and it mediates messages between the brain and the peripheral nerves. The spinal cord is primarily composed of nerves, which are organized in systematic pathways, also described as tracts.
The spine (backbone) encloses and protects the spinal cord. Damage to the spinal cord can occur as a result of problems such as traumatic injuries, infections, and disease. Treatment for conditions that affect the spinal cord often includes rehabilitation and may also involve medication and/or surgery.
The spinal cord is adjacent to and below the medulla, which is the lowest part of the brain. The top region of the spinal cord extends down from the medulla all the way to the lower back.
Throughout the spinal cord, there is a consistent arrangement of nerves. The sensory nerve pathways are located toward the posterior (back) of the spinal cord, while the motor nerve pathways run along the lateral (sides) and anterior (front) regions of the spinal cord.
Cerebrospinal fluid (CSF) , with nutrients and immune cells, flows around the spinal cord. Meninges, which are layers of protective connective tissue, surround the spinal cord and CSF.
Meninges are composed of three thin layers—the innermost pia mater, the middle arachnoid mater, and the outermost dura mater. All of these structures—the spinal cord, CSF, and meninges—are enclosed in the backbone, which is also referred to as the spine and the vertebral column.
Structure and Location
From top to bottom, the regions of the spinal cord include the cervical, thoracic, lumbar, and sacral levels. Each of these levels corresponds to spinal nerves that emerge from the spinal cord toward structures of the body, such as the arms, legs, and trunk.
The deep, central area of the spinal cord is referred to as gray matter, and the portion that is located nearer to the outer edge of the spinal cord is referred to as white matter.
A coating called myelin (a type of fat) insulates all nerves. The white matter tends to have more myelination than the grey matter, giving it a whiter appearance when viewed with a microscope.
The grey matter of the spinal cord is shaped somewhat like an open-winged butterfly lying across the center of the spinal cord. This butterfly-shaped gray matter contains nerve roots. The white matter is composed of several tracts (pathways) that travel up and down the spinal cord.
Regions of the spinal cord include the following.
Anterior Horn
This region is the frontal portion of the gray matter of the spinal cord, and it is composed of nerves that send motor signals to the spinal nerves.
Lateral and Anterior Tracts
These white matter pathways carry motor signals down the spinal cord in the corticospinal tract. This tract travels all the way down the spinal cord at the front and sides of the white matter regions.
Motor control of the voluntary muscles (muscles you choose to move) travels through the spinal cord in the corticospinal tract. Motor signals are initiated in the motor strip, a region of the cerebral cortex of the brain.
These motor signals travel down the internal capsule, and then cross over to the other side of the body in the brain stem. From there, these messages are sent to the anterior horn and the lateral and anterior tracts of the spinal cord. The motor message exits the spinal cord through the ventral root (the front portion) of the spinal nerves.
Dorsal Horn
This area is the posterior region of the grey matter. The spinal nerves deliver sensory messages such as light touch, position sense, and vibration to the dorsal horns.
Posterior Tracts
Also described as the spinothalamic tract, this is a long, white matter pathway that extends all the way up the spine to the brain. The spinal cord mediates sensation coming from the skin, bones, and internal organs.
Your skin detects these sensations and sends messages from peripheral sensory nerves (embedded in the skin) to the spinal nerves, then to the dorsal horn and up through the spinothalamic tracts, crossing over to the other side of the spinal cord before reaching the brain.
Eventually, these messages reach the brain stem, then the thalamus, and then the sensory strip, which is directly behind the motor strip in the cerebral cortex of the brain.
Lateral Horn
The lateral horns of the spinal cord are located at the two sides of the gray matter. This area is composed of nerves that mediate autonomic functions of the body. The autonomic nervous system regulates involuntary functions (actions you don't purposely control), such as digestion and breathing.
The primary role of the spinal cord is to relay sensory, motor, and autonomic messages between the brain and the rest of the body. Myelinated nerves along the pathways of the spinal cord send electrical signals to each other to facilitate these actions.
The motor messages sent through the corticospinal tract eventually reach the corresponding muscle as the spinal nerve branches into smaller peripheral motor nerves that extend to the target muscle. As a result of this nerve stimulation, you can voluntarily move your arms, legs, neck, back, and abdominal muscles.
Your spinal cord sends messages from your peripheral sensory nerves to your brain, allowing you to detect sensations that include light touch, vibration, pain, temperature, and the position of your body.
The spinal cord sends messages to regulate the internal organs of the body. This includes control of smooth muscles, such as the muscles that move your lungs, stomach, intestines, bladder, and uterus.
There are a number of medical problems that can affect the spinal cord. Disease of the spinal cord is often described as myelopathy . These conditions cause impairment of motor, sensory, and/or autonomic function.
Myelopathy also often causes spasticity , which is stiffness of the affected arm and/or leg. The symptoms of any spinal cord problem typically correspond to the section of the spinal cord that is impaired. Sometimes, spinal cord injuries also affect functions that are controlled by areas below the level of spinal cord damage due to disruption of the spinal cord tracts.
Diagnosis of a spinal cord condition can include tests such as a physical examination, spinal imaging, nerve conduction studies (NCV), and/or electromyography (EMG).
Conditions that affect the spine include:
Multiple sclerosis (MS)
This is a demyelinating condition that may affect the brain and/or spine. Multiple sclerosis lesions in the spine can cause weakness, sensory loss, tingling, and pain, and they may affect bowel and bladder function.
Spinal Cord Compression
When the spinal cord is placed under physical pressure , weakness, sensory loss, and autonomic deficits can occur. Severe degenerative disease of the bone or cartilage of the spine can cause these structures to fall out of place—potentially resulting in physical impingement on the spinal cord. Metastatic (spreading throughout the body) cancer can cause spinal cord compression as well.
An injury can cause the spine to move out of place and can even cause a spine fracture (break), which can injure the spinal cord . Injuries may also cause spinal cord compression due to bleeding, and an injury can directly damage the spinal cord.
Amyotrophic Lateral Sclerosis (ALS)
Sometimes called Lou Gehrig's disease , this is a rare condition characterized by the gradual degeneration of the motor neurons located in the spinal cord. ALS causes progressive weakness and, eventually, a complete loss of muscle control. As a result, most individuals affected by ALS need a high level of supportive care.
Currently, there is no cure for ALS. However, medications such as Radicava (edaravone), Rilutek (riluzole), Relyvrio (sodium phenylbutyrate/ taurursodiol), and Qalsody (tofersen) can help relieve the symptoms and improve the quality of life for people with this condition.
An infection or inflammation of the meninges, often described as spinal meningitis, can cause symptoms such as a headache, stiff neck, fever, nausea, and vomiting. Episodes of bacterial meningitis require antibiotics. Other types of meningitis may require anti-inflammatory therapy or other treatments that target the cause.
Vitamin B12 Deficiency
A deficit in this vitamin can cause many medical issues , including anemia, nerve damage, and subacute combined degeneration of the spinal cord, which is a very rare demyelinating condition that can cause weakness, sensory loss, and stiffness.
Spinal cord cancer is not common, but tumors can develop in any region of the spinal cord. Late-stage cancer often metastasizes to the spine and/or spinal cord, causing spinal cord compression. Meningeal carcinomatosis is the spread of cancer cells throughout the meninges and CSF.
Spinal Cord Infarct
If the blood supply to the spinal cord is interrupted, an area of the spine might not receive an adequate supply of blood. This can lead to severe damage, with resulting loss of spinal cord function.
Spinal Muscular Atrophy (SMA)
Spinal muscular atrophy is a hereditary condition that can cause substantial muscle weakness. SMA is characterized by degeneration of the motor neurons in the spinal cord. There are a few treatments used for SMA, including Spinraza (nusinersen) and Zolgensma (onasemnogene abeparvovec).
This contagious viral infection is usually preventable with a vaccine. In some instances, the infection involves one or more regions of the spinal cord, causing muscle paralysis of the areas that are controlled by the affected spinal cord regions.
Spinal cord diseases and injuries typically require medical and/or surgical interventions. Treatment may include steroids to reduce inflammation or antibiotics to target bacterial infections. Certain neurological conditions, such as MS, ALS, and SMA, also can improve with prescription medications indicated for the specific conditions.
If you have spinal cord compression, you may need surgery to reduce pressure on your spinal cord due to cancer or a bone impingement. Cancer may require treatment with chemotherapy and radiation therapy.
Therapies also usually include physical therapy and rehabilitation exercises. Some people may need to use a cane, walker, or wheelchair while recovering from a condition involving the spinal cord.
Cho TA. Spinal cord functional anatomy . Continuum (Minneap Minn) . 2015;21(1 Spinal Cord Disorders):13-35. doi:10.1212/01.CON.0000461082.25876.4a
National Institute of Neurological Disorders and Stroke. Transverse myelitis fact sheet .
Ziu E, Mesfin FB. Cancer, spinal metastasis . StatPearls.
ALS Association. FDA-Approved Drugs for Treating ALS .
National Institute of Neurological Disorders and Stroke. Meningitis and encephalitis .
MedlinePlus. Vitamin B12 .
National Institute for Neurological Disorders and Stroke. Spinal muscular atrophy fact sheet .
Menant JC, Gandevia SC. Poliomyelitis . Handb Clin Neurol . 2018;159:337-344. doi:10.1016/B978-0-444-63916-5.00021-5
By Heidi Moawad, MD Dr. Moawad is a neurologist and expert in brain health. She regularly writes and edits health content for medical books and publications.

An official website of the United States government
The .gov means it's official. Federal government websites often end in .gov or .mil. Before sharing sensitive information, make sure you're on a federal government site.
The site is secure. The https:// ensures that you are connecting to the official website and that any information you provide is encrypted and transmitted securely.
- Publications
- Account settings
- Browse Titles
NCBI Bookshelf. A service of the National Library of Medicine, National Institutes of Health.
StatPearls [Internet]. Treasure Island (FL): StatPearls Publishing; 2024 Jan-.

StatPearls [Internet].
Neuroanatomy, motor neuron.
Lindsay C. Zayia ; Prasanna Tadi .
Affiliations
Last Update: July 24, 2023 .
- Introduction
While the term “motor neuron” evokes the idea that there is only one type of neuron that conducts movement, this is far from the truth. In fact, within the classification of a “motor neuron,” there lies both upper and lower motor neurons, which are entirely different in terms of their origins, synapse points, pathways, neurotransmitters, and lesion characteristics. Overall, motor neurons (or motoneurons) comprise various tightly controlled, complex circuits throughout the body that allows for both voluntary and involuntary movements through the innervation of effector muscles and glands. The upper and lower motor neurons form a two-neuron circuit. The upper motor neurons originate in the cerebral cortex and travel down to the brain stem or spinal cord, while the lower motor neurons begin in the spinal cord and go on to innervate muscles and glands throughout the body. Understanding the difference between upper and lower motor neurons, as well as the pathway that they take, is crucial to being able to not only diagnose these neuronal injuries but also localize the lesions efficiently.
- Structure and Function
The upper and lower motor neurons together comprise a two-neuron pathway that is responsible for movement. Upper and lower motor neurons utilize different neurotransmitters to relay their signals. Upper motor neurons use glutamate, while lower motor neurons use acetylcholine. [1]
To perform a movement, a signal must begin in the primary motor cortex of the brain, which is in the precentral gyrus. In the primary motor cortex are the cell bodies of the upper motor neurons, referred to as Betz cells. [2] Specifically, these cells are located in layer 5 of the motor cortex and have long apical dendrites that branch up into layer 1. [3] The upper motor neuron is responsible for integrating all of the excitatory and inhibitory signals from the cortex and translating it into a signal that will initiate or inhibit voluntary movement. Thalamocortical neurons and callosal projection neurons regulate upper motor neurons. While the mechanism of regulation by these entities is not completely understood, it is thought that the majority of the excitatory input to these neurons comes from neurons located in layers 2, 3, and 5 of the motor cortex. The axons of the upper motor neuron travel down through the posterior limb of the internal capsule. From there, they continue through the cerebral peduncles in the midbrain, longitudinal pontine fibers, and eventually the medullary pyramids. It is at this location that the majority (approximately 90%) of the fibers will decussate and continue down the spinal cord on the contralateral side of the body as the lateral corticospinal tract. The lateral corticospinal tract is the largest descending pathway and is located in the lateral funiculus. This tract will synapse directly onto the lower motor neuron in the anterior horn of the spinal cord. The pyramidal tract fibers that did not decussate at the medulla comprise the anterior corticospinal tract, which is much smaller than the lateral corticospinal tract. This tract is located near the anterior median fissure and is responsible for axial and proximal limb movement and control, which helps with posture. Although it does not decussate at in the medulla, this tract does decussate at the spinal level being innervated. [4] [5] [6]
The lower motor neuron is responsible for transmitting the signal from the upper motor neuron to the effector muscle to perform a movement. There are three broad types of lower motor neurons: somatic motor neurons, special visceral efferent (branchial) motor neurons, and general visceral motor neurons. [1]
Somatic motor neurons are in the brainstem and further divide into three categories: alpha, beta, and gamma. Alpha motor neurons innervate extrafusal muscle fibers and are the primary means of skeletal muscle contraction. The large alpha motor neuron cell body can be either in the brainstem or spinal cord. In the spinal cord, the cell bodies are found in the anterior horn and thus are called anterior horn cells. From the anterior horn cell, a single axon goes on to innervate many muscle fibers within a single muscle. The properties of these muscle fibers are nearly identical, allowing for controlled, synchronous movement of the motor unit upon depolarization of the lower motor neuron. Beta motor neurons are poorly characterized, but it has been established that they innervate both extrafusal and intrafusal fibers. Gamma motor neurons innervate muscle spindles and dictate their sensitivity. These neurons primarily respond to stretch of the muscle spindle. Despite being named a “motor neuron,” these neurons do not directly cause any motor function. It is thought that they get activated along with alpha motor neurons and fine-tune the muscle contraction (alpha-gamma coactivation). A disruption in either alpha or gamma motor neurons will result in a disruption of muscle tone. [7] [1]
Lower motor neurons also play a role in the somatic reflex arc. When muscle spindles detect a sudden stretch, a signal travels down the afferent nerve fibers. These nerve fibers synapse either directly onto the alpha motor neuron (monosynaptic reflex arc), or onto interneurons, which then synapse onto the alpha motor neuron (polysynaptic reflex arc). The lower motor neuron innervates the effector muscle, allowing for a quick muscle response. A reflex arc allows for interpretation of and reaction on the stimulus immediately through the spinal cord, bypassing the brain, resulting in a faster effector response. [1] [8]
Branchial motor neurons innervate the muscles of the head and neck that derive from the branchial arches. They are in the brainstem. The branchial motor neurons and sensory neurons together form the nuclei of cranial nerves V, VII, IX, X, and XI. [1]
Visceral motor neurons contribute to both the sympathetic and parasympathetic functions of the autonomic nervous system. In the sympathetic nervous system, central motor neurons are present in the spinal cord from T1-L2. They appear in the intermediolateral (IML) nucleus. Motor neurons from this nucleus have three different pathways. The first two pathways are to the prevertebral and paravertebral ganglia, from which peripheral neurons go on to innervate the heart, colon, intestines, kidneys, and lungs. The third possible pathway in this system is to the catecholamine-producing chromaffin cells of the adrenal medulla. By targeting these three pathways, the visceral motor neurons in the sympathetic division contribute to the “fight-or-flight” response. On the other hand, in the parasympathetic system, the visceral motor neurons help give rise to cranial nerves III, VII, IX, and X. Besides in the brainstem, these visceral motor neurons contribute to the parasympathetic system in the spinal cord at levels S2-S4. Similarly to the sympathetic division, these motor neurons directly innervate ganglia in the heart, pancreas, lungs, and kidneys. Thus, in both divisions of the autonomic system, these lower motor neurons take on a different role than somatic motor neurons in that they do not directly innervate an effector muscle, and instead innervate ganglia. [1]
- Blood Supply and Lymphatics
The primary motor cortex is supplied primarily by the middle cerebral artery (MCA), along with the anterior cerebral artery (ACA). The MCA supplies the area of the primary motor cortex that is responsible for the upper limbs and face, while the ACA supplies blood to the area that controls the lower limbs. As previously discussed, the upper motor neurons continue down as the pyramidal tract, which receives vascular supply from the lenticulostriate arteries. Once this tract reaches the brainstem, the paramedian branches of the basilar artery become the primary source of blood. At the caudal medulla level, the anterior spinal artery supplies most of the blood. This artery continues to provide blood to the lateral and anterior corticospinal tracts and anterior horn cells in the spinal cord. [9]
- Surgical Considerations
Because there are numerous causes of upper and lower motor neuron dysfunction and injury, surgical consideration requires individualization for each patient. The overall goal of any form of treatment, surgical or not, should be focused on reducing pain and preserving or enhancing day-to-day functionality. [10]
Surgical intervention may also help prevent upper extremity deformity due to contractures or spasticity that may present with an upper motor neuron injury. Examples of surgical procedures that are options for a patient with severe upper motor neuron disease include tendon lengthening, muscle origin release, myotomy, tenotomy, neurectomy, arthrodesis, and joint osteocapsular release. Surgical interventions are chosen based on an individual patient’s level of functioning. [10]
- Clinical Significance
Upper and lower motor neuron lesions cause very different clinical findings. An upper motor neuron lesion is a lesion anywhere from the cortex to the corticospinal tract. This lesion causes hyperreflexia, spasticity, and a positive Babinski reflex, presenting as an upward response of the big toe when the plantar surface of the foot is stroked, with other toes fanning out. On the other hand, lower motor neuron lesions are lesions anywhere from the anterior horn of the spinal cord, peripheral nerve, neuromuscular junction, or muscle. This type of lesion causes hyporeflexia, flaccid paralysis, and atrophy.
Knowledge of the anatomy of the motor neurons is critical to the ability to localize the lesion when faced with a patient who presents with a weakness that is likely due to a motor neuron injury. Focusing mainly on the lateral corticospinal tract, it is essential to keep in mind that this neuronal pathway decussates at the level of the pyramids in the medulla. This crossing means that an upper motor neuron lesion above the medulla will cause symptoms on the contralateral side of the body. However, a lesion to the lateral corticospinal tract after it decussates will present on the ipsilateral side of the body.
Upper motor neuron syndrome occurs when there is injury anywhere to the descending tract before the anterior horn of the spinal cord (cortex, internal capsule, pyramidal tract, lateral corticospinal tract). Examples of pathology that cause upper motor neuron symptoms are strokes, traumatic brain injury, spinal cord injury, amyotrophic lateral sclerosis (ALS), primary lateral sclerosis (PLS), multiple sclerosis (MS), or anoxic brain injury. There are both positive and negative features of the upper motor neuron syndrome. Positive features include hyperreflexia (abnormally brisk reflexes), spasticity (a brisk stretch of muscles causes a sudden increase in tone followed by decreased muscle resistance), and a positive Babinski reflex. Negative features include impaired motor control, easy fatiguability, weakness, and loss of dexterity. [11] [5] [10]
Lower motor neuron syndrome occurs when there is an injury to the anterior horn cells or the peripheral nerve. Diseases of the neuromuscular junction or muscle itself may mimic a lower motor neuron lesion and are important to consider in the differential diagnosis. Similarly to an upper motor neuron lesion, the patient with a lower motor neuron lesion will present with weakness; however, distinct lower motor neuron lesion findings will include hyporeflexia, flaccid paralysis, fasciculations, and atrophy.
There are many forms of motor neuron disease, the most common of which is amyotrophic lateral sclerosis (ALS). This disease is unique in that it presents with both upper and motor neuron signs. The patient will typically present with weakness, along with spastic paralysis and hyperreflexia in the lower limbs and flaccid paralysis and hyporeflexia in the upper limbs. The patient may also present with fasciculations in both the tongue and extremities. Of note, there is no sensory loss. ALS is a progressive neurogenerative disease, and eventually, the patient will have serious dysarthria, dysphagia, extreme weakness, and dyspnea. The estimated median survival is 2 to 4 years, with the most common cause of death being respiratory failure. [12]
One group of genetic disorders that causes lower motor neuron disease is spinal muscular atrophy (SMA). There are many different forms of SMA, but all of them are characterized by degeneration of the motor nuclei in the brainstem, in addition to the anterior horn cells found in the spinal cord. One specific type of SMA is spinobulbar muscular atrophy (Kennedy disease). This x-linked disease usually presents in adulthood (age 30 to 50). First presenting signs typically include tremor, lower extremity weakness, and orolingual fasciculations. This pathology is a progressive disease that is later characterized by the above symptoms in addition to atrophy of limb, bulbar, and facial muscles. [13]
Poliomyelitis is another lower motor neuron disease. This disease results from poliovirus and results in the destruction of the anterior horn cells. Subsequently, the affected patient will experience weakness and lower motor neuron symptoms, including flaccid paralysis in the lower limbs. Usually, this presents asymmetrically. The patient may also provide a history of muscle aches or muscle spasm that occurred in the recent past. Unfortunately, this weakness and paralysis may extend up to involve the respiratory muscles. Many patients will recover some strength, but may later decompensate into “postpolio syndrome,” which is characterized by the onset of additional weakness, pain, and/or atrophy. Among other viral causes of anterior horn cell destruction are coxsackievirus, West Nile virus, and echovirus. [13]
While most cranial nerves are innervated by upper motor neurons bilaterally, cranial nerves VII and XII are the exceptions, as they receive only unilateral input from the contralateral side of the brain. Specifically, damage to the corticobulbar tract and/or facial nerve causes a unique presentation depending on whether the damage occurred in the upper vs. lower motor neuron. The forehead region is dually innervated by corticobulbar tracts from each side of the brain, while the rest of the face below the forehead is innervated primarily by the lower motor neuron of CN VII. An upper motor neuron lesion of the facial nerve can occur anywhere in the corticobulbar tract rostral to the facial motor nucleus on the pons. If an upper motor neuron lesion occurs, the forehead will be spared due to its dual innervation. However, a lower motor neuron lesion of CN VII results in flaccid paralysis of the entire ipsilateral side of the face. [14]
Overall, clinicians should consider motor neuron disease whenever a patient presents with weakness and any of the previously described motor neuron lesion signs without significant sensory loss. Referral to a neurologist for subsequent testing is warranted in these cases.
- Review Questions
- Access free multiple choice questions on this topic.
- Comment on this article.
Figure showing Labeled Motor Neuron. Contributed by Katherine Humphries
Disclosure: Lindsay Zayia declares no relevant financial relationships with ineligible companies.
Disclosure: Prasanna Tadi declares no relevant financial relationships with ineligible companies.
This book is distributed under the terms of the Creative Commons Attribution-NonCommercial-NoDerivatives 4.0 International (CC BY-NC-ND 4.0) ( http://creativecommons.org/licenses/by-nc-nd/4.0/ ), which permits others to distribute the work, provided that the article is not altered or used commercially. You are not required to obtain permission to distribute this article, provided that you credit the author and journal.
- Cite this Page Zayia LC, Tadi P. Neuroanatomy, Motor Neuron. [Updated 2023 Jul 24]. In: StatPearls [Internet]. Treasure Island (FL): StatPearls Publishing; 2024 Jan-.
In this Page
Bulk download.
- Bulk download StatPearls data from FTP
Related information
- PMC PubMed Central citations
- PubMed Links to PubMed
Similar articles in PubMed
- Neuroanatomy, Lower Motor Neuron Lesion. [StatPearls. 2024] Neuroanatomy, Lower Motor Neuron Lesion. Javed K, Daly DT. StatPearls. 2024 Jan
- Spinal premotor interneurons controlling antagonistic muscles are spatially intermingled. [Elife. 2022] Spinal premotor interneurons controlling antagonistic muscles are spatially intermingled. Ronzano R, Skarlatou S, Barriga BK, Bannatyne BA, Bhumbra GS, Foster JD, Moore JD, Lancelin C, Pocratsky AM, Özyurt MG, et al. Elife. 2022 Dec 13; 11. Epub 2022 Dec 13.
- Neuroanatomy, Extrapyramidal System. [StatPearls. 2024] Neuroanatomy, Extrapyramidal System. Lee J, Muzio MR. StatPearls. 2024 Jan
- Review Upper and lower motor neuron neurophysiology and motor control. [Handb Clin Neurol. 2023] Review Upper and lower motor neuron neurophysiology and motor control. de Carvalho M, Swash M. Handb Clin Neurol. 2023; 195:17-29.
- Review Applications of Proteomics to Nerve Regeneration Research. [Neuroproteomics. 2010] Review Applications of Proteomics to Nerve Regeneration Research. Massing MW, Robinson GA, Marx CE, Alzate O, Madison RD. Neuroproteomics. 2010
Recent Activity
- Neuroanatomy, Motor Neuron - StatPearls Neuroanatomy, Motor Neuron - StatPearls
Your browsing activity is empty.
Activity recording is turned off.
Turn recording back on
Connect with NLM
National Library of Medicine 8600 Rockville Pike Bethesda, MD 20894
Web Policies FOIA HHS Vulnerability Disclosure
Help Accessibility Careers
Electromyography (EMG) and Nerve Conduction Studies
- What are EMG and nerve conduction studies? |
- Why would I need an EMG or nerve conduction study? |
- What happens during an EMG or nerve conduction study? |
- What are the problems with an EMG or nerve conduction study? |
Your brain tells your muscles what to do by sending electrical signals to them. The signals travel down your spinal cord and then through different nerves to your muscles.
What are EMG and nerve conduction studies?
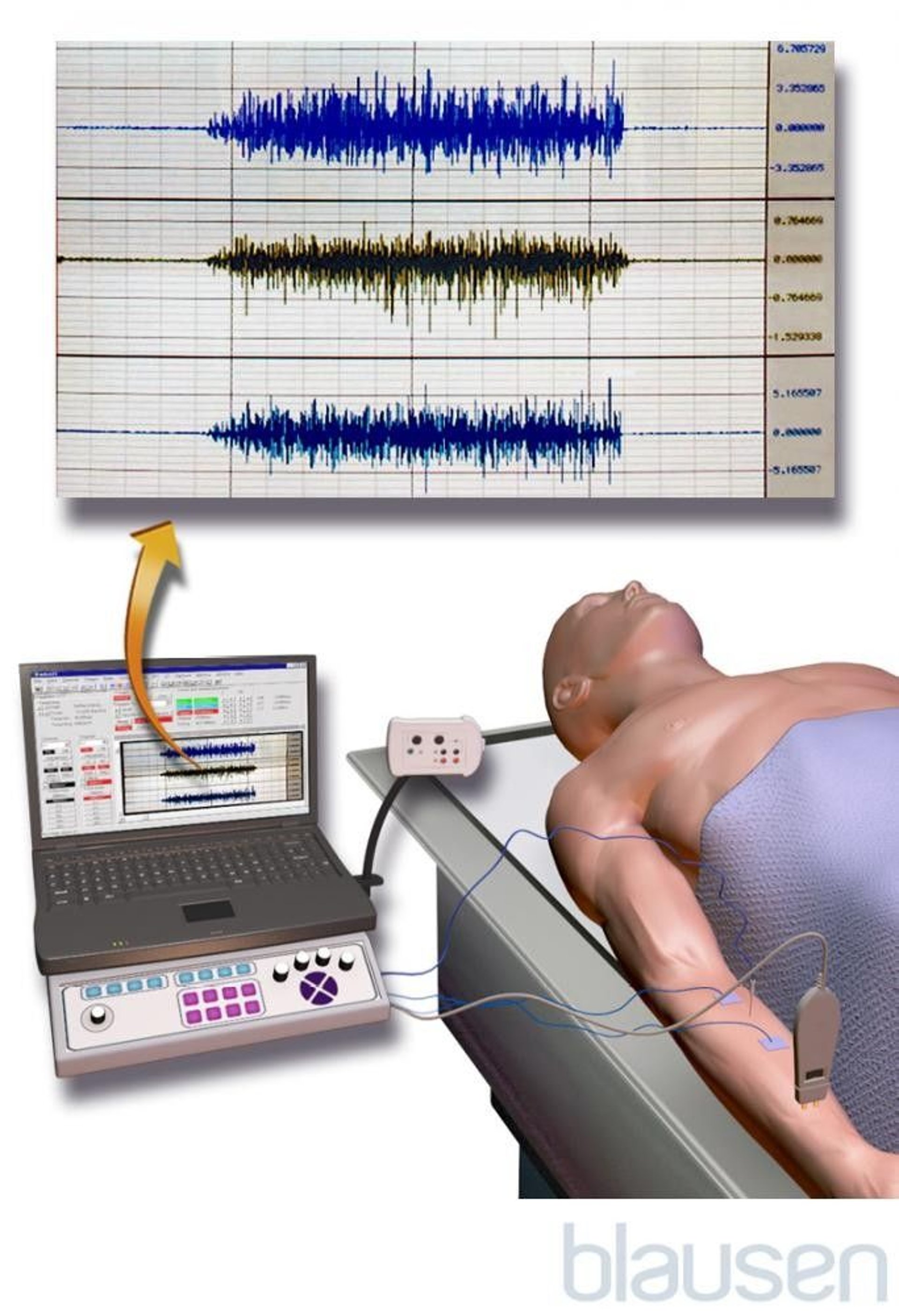
EMG and nerve conduction studies are tests to see if you have muscle weakness or loss of feeling from an injury to your spinal cord, muscles, or nerves.
To do an EMG, doctors insert small needles into a muscle to record your muscle’s electrical activity when it’s resting and when it’s active
To do nerve conduction studies, doctors use skin sensors or needles to send small electric shocks through different nerves to see how well your nerves work
Why would I need an EMG or nerve conduction study?
Doctors may do an EMG, nerve conduction study, or both if you have symptoms like tingling or muscle weakness. These symptoms can be caused by many problems in different parts of your body. An EMG or nerve conduction study can help your doctor tell if your symptoms are caused by nerve or muscle problems such as:
Muscular dystrophy
Carpal tunnel syndrome
Amyotrophic lateral sclerosis (ALS)
What happens during an EMG or nerve conduction study?
During an EMG:
Doctors put needles into your muscle
The needles are connected by wires to a machine that records your muscle's electrical activity while you move it and relax it
During a nerve conduction study:
Doctors put a sticky sensor on your skin over the nerve they are testing
They stimulate another part of the nerve with a small electric shock
A machine measures how fast the electrical signal travels down the nerve
What are the problems with an EMG or nerve conduction study?
The needles and electric shocks can be uncomfortable or hurt. You may have some bruises afterward.

- Cookie Preferences

Copyright © 2024 Merck & Co., Inc., Rahway, NJ, USA and its affiliates. All rights reserved.
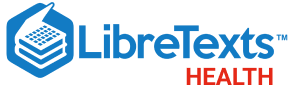
- school Campus Bookshelves
- menu_book Bookshelves
- perm_media Learning Objects
- login Login
- how_to_reg Request Instructor Account
- hub Instructor Commons
- Download Page (PDF)
- Download Full Book (PDF)
- Periodic Table
- Physics Constants
- Scientific Calculator
- Reference & Cite
- Tools expand_more
- Readability
selected template will load here
This action is not available.
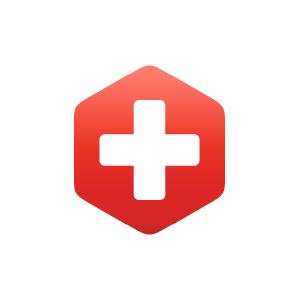
12.3: The Function of Nervous Tissue
- Last updated
- Save as PDF
- Page ID 703
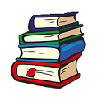
Learning Objectives
- Distinguish the major functions of the nervous system: sensation, integration, and response
- List the sequence of events in a simple sensory receptor–motor response pathway
Having looked at the components of nervous tissue, and the basic anatomy of the nervous system, next comes an understanding of how nervous tissue is capable of communicating within the nervous system. Before getting to the nuts and bolts of how this works, an illustration of how the components come together will be helpful. An example is summarized in Figure \(\PageIndex{1}\).
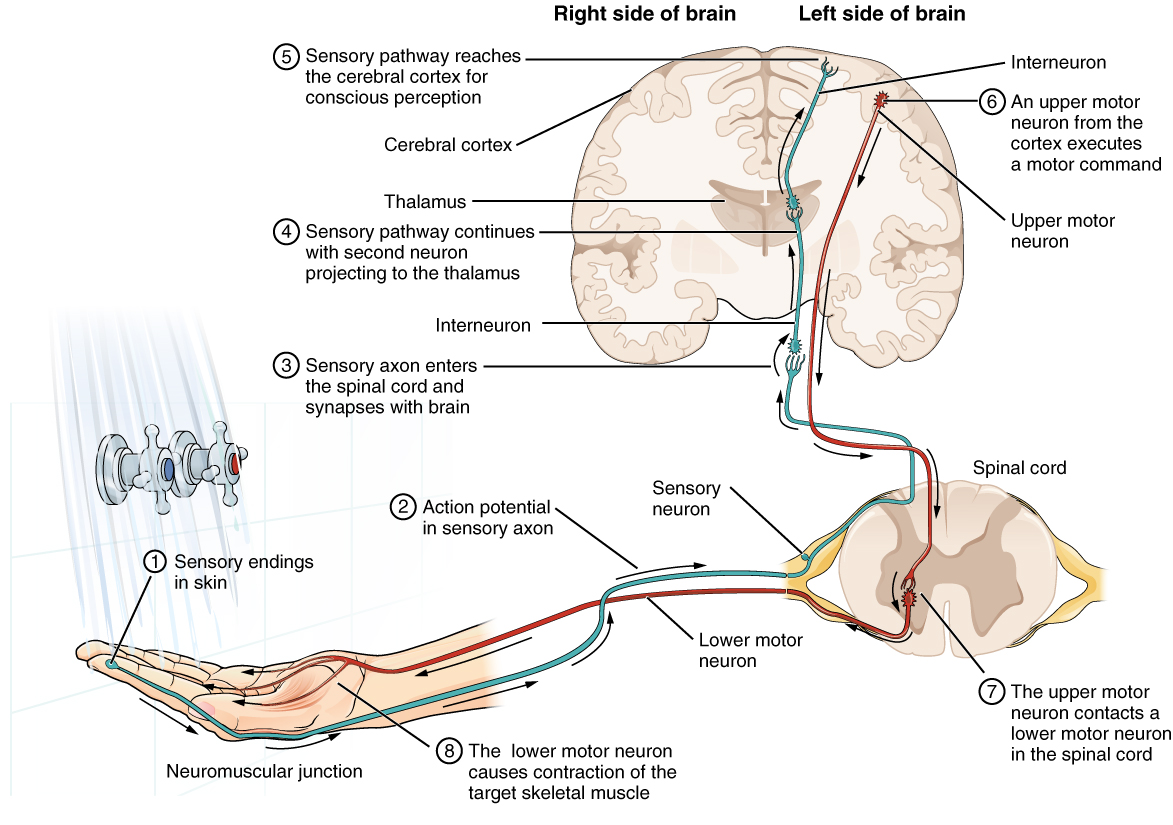
Fi gure 12.3.1: Testing the Water. (1) The sensory neuron has endings in the skin that sense a stimulus such as water temperature. The strength of the signal that starts here is dependent on the strength of the stimulus. (2) The graded potential from the sensory endings, if strong enough, will initiate an action potential at the initial segment of the axon (which is immediately adjacent to the sensory endings in the skin). (3) The axon of the peripheral sensory neuron enters the spinal cord and contacts another neuron in the gray matter. The contact is a synapse where another graded potential is caused by the release of a chemical signal from the axon terminals. (4) An action potential is initiated at the initial segment of this neuron and travels up the sensory pathway to a region of the brain called the thalamus. Another synapse passes the information along to the next neuron. (5) The sensory pathway ends when the signal reaches the cerebral cortex. (6) After integration with neurons in other parts of the cerebral cortex, a motor command is sent from the precentral gyrus of the frontal cortex. (7) The upper motor neuron sends an action potential down to the spinal cord. The target of the upper motor neuron is the dendrites of the lower motor neuron in the gray matter of the spinal cord. (8) The axon of the lower motor neuron emerges from the spinal cord in a nerve and connects to a muscle through a neuromuscular junction to cause contraction of the target muscle.
Imagine you are about to take a shower in the morning before going to school. You have turned on the faucet to start the water as you prepare to get in the shower. After a few minutes, you expect the water to be a temperature that will be comfortable to enter. So you put your hand out into the spray of water. What happens next depends on how your nervous system interacts with the stimulus of the water temperature and what you do in response to that stimulus.
Found in the skin of your fingers or toes is a type of sensory receptor that is sensitive to temperature, called a thermoreceptor . When you place your hand under the shower (Figure \(\PageIndex{2}\)), the cell membrane of the thermoreceptors changes its electrical state (voltage). The amount of change is dependent on the strength of the stimulus (how hot the water is). This is called a graded potential . If the stimulus is strong, the voltage of the cell membrane will change enough to generate an electrical signal that will travel down the axon. You have learned about this type of signaling before, with respect to the interaction of nerves and muscles at the neuromuscular junction. The voltage at which such a signal is generated is called the threshold , and the resulting electrical signal is called an action potential . In this example, the action potential travels—a process known as propagation —along the axon from the axon hillock to the axon terminals and into the synaptic end bulbs. When this signal reaches the end bulbs, it causes the release of a signaling molecule called a neurotransmitter .
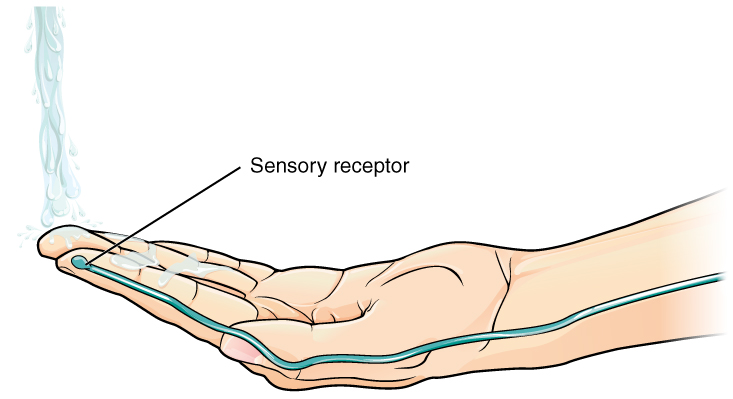
The neurotransmitter diffuses across the short distance of the synapse and binds to a receptor protein of the target neuron. When the molecular signal binds to the receptor, the cell membrane of the target neuron changes its electrical state and a new graded potential begins. If that graded potential is strong enough to reach threshold, the second neuron generates an action potential at its axon hillock. The target of this neuron is another neuron in the thalamus of the brain, the part of the CNS that acts as a relay for sensory information. At another synapse, neurotransmitter is released and binds to its receptor. The thalamus then sends the sensory information to the cerebral cortex , the outermost layer of gray matter in the brain, where conscious perception of that water temperature begins.
Within the cerebral cortex, information is processed among many neurons, integrating the stimulus of the water temperature with other sensory stimuli, with your emotional state (you just aren't ready to wake up; the bed is calling to you), memories (perhaps of the lab notes you have to study before a quiz). Finally, a plan is developed about what to do, whether that is to turn the temperature up, turn the whole shower off and go back to bed, or step into the shower. To do any of these things, the cerebral cortex has to send a command out to your body to move muscles (Figure \(\PageIndex{3}\)).

Figure \(\PageIndex{3}\): The Motor Response. On the basis of the sensory input and the integration in the CNS, a motor response is formulated and executed.
A region of the cortex is specialized for sending signals down to the spinal cord for movement. The upper motor neuron is in this region, called the precentral gyrus of the frontal cortex , which has an axon that extends all the way down the spinal cord. At the level of the spinal cord at which this axon makes a synapse, a graded potential occurs in the cell membrane of a lower motor neuron . This second motor neuron is responsible for causing muscle fibers to contract. In the manner described in the chapter on muscle tissue, an action potential travels along the motor neuron axon into the periphery. The axon terminates on muscle fibers at the neuromuscular junction. Acetylcholine is released at this specialized synapse, which causes the muscle action potential to begin, following a large potential known as an end plate potential. When the lower motor neuron excites the muscle fiber, it contracts. All of this occurs in a fraction of a second, but this story is the basis of how the nervous system functions.
CAREER CONNECTIONS: Neurophysiologist
Understanding how the nervous system works could be a driving force in your career. Studying neurophysiology is a very rewarding path to follow. It means that there is a lot of work to do, but the rewards are worth the effort.
The career path of a research scientist can be straightforward: college, graduate school, postdoctoral research, academic research position at a university. A Bachelor’s degree in science will get you started, and for neurophysiology that might be in biology, psychology, computer science, engineering, or neuroscience. But the real specialization comes in graduate school. There are many different programs out there to study the nervous system, not just neuroscience itself. Most graduate programs are doctoral, meaning that a Master’s degree is not part of the work. These are usually considered five-year programs, with the first two years dedicated to course work and finding a research mentor, and the last three years dedicated to finding a research topic and pursuing that with a near single-mindedness. The research will usually result in a few publications in scientific journals, which will make up the bulk of a doctoral dissertation. After graduating with a Ph.D., researchers will go on to find specialized work called a postdoctoral fellowship within established labs. In this position, a researcher starts to establish their own research career with the hopes of finding an academic position at a research university.
Other options are available if you are interested in how the nervous system works. Especially for neurophysiology, a medical degree might be more suitable so you can learn about the clinical applications of neurophysiology and possibly work with human subjects. An academic career is not a necessity. Biotechnology firms are eager to find motivated scientists ready to tackle the tough questions about how the nervous system works so that therapeutic chemicals can be tested on some of the most challenging disorders such as Alzheimer’s disease or Parkinson’s disease, or spinal cord injury.
Others with a medical degree and a specialization in neuroscience go on to work directly with patients, diagnosing and treating mental disorders. You can do this as a psychiatrist, a neuropsychologist, a neuroscience nurse, or a neurodiagnostic technician, among other possible career paths.
Chapter Review
Sensation starts with the activation of a sensory ending, such as the thermoreceptor in the skin sensing the temperature of the water. The sensory endings in the skin initiate an electrical signal that travels along the sensory axon within a nerve into the spinal cord, where it synapses with a neuron in the gray matter of the spinal cord. The temperature information represented in that electrical signal is passed to the next neuron by a chemical signal that diffuses across the small gap of the synapse and initiates a new electrical signal in the target cell. That signal travels through the sensory pathway to the brain, passing through the thalamus, where conscious perception of the water temperature is made possible by the cerebral cortex. Following integration of that information with other cognitive processes and sensory information, the brain sends a command back down to the spinal cord to initiate a motor response by controlling a skeletal muscle. The motor pathway is composed of two cells, the upper motor neuron and the lower motor neuron. The upper motor neuron has its cell body in the cerebral cortex and synapses on a cell in the gray matter of the spinal cord. The lower motor neuron is that cell in the gray matter of the spinal cord and its axon extends into the periphery where it synapses with a skeletal muscle in a neuromuscular junction.
Review Questions
Q. If a thermoreceptor is sensitive to temperature sensations, what would a chemoreceptor be sensitive to?
C. molecules
D. vibration
Q. Which of these locations is where the greatest level of integration is taking place in the example of testing the temperature of the shower?
A. skeletal muscle
B. spinal cord
C. thalamus
D. cerebral cortex
Q. How long does all the signaling through the sensory pathway, within the central nervous system, and through the motor command pathway take?
A. 1 to 2 minutes
B. 1 to 2 seconds
C. fraction of a second
D. varies with graded potential
Q. What is the target of an upper motor neuron?
A. cerebral cortex
B. lower motor neuron
C. skeletal muscle
D. thalamus
Critical Thinking Questions
Q. Sensory fibers, or pathways, are referred to as “afferent.” Motor fibers, or pathways, are referred to as “efferent.” What can you infer about the meaning of these two terms (afferent and efferent) in a structural or anatomical context?
A. Afferent means “toward,” as in sensory information traveling from the periphery into the CNS. Efferent means “away from,” as in motor commands that travel from the brain down the spinal cord and out into the periphery.
Q. If a person has a motor disorder and cannot move their arm voluntarily, but their muscles have tone, which motor neuron—upper or lower—is probably affected? Explain why.
A. The upper motor neuron would be affected because it is carrying the command from the brain down.

Advertisement
How does the spine form?
- Share Content on Facebook
- Share Content on LinkedIn
- Share Content on Flipboard
- Share Content on Reddit
- Share Content via Email
In a way, your spine is the keystone that holds your body together. It's at the center of your axial skeleton -- the flexible column that holds up the center of your body. At the top of your axial skeleton is your skull, and your coccyx, or tailbone, is at the other end. Together, all these bones shelter some of the most important organs in your body. Your brain sits in its protective skull casing, and the organs in your chest are protected by 12 pairs of ribs, all of which attach directly to your spine.
As if that wasn't enough, your spine is also what lets you move your arms and legs. Your arms attach to your spine and ribs via the collarbones and shoulder blades. Your legs attach to the spine through your hips. When you want to move your arms and legs, signals travel down your spinal cord, which is enclosed in your spine. Nerves carry the signals from the spinal cord to the muscles you want to move.
Arms and legs aren't the only parts of your body that move around, though -- your axial skeleton is also flexible. Discs provide lubrication between each of the 26 bones in your spine. As long as your discs are healthy, your vertebrae don't grind together when you bend and twist. Muscles attach to protrusions on the vertebrae called processes . When the muscles contract, they pull on these leverlike surfaces, and your vertebrae move.
Since your spine has a lot of responsibility, it's not surprising that it has to develop in exactly the right way in order for the whole system to work. So where does it come from, and what can go wrong as it grows?
Spinal Development
If you've read How Pregnancy Works , you already know about how a baby develops in a woman's body. A man's sperm joins with a woman's egg, creating a one-celled zygote . That one cell divides into two, which divide into four, over and over until there are about 100 cells. At this point, a few days after conception, the zygote becomes a blastocyst , and that's when the earliest beginnings of spinal development start to happen.
A blastocyst starts off as a collection of similar cells, but it doesn't stay that way for long. It develops three cell layers, called germ layers , in a process called gastrulation. The layers are the ectoderm, mesoderm and endoderm. Most of the body's innermost organs are formed from the endoderm, while most of the external features, like skin and hair, come from the ectoderm. The spine, part of the middle of the body, comes from the mesoderm. These layers are distinct within 12 days of the egg's fertilization.
The road from undifferentiated cells to a whole body is complex, so here's a rundown of what happens just in terms of the spine:
- The layers of the blastocyst move to where they're needed, arranging themselves to build a body.
- Cells from the mesoderm get together to form a structure called the notochord . This structure provides some support for the developing embryo.
- About 25 days after fertilization, the ectoderm above the notochord folds. The folds form a canal called the neural tube, which will become the central nervous system.
- Mesenchymal cells from the mesoderm form groups called somites on either side of the neural tube. These are like tiny building blocks that will become the vertebrae. Since an embryo develops a tail that disappears as it grows, there are more somites than vertebrae.
- When the embryo is 6 or 7 weeks old, ossification , or bone formation, begins. The somites harden, eventually becoming vertebrae. How Bones Work explains exactly what's happening during ossification. The notochord becomes part of the discs that lubricate the connections between the vertebrae.
In order for the spine to grow correctly, everything has to happen without a hitch, from migration of the blastocyst's layers to ossification. If the spinal column doesn't close correctly, the result can be one of a number of birth defects. Among the most common are neural tube defects, which include spina bifida and anencephaly, or a lack of brain development. Getting enough folate and folic acid during the earliest days of pregnancy reduces the risk of these defects.
You can learn more about the human body and related topics by following the links on the next page.
A fetus's spine looks much different from an adult's spine. While in the womb, a fetus's spine has one C-shaped curve. Once the baby is born and starts walking, the spine settles into four curves that hold the body up while distributing weight.
Lots More Information
Related howstuffworks articles.
- How can scientists use an inkjet printer to make bones?
- Can people get bone marrow transplants from baboons?
- Why is sitting in a chair for long periods bad for your back?
- How Prenatal Testing Works
- How Ultrasound Works
- How Bones Work
- How FOP Works
- How Osteogenesis Imperfecta Works
- Why do a child's bones heal faster than an adult's?
- How do broken bones heal?
- How Body Farms Work
More Great Links
- Human Embryology
- Spina Bifida Association
- Tortora, Gerald J. and Sandra Reynolds Grabowsi. "Principles of Anatomy and Physiology." Ninth edition. John Wiley & Sons, Inc. New York. 2000.
- Mayo Clinic. "Fetal Development: What happens in the first trimester?" 7/25/2007 (6/9/2009) http://www.mayoclinic.com/health/prenatal-care/PR00112
- Medline Plus. "Neural Tube Defects." (6/9/2009) http://www.nlm.nih.gov/medlineplus/neuraltubedefects.html#cat1
- Merck. "Brain and Spinal Cord Defects." Merck Manuals. (6/9/2009) http://www.merck.com/mmhe/sec23/ch265/ch265h.html
- National Institute on Alcohol Abuse and Alcoholism. "Embryonic Development of the Nervous System." 2/2005. (6/9/2009) http://www.niaaa.nih.gov/Resources/GraphicsGallery/FetalAlcoholSyndrome/Embryonic.htm
- Universities of Fribourg, Lausanne and Bern. "Human Embryology." Embryology.ch. (6/9/2009) http://www.embryology.ch/genericpages/moduleembryoen.html
Please copy/paste the following text to properly cite this HowStuffWorks.com article:
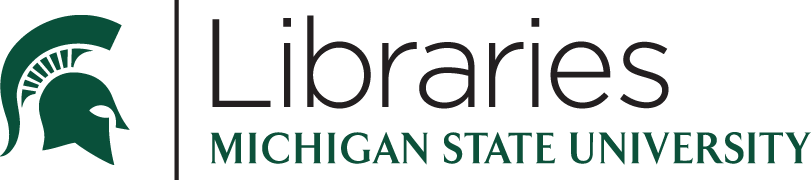
Want to create or adapt books like this? Learn more about how Pressbooks supports open publishing practices.
40 Spinal Motor Control and Proprioception
- Glossary terms
Key Takeaways
- Test Yourself
Additional Review
The motor system refers to the nerve cells that are used to control our body. The key roles of the motor system are to plan, control, and execute voluntary (deliberate) movements, and to control involuntary (subconscious or automatic) functions, such as digesting food. The motor system is sometimes described as a top-down process: in a voluntary movement, neural activity in the frontal lobe sends commands down to motor neurons located in the brainstem or spinal cord, which in turn activate muscle groups. In reality, motor control is more of a loop, rapidly communicating between the sensory cortex and motor cortex. Sensory information about limb position, posture, and objects in contact with the skin inform the descending motor plan. Simultaneously, the motor plan provides predictions about upcoming movement.
There are multiple levels of control. Within the spinal cord, simple reflexes can function without higher input from the brain. Slightly more complex spinal control occurs when central pattern generators function during repetitive movements like walking. The motor and premotor cortices in the brain are responsible for the planning and execution of voluntary movements. And finally, the basal ganglia and cerebellum modulate the responses of the neurons in the motor cortex to help with coordination, motor learning, and balance.
This lesson explores the lowest level of control at the level of the spinal cord.
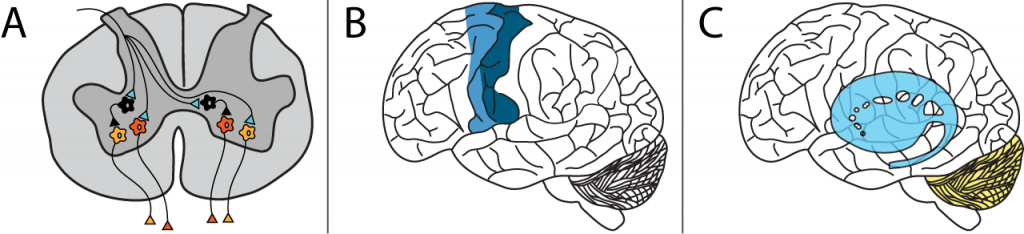
Alpha Motor Neurons
Muscle fibers are innervated by alpha motor neurons . Alpha motor neurons are also called lower motor neurons because they are not located in higher brain areas. These cells are the only cells that directly command muscle contraction. The cell bodies of the alpha motor neurons are located in the central nervous system in the ventral horn of the spinal cord. Their axons leave the spinal cord via the ventral roots and travel to the muscle via efferent peripheral spinal nerves.
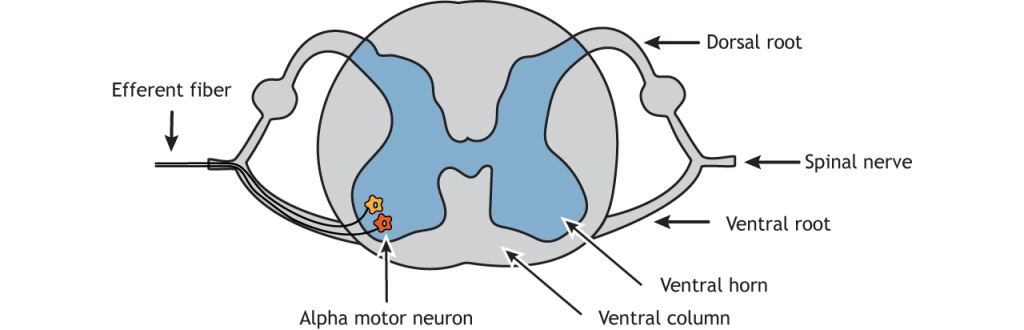
Like the sensory systems, the motor system is also organized in a topographic fashion, referred to as ‘somatotopic organization’. Within the spinal cord, alpha motor neurons that innervate muscles in the arms and legs are located in the lateral portion of the ventral horn, whereas alpha motor neurons that innervate muscles in the trunk are located in the medial portion.
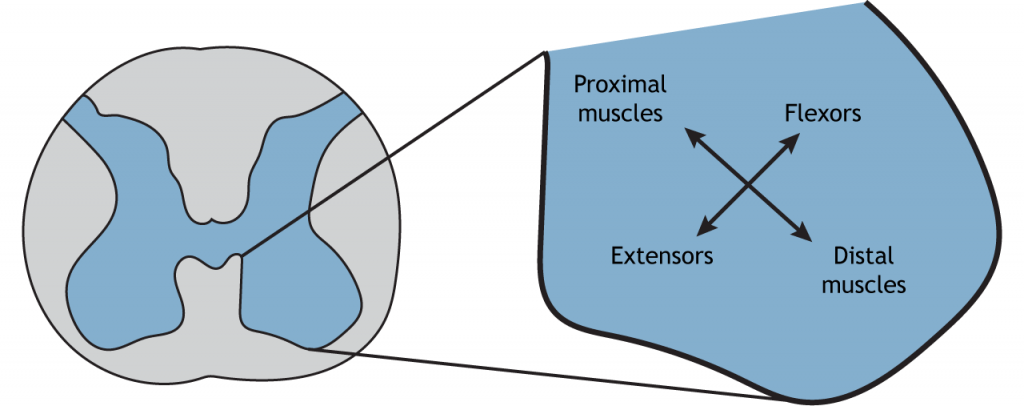
Location of Alpha Motor Neurons
The structure of the spinal cord is reviewed in Chapter 26 . Examining cross sections of the spinal cord at different levels reveals that there is a non-uniform distribution of lower motor neurons. This is determined by the size of the ventral horn at the different levels of the spinal cord. At the cervical enlargement the larger ventral horns are due to the presence of more lower motor neurons that function in movement of the arms. At the lumbar enlargement the larger ventral horns are due to the presence of more lower motor neurons that function in movement of the legs.
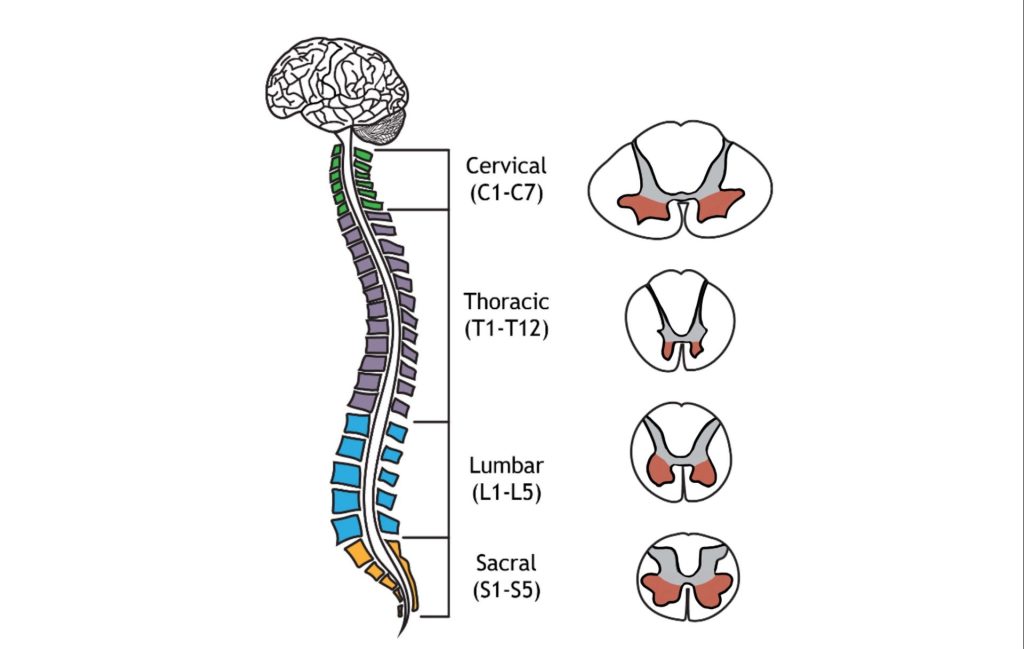
Neuromuscular Junction
The lower motor neurons communicate with muscle fibers (muscle cells) at the neuromuscular junction (NMJ). The NMJ is similar to other chemical synapses, however the postsynaptic cell is a muscle cell separated by about 30 nm. The presynaptic cell is the motor neuron and the postsynaptic site is the sarcolemma, the cell membrane of the long cylindrical muscle fibers (muscle cells). The neuromuscular junction is one of the largest synapses in the body and one of the most well-studied because of its peripheral location.
The neuromuscular junction is located midway down the length of the muscle fiber. The muscle fiber will contract in response to depolarization traveling down the sarcolemma of the muscle fiber. The synapse is located midway down the length of the muscle fiber so that the postsynaptic signal can travel in both directions down the long muscle fiber and quickly activate a contraction along the entire cell.
Acetylcholine is the neurotransmitter released at the neuromuscular junction (NMJ), and it acts upon ligand-gated, non-selective cation channels called nicotinic acetylcholine receptors that are present in postjunctional folds of the muscle fiber. Nicotinic acetylcholine receptors allow the influx of sodium ions into the muscle cell. The depolarization will cause nearby voltage-gated channels to open and fire an action potential in the muscle fiber. In a healthy system, an action potential in the motor neurons always causes an action potential in the muscle cell. The action potential leads to contraction of the muscle fiber.
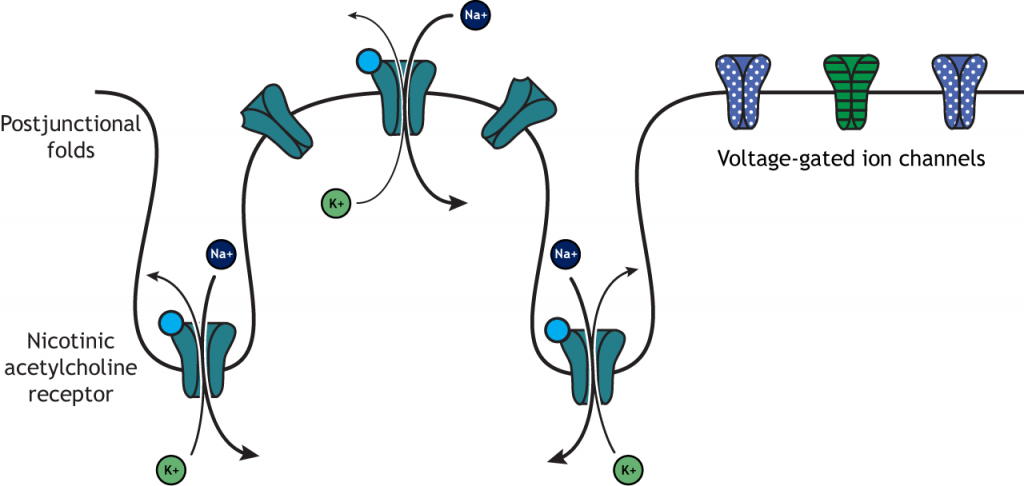
To review, an action potential traveling down the motor neuron (presynaptic cell) will cause the release of acetylcholine into the synapse. Acetylcholine binds to postsynaptic nicotinic acetylcholine receptors that are located in the folded sarcolemma (increasing surface area), causing depolarization of muscle fibers and ultimately muscle contraction.
Acetylcholinesterase , an enzyme that breaks down acetylcholine and terminates its action, is present in the synaptic cleft of the neuromuscular junction. Muscle contraction must be tightly controlled. Thus, the actions of acetylcholinesterase are very important to cease muscle contraction quickly.
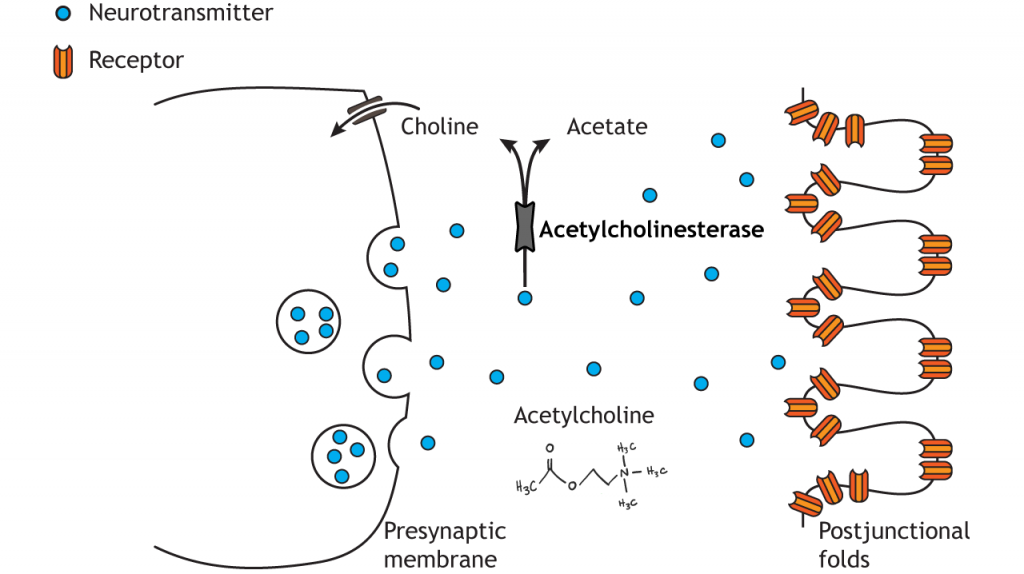
Clinical Application: Myasthenia gravis
Myasthenia gravis (MG) is an autoimmune disorder characterized by muscle weakness, resulting in difficulty with speech, trouble with movement and swallowing, drooping eyelids, and double vision. Each year, an estimated 20 out of a million people get diagnosed with MG.
The muscle weakness seen in MG results from immune system-mediated destruction of the nicotinic acetylcholine receptors expressed at the NMJ. Thus, when the lower motor neuron releases acetylcholine, the muscle cells are unable to detect this release, so they fail to contract appropriately.
One therapeutic strategy involves inhibition of acetylcholinesterase, the enzyme that degrades acetylcholine. This causes the synaptic acetylcholine to remain in the synapse longer, increasing the chance that receptors get activated. Alternatively, autoimmune diseases like MG can be improved with immunosuppressant therapy. With successful treatment, MG usually does not result in changes in lifespan.

Motor Units
Importantly, there are many more muscles cells than there are motor neurons. One alpha motor neuron can innervate multiple fibers within one muscle due to the branching of motor neuron axons. Each axon terminal synapses (innervates) a single muscle fiber. A motor neuron and all the fibers innervated by it are called a motor unit . The muscle fibers within one motor unit are often spread throughout the muscle to spread the contraction throughout the full muscle. Further, motor units in a muscle usually contract asynchronously to help protect the muscle from fatigue. A graded contraction of the muscle is produced by activating varying numbers of motor units.
Motor units differ in size. Small motor units are motor units that innervate fewer muscle fibers and thus control fine movements. Small motor units are located in the eyes and fingers, both of which function in fine and precise movements. Large motor units innervate many muscle fibers and are typically found in weight-bearing muscles like the thighs.
The group of motor neurons that innervate all the fibers of one muscle is called a motor pool .
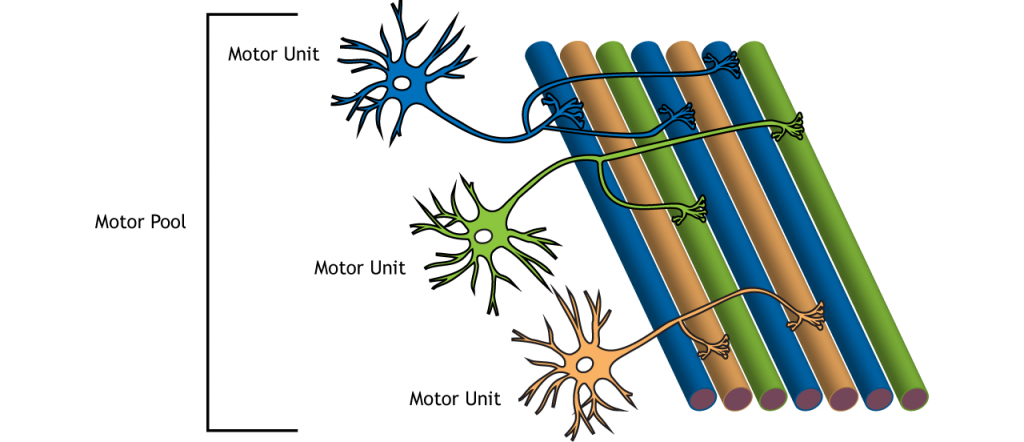
Types of Motor Units
In addition to the size of the motor unit, the types of muscle fibers that are innervated by motor units can also differ. There are three different types of motor units:
- Slow motor units . Slow motor units are slow to contract and generate less force but can work for a long time. They are used in endurance exercise like jogging.
- Fast fatigue-resistant motor units . Fast fatigue-resistant motor units are quick to contract (though not as fast the fast fatigable motor units). These motor units generate more force that then slow motor units, but are much more resistant to fatigue than the fast fatigable motor units.
- Fast fatigable motor units . Fast fatigable motor units are quickest to contract and generate the most force. These motor units are more prone to fatigue due to decreased number of mitochondria within the muscles. Fast fatigable motor units generate a lot of force quickly, but also tire quickly. They are used mostly in high intensity exercise like lifting weights and sprinting.
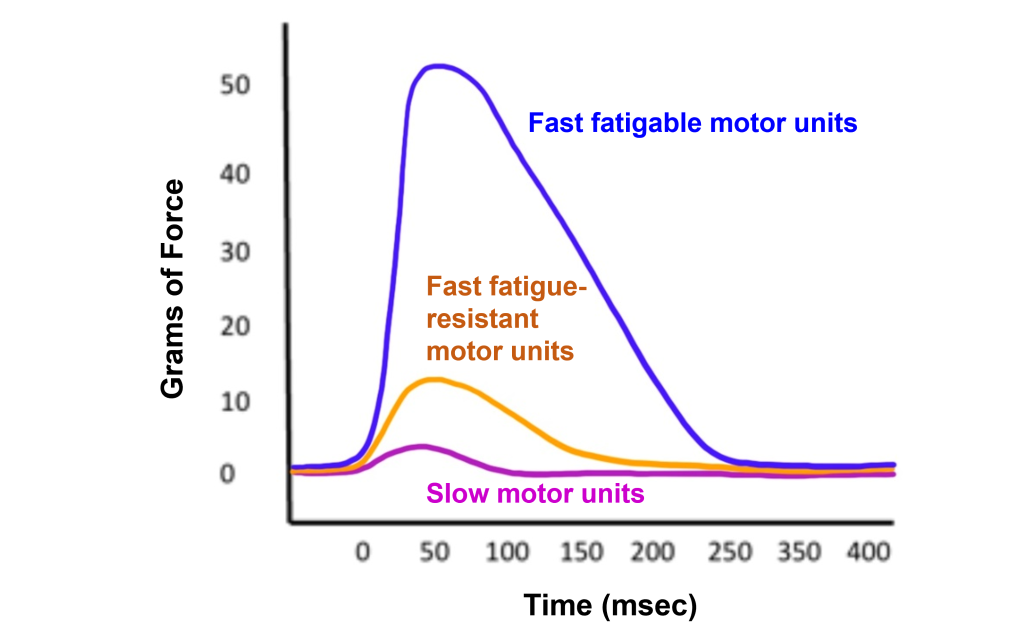
Muscle Activation
Action potential triggered in a muscle.
When an action potential is triggered in a muscle it causes a muscle twitch (contraction) that is followed by a period of relaxation. A muscle twitch shows an increase in tension after a short delay (latent period). During the contraction period, the muscle tension increased, and then has a long relaxation period, causing the muscle tension to be increased long after the initial stimulus.
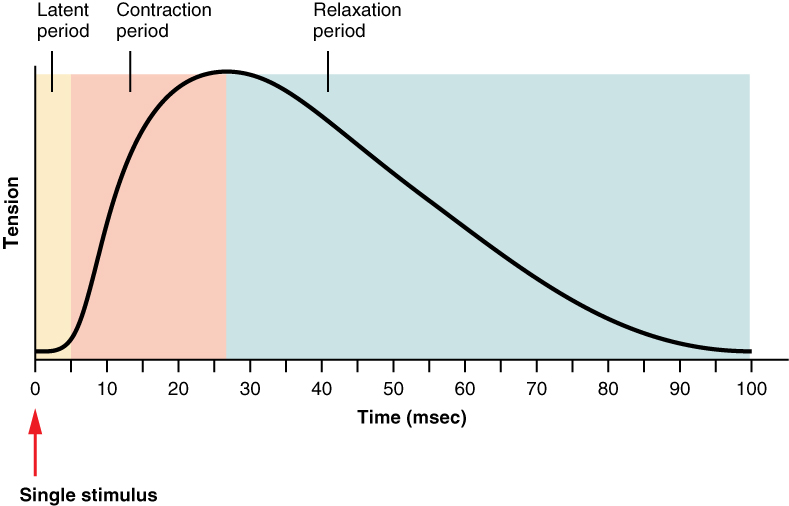
When a stimulus occurs during the long relaxation period following a muscle twitch, the newly generated muscle twitch will summate to increase the strength of the overall muscle contraction. Shortening the time between stimuli will result in unfused tetanus and if stimuli are very close together will result in fused tetanus . Therefore, a higher rate of action potentials in the alpha motor neuron will generate more muscle contraction.
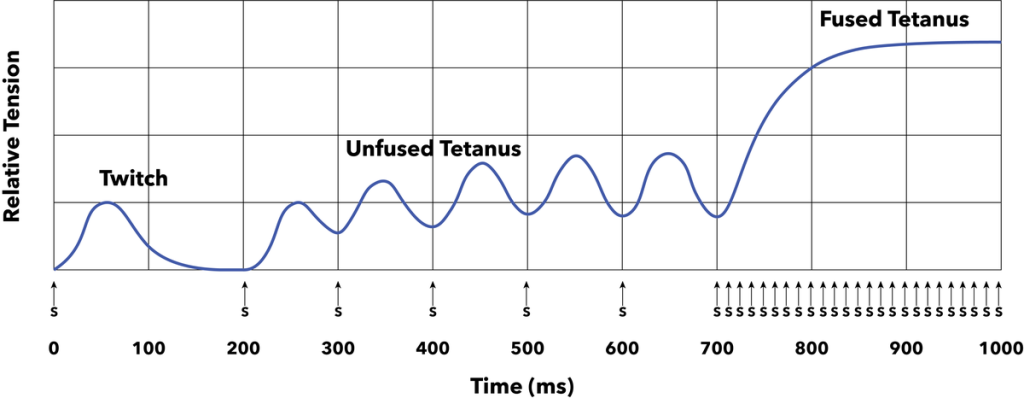
Muscle Recruitment
In addition to the rate of action potentials changing the force of the muscle contraction, muscle recruitment can also increase the strength of contraction within a muscle. When generating motor activity, the smallest motor units will be activated to contract first due to their size and increased excitability to move the load. Increasingly larger motor units are recruited to lift heavier loads. Recruitment of motor units allows for us to generate appropriate muscle tension to move a given load. For example, if you need to pick up a pencil, then we do not need to use the same force as if we were trying to pick up a 20-pound weight. Only smaller motor units would be activated to pick up the pencil, and increasingly larger motor units would be recruited to lift the heavier load of the 20-pound weight.
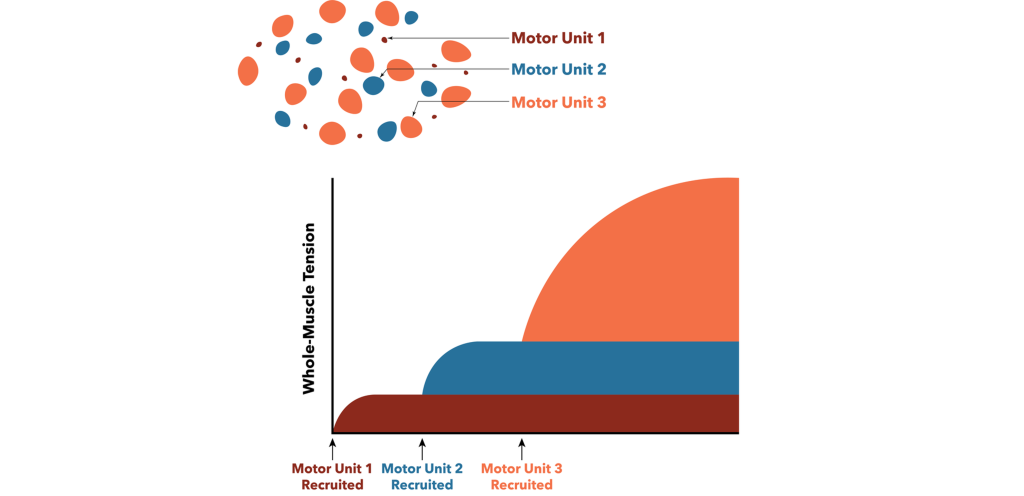
Alpha Motor Neuron Inputs
The alpha motor neurons that directly cause muscle contraction receive inputs from three different sources.
- Sensory cells from the dorsal root ganglion that provide sensory information from the muscles through proprioception.
- Upper motor neurons from the motor cortex in the brain and brain stem that are responsible for initiating voluntary movement.
- Interneurons in the spinal cord. These represent the largest input to the alpha motor neurons and can either provide excitation or inhibition to the alpha motor neuron.
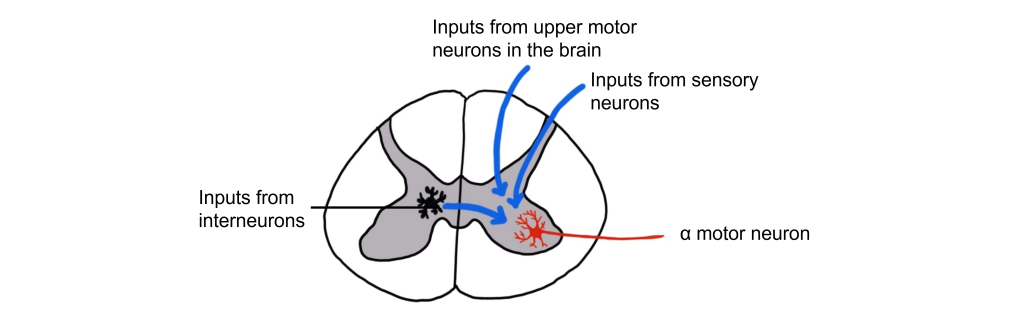
Proprioception
Raise your arms above your head. Even without seeing your arms, your nervous system has mechanisms that inform you about the location and position of your body parts, including how much your joints are bent. This sense is called proprioception and is critically important for coordinated movement and motor reflexes that contribute to those tiny, rapid adjustments that are made while maintaining balance. Proprioceptive information ascends through the spinal cord and into the brain via the dorsal column-medial lemniscus tract . Proprioception is also processed in the primary somatosensory cortex.
Proprioception refers to the “body sense” that informs us about how our bodies are positioned and moving in space. Proprioceptors are receptors that provide proprioception information.
There are two main types of proprioceptors:
- Muscle spindles measure muscle stretch (muscle length) and transmit this sensory information via 1a sensory afferent fibers. Muscle spindles are nested within and arranged parallel to the extrafusal muscle fibers.
- Golgi Tendon Organs measure muscle tension and transmit this sensory information via 1b sensory afferent fibers. Golgi tendon organs are located between the extrafusal muscle fibers and their points of attachment at the bone.
Muscle Spindles
Extrafusal and intrafusal muscle fibers.
Muscle spindles are fibrous capsules that are located within muscles. Intrafusal muscle fibers are special muscle fibers that are located within the fibrous capsule of the muscle spindle. The intrafusal muscle fibers are innervated by gamma motor neurons that will cause them to contract.
Extrafusal muscle fibers , however, make up the bulk of the muscle and are located outside of the muscle spindle. The extrafusal muscle fibers are stimulated to contract by the alpha motor neurons .
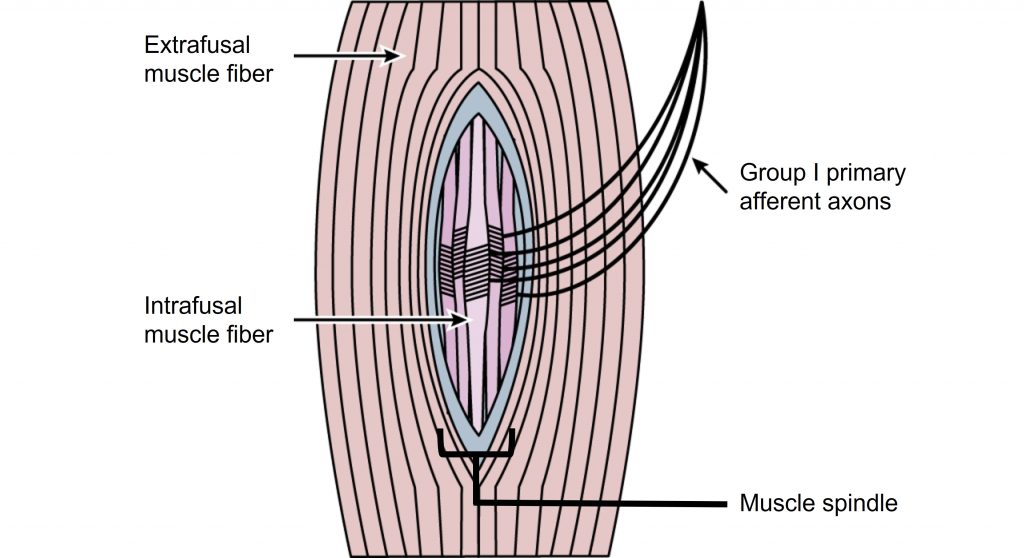
Muscle Spindles Function
Group 1a sensory afferent axons , which have a large diameter and are heavily myelinated, wrap around the intrafusal fibers contained within the muscle spindle. These sensory afferent fibers will signal when the intrafusal fibers of the muscle spindle are experiencing stretch and communicate information about muscle length.
Within the spinal cord, a single sensory 1a afferent axon synapses on every alpha motor neuron within the motor pool that innervates the muscle that contains the muscle spindle. This allows for a fast and powerful contraction of the muscle in response to a change in muscle stretch.
Gamma Motor Neuron Function
When the muscle experiences a stretch and the extrafusal fibers are stretched, the muscle spindle and the intrafusal fibers are also stretched (due to being within the muscle and surrounded by the extrafusal fibers ). When the muscle spindle stretches, the 1a sensory axon will start to fire action potentials. The sensory axon synapses with an alpha motor neuron that will then cause the extrafusal muscle fibers to contract. As the extrafusal fibers contract and the muscle shortens, the muscle spindle goes slack, and the 1a axon will no longer fire action potentials as it is no longer being stretched. The gamma motor neuron is then activated that innervates the intrafusal muscle fibers , causing the intrafusal fibers to contract, allowing the muscle spindle to sense stretch again. Therefore, the gamma motor neuron is critical for allowing the muscle spindle to continue providing information about muscle stretch even when the muscle has experienced contraction.
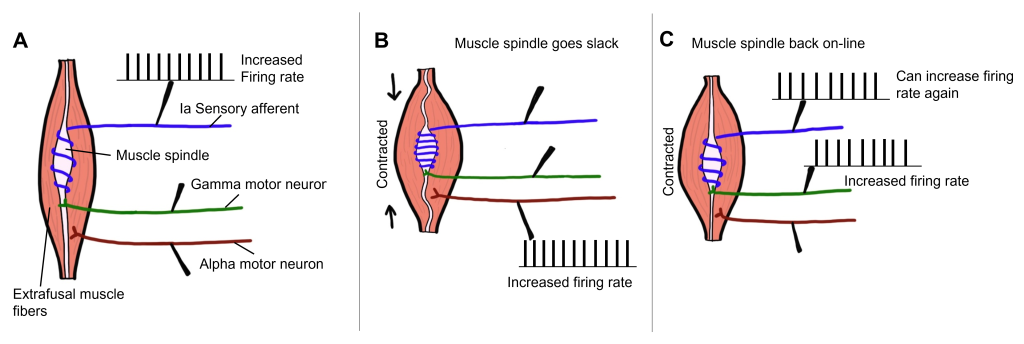
Golgi Tendon Organ
The Golgi tendon organ is a proprioceptor that measures muscle tension, or the force of contraction. They also contribute to our detection of weight, as we lift something heavy for example. Golgi tendon organs are located in the tendon that connects the muscle to bone. The tendon is made up of collagen fibrils and the Group 1b sensory axons are intertwined within the collagen fibrils. When the muscle experiences an increase in tension, the collagen fibrils surrounding the 1b sensory axon physically squeeze the 1b axon, opening mechanically-gated ion channels within the 1b sensory axon.
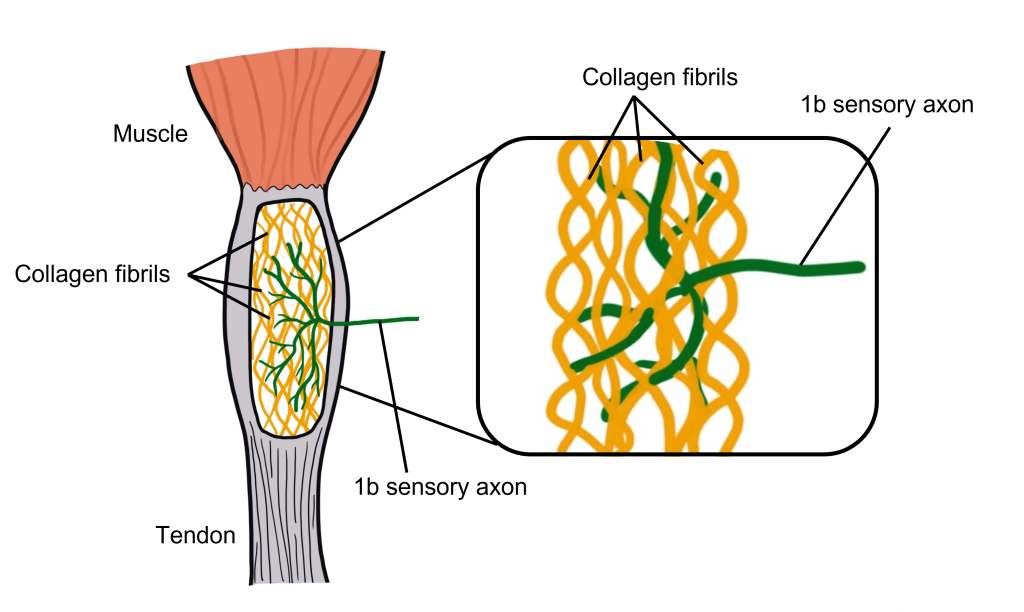
The purpose of the Golgi tendon organ is to allow for an optimal range of tension for the muscle and to protect the muscle from injury due to being overloaded. This is accomplished through a negative feedback loop controlled by the Golgi tendon organ. When the muscle experiences an increase in muscle tension, the 1b sensory axon starts to fire action potentials. The sensory neuron synapses onto an inhibitory interneuron within the spinal cord, which when active will release GABA onto the alpha motor neuron that innervates the same muscle that experienced the increase in muscle tension to begin with. When GABA binds to the alpha motor neuron, it will decrease firing of the alpha motor neuron, leading to a decrease in contraction of the muscle. This is an example of negative feedback as the increased muscle tension ultimately leads to physiological changes that decrease muscle tension.
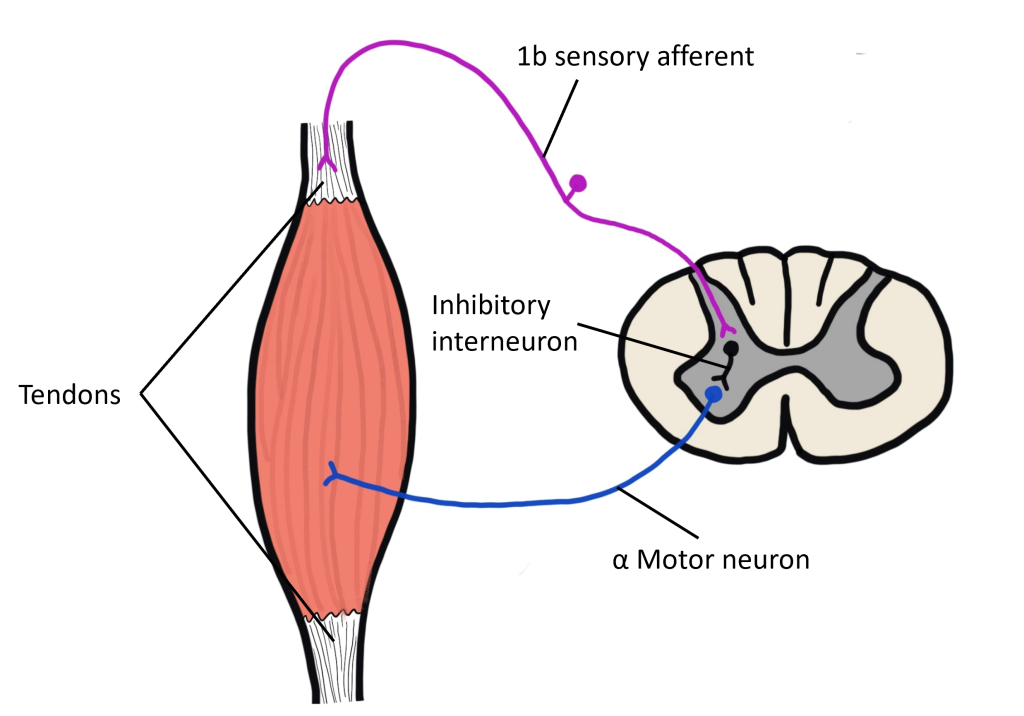
- Motor neuron cell bodies are located in the ventral horn of the spinal cord.
- Motor neuron axons are located in the peripheral nervous system and travel to muscles via spinal nerves.
- Acetylcholine is released at the neuromuscular junction and acts upon ionotropic nicotinic acetylcholine receptors.
- The spinal cord is topographically organized.
- Muscle twitches can summate to increase muscle tension.
- A motor unit is an alpha motor neuron and all of the motor fibers that it innervates. Motor units differ in size, recruitment, and power.
- Muscle spindles and Golgi tendon organs are proprioceptors that communicate information about the location and position of the body.
Test Yourself!
- What is the difference between a motor unit and a motor pool?
Attributions
Portions of this chapter were remixed and revised from the following sources:
- Foundations of Neuroscience by Casey Henley. The original work is licensed under a Creative Commons Attribution-NonCommercial-ShareAlike 4.0 International License
- Open Neuroscience Initiative by Austin Lim. The original work is licensed under a Creative Commons Attribution-NonCommercial 4.0 International License .
Media Attributions
- MotorControlRegions © Casey Henley adapted by Valerie Hedges is licensed under a CC BY-NC-SA (Attribution NonCommercial ShareAlike) license
- Alpha Motor Neuron © Casey Henley adapted by Valerie Hedges is licensed under a CC BY-NC-SA (Attribution NonCommercial ShareAlike) license
- Spinal Cord Map © Casey Henley adapted by Valerie Hedges is licensed under a CC BY-NC-SA (Attribution NonCommercial ShareAlike) license
- Untitled_Artwork 1 © Casey Henley adapted by Valerie Hedges is licensed under a CC BY-NC-SA (Attribution NonCommercial ShareAlike) license
- NMJ Ion Flow © Casey Henley adapted by Valerie Hedges is licensed under a CC BY-NC-SA (Attribution NonCommercial ShareAlike) license
- NeuromuscularJunction © Casey Henley adapted by Valerie Hedges is licensed under a CC BY-NC-SA (Attribution NonCommercial ShareAlike) license
- Myasthenia Gravis © Posey and Spiller adapted by Valerie Hedges is licensed under a Public Domain license
- Motor Unit And Pool © Casey Henley adapted by Valerie Hedges is licensed under a CC BY-NC-SA (Attribution NonCommercial ShareAlike) license
- Types of motor units © Valerie Hedges is licensed under a CC BY-NC-SA (Attribution NonCommercial ShareAlike) license
- Muscle_Twitch_Myogram © OpenStax adapted by Valerie Hedges is licensed under a CC BY (Attribution) license
- Twitch_vs_unfused_tetanus_vs_fused_tetanus © Daniel Walsh and Alan Sved adapted by Valerie Hedges is licensed under a CC BY-SA (Attribution ShareAlike) license
- Motor unit recruitment © Daniel Walsh and Alan Sved is licensed under a CC BY-SA (Attribution ShareAlike) license
- Motor neuron inputs © Valerie Hedges is licensed under a CC BY-NC-SA (Attribution NonCommercial ShareAlike) license
- Muscle Spindle © Casey Henley adapted by Valerie Hedges is licensed under a CC BY-NC-SA (Attribution NonCommercial ShareAlike) license
- Gamma motor neuron function © Valerie Hedges is licensed under a CC BY-NC-SA (Attribution NonCommercial ShareAlike) license
- Golgi tendon organ © Valerie Hedges is licensed under a CC BY-NC-SA (Attribution NonCommercial ShareAlike) license
- golgi tendon organ signaling © Valerie Hedges is licensed under a CC BY-NC-SA (Attribution NonCommercial ShareAlike) license
traveling from the CNS to the body
Toward the edge
Toward the middle
The synapse between a motor neuron and a muscle fiber
a motor neuron and all of the muscle fibers that is innervates
The group of motor neurons that innervate all the fibers of one muscle
Body sense that allows for understanding of location and position of body parts
sensory receptors that provide information about proprioception
proprioceptors that communicate muscle length (muscle stretch)
muscle fibers that are located inside of the muscle spindle capsule
muscle fibers that are outside of the muscle spindle capsule. Extrafusal fibers make up the bulk of the muscle.
Proprioceptor that communicates information about muscle length
Proprioceptor that measures muscle tension
Introduction to Neuroscience Copyright © 2022 by Valerie Hedges is licensed under a Creative Commons Attribution-NonCommercial-ShareAlike 4.0 International License , except where otherwise noted.
Share This Book
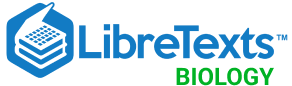
- school Campus Bookshelves
- menu_book Bookshelves
- perm_media Learning Objects
- login Login
- how_to_reg Request Instructor Account
- hub Instructor Commons
- Download Page (PDF)
- Download Full Book (PDF)
- Periodic Table
- Physics Constants
- Scientific Calculator
- Reference & Cite
- Tools expand_more
- Readability
selected template will load here
This action is not available.
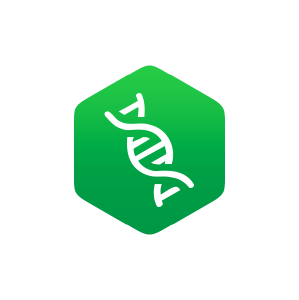
15.8C: The Human Central Nervous System
- Last updated
- Save as PDF
- Page ID 5648
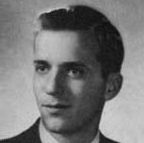
- John W. Kimball
- Tufts University & Harvard
The central nervous system is made up of the spinal cord and brain. The spinal cord conducts sensory information from the peripheral nervous system (both somatic and autonomic) to the brain. It also conducts motor information from the brain to our various effectors: skeletal muscles, cardiac muscle, smooth muscle, glands, and serves as a minor reflex center. The brain receives sensory input from the spinal cord as well as from its own nerves (e.g., olfactory and optic nerves) and devotes most of its volume (and computational power) to processing its various sensory inputs and initiating appropriate and coordinated motor outputs
White Matter vs. Gray Matter
Both the spinal cord and the brain consist of white matter (bundles of axons each coated with a sheath of myelin) and gray matter (masses of the cell bodies and dendrites each covered with synapses). In the spinal cord, the white matter is at the surface, the gray matter inside. In the brain of mammals , this pattern is reversed. However, the brains of "lower" vertebrates like fishes and amphibians have their white matter on the outside of their brain as well as their spinal cord.
The Meninges
Both the spinal cord and brain are covered in three continuous sheets of connective tissue, the meninges. From outside in, these are thedura mater — pressed against the bony surface of the interior of the vertebrae and the cranium, the arachnoid, nd the pia mater. The region between the arachnoid and pia mater is filled with cerebrospinal fluid (CSF).
The Interstitial Fluid of the Central Nervous System
The cells of the central nervous system are bathed in a fluid, called cerebrospinal fluid ( CSF ), that differs from that serving as the interstitial fluid (ISF) of the cells in the rest of the body. Cerebrospinal fluid leaves the capillaries in the choroid plexus of the brain. It contains far less protein than "normal" because of the blood-brain barrier , a system of tight junctions between the endothelial cells of the capillaries. This barrier creates problems in medicine as it prevents many therapeutic drugs from reaching the brain. CSF flows uninterrupted throughout the central nervous system through the central cerebrospinal canal of the spinal cord and through an interconnected system of four ventricles in the brain.
CSF returns to the blood through lymphatic vessels draining the brain.In mice, the flow of CSF increases by 60% when they are asleep. Perhaps one function of sleep is to provide the brain a way of removing potentially toxic metabolites accumulated during waking hours.
The Spinal Cord
31 pairs of spinal nerves arise along the spinal cord. These are "mixed" nerves because each contain both sensory and motor axons. However, within the spinal column, all the sensory axons pass into the dorsal root ganglion where their cell bodies are located and then on into the spinal cord itself. All the motor axons pass into the ventral roots before uniting with the sensory axons to form the mixed nerves.
The spinal cord carries out two main functions:
- It connects a large part of the peripheral nervous system to the brain. Information (nerve impulses) reaching the spinal cord through sensory neurons are transmitted up into the brain. Signals arising in the motor areas of the brain travel back down the cord and leave in the motor neurons.
- The spinal cord also acts as a minor coordinating center responsible for some simple reflexes like the withdrawal reflex.
The interneurons carrying impulses to and from specific receptors and effectors are grouped together in spinal tracts .
Crossing Over of the Spinal Tracts
Impulses reaching the spinal cord from the left side of the body eventually pass over to tracts running up to the right side of the brain and vice versa. In some cases this crossing over occurs as soon as the impulses enter the cord. In other cases, it does not take place until the tracts enter the brain itself.
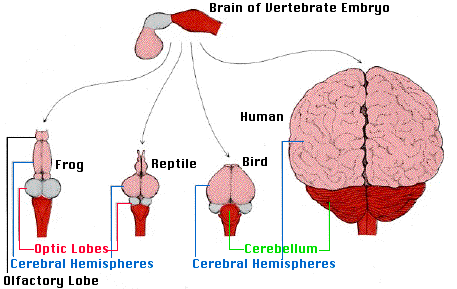
The brain of all vertebrates develops from three swellings at the anterior end of the neural tube of the embryo. From front to back these develop into the
- forebrain (also known as the prosencephalon — shown in light color)
- midbrain (mesencephalon — gray)
- hindbrain (rhombencephalon — dark color) The human brain is shown from behind so that the cerebellum can be seen.
The human brain receives nerve impulses from the spinal cord and 12 pairs of cranial nerves:
- Some of the cranial nerves are "mixed", containing both sensory and motor axons
- Some, e.g., the optic and olfactory nerves (numbers I and II) contain sensory axons only
- Some, e.g. number III that controls eyeball muscles, contain motor axons only.
The Hindbrain
The main structures of the hindbrain (rhombencephalon) are the medulla oblongata, pons and cerebellum.
Medulla oblongata
The medulla looks like a swollen tip to the spinal cord. Nerve impulses arising here rhythmically stimulate the intercostal muscles and diaphragm thus making breathing possible. It also regulate heartbeats and regulate the diameter of arterioles thus adjusting blood flow. The neurons controlling breathing have mu (µ) receptors , the receptors to which opiates , like heroin, bind. This accounts for the suppressive effect of opiates on breathing. Destruction of the medulla causes instant death.
The pons seems to serve as a relay station carrying signals from various parts of the cerebral cortex to the cerebellum. Nerve impulses coming from the eyes, ears and touch receptors are sent on the cerebellum. The pons also participates in the reflexes that regulate breathing.
The reticular formation is a region running through the middle of the hindbrain (and on into the midbrain). It receives sensory input (e.g., sound) from higher in the brain and passes these back up to the thalamus. The reticular formation is involved in sleep, arousal (and vomiting).
The cerebellum consists of two deeply-convoluted hemispheres. Although it represents only 10% of the weight of the brain, it contains as many neurons as all the rest of the brain combined. Its most clearly-understood function is to coordinate body movements. People with damage to their cerebellum are able to perceive the world as before and to contract their muscles, but their motions are jerky and uncoordinated. So the cerebellum appears to be a center for learning motor skills (implicit memory). Laboratory studies have demonstrated both long-term potentiation ( LTP ) and long-term depression ( LTD ) in the cerebellum.
The Midbrain
The midbrain (mesencephalon) occupies only a small region in humans (it is relatively much larger in "lower" vertebrates). We shall look at only three features:
- the reticular formation : collects input from higher brain centers and passes it on to motor neurons.
- the substantia nigra : helps "smooth" out body movements; damage to the substantia nigra causes Parkinson's disease.
- the ventral tegmental area ( VTA ): packed with dopamine -releasing neurons that are activated by nicotinic acetylcholine receptors and whose projections synapse deep within the forebrain.The VTA seems to be involved in pleasure: nicotine, amphetamines and cocaine bind to and activate its dopamine-releasing neurons and this may account at least in part for their addictive qualities.
The midbrain along with the medulla and pons are often referred to as the "brainstem".
The Forebrain
The human forebrain (prosencephalon) is made up of a pair of large cerebral hemispheres , called the telencephalon . Because of crossing over of the spinal tracts, the left hemisphere of the forebrain deals with the right side of the body and vice versa. A group of structures located deep within the cerebrum make up the diencephalon .
Diencephalon
We shall consider four of its structures:
- All sensory input (except for olfaction) passes through these paired structures on the way up to the somatic-sensory regions of the cerebral cortex and then returns to them from there.
- Signals from the cerebellum pass through them on the way to the motor areas of the cerebral cortex.
- Lateral geniculate nucleus ( LGN ). All signals entering the brain from each optic nerve enter a LGN and undergo some processing before moving on the various visual areas of the cerebral cortex.
- The seat of the autonomic nervous system. Damage to the hypothalamus is quickly fatal as the normal homeostasis of body temperature, blood chemistry, etc. goes out of control.
- The source of 8 hormones, two of which pass into the posterior lobe of the pituitary gland.
- Posterior lobe of the pituitary . Receives vasopressin and oxytocin from the hypothalamus and releases them into the blood.
The Cerebral Hemispheres
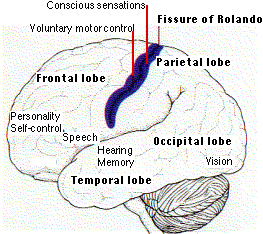
Each hemisphere of the cerebrum is subdivided into four lobes visible from the outside:
Hidden beneath these regions of each cerebral cortex is
- An olfactory bulb ; they receive input from the olfactory epithelia.
- A striatum ; they receive input from the frontal lobes and also from the limbic system (below). At the base of each striatum is a nucleus accumbens (NA).
The pleasurable (and addictive) effects of amphetamines, cocaine, and perhaps other psychoactive drugs seem to depend on their producing increasing levels of dopamine at the synapses in the nucleus accumbens (as well as the VTA).
- hippocampus . It is essential for the formation of long-term memories.
- vasopressin whose activation increases aggressiveness and other signs of the flight or fight response
- oxytocin whose activation lessens the signs of stress
The amygdala receives a rich supply of signals from the olfactory system, and this may account for the powerful effect that odor has on emotions (and evoking memories).
Mapping the Functions of the Brain
It is estimated that the human brain contains some 86 billion (8.6 x 10 10 ) neurons averaging 10,000 synapses on each; that is, almost 10 15 connections. How to unravel the workings of such a complex system?
Several methods have been useful.
Microscopic examination with the aid of selective stains has revealed many of the physical connections created by axons in the brain.
The Electroencephalograph (EEG)
This device measures electrical activity (brain "waves") that can be detected at the surface of the scalp. It can distinguish between, for example, sleep and excitement. It is also useful in diagnosing brain disorders such as a tendency to epileptic seizures.
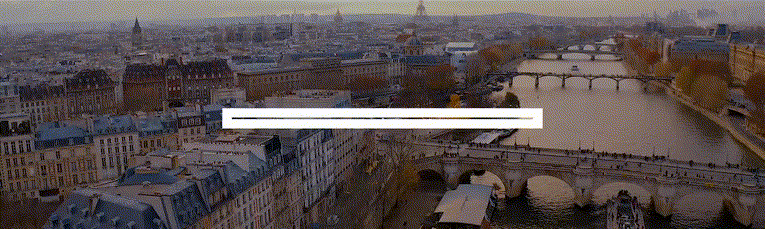
Damage to the Brain
Many cases of brain damage from, for example,
- strokes (interruption of blood flow to a part of the brain)
- tumors in the brain
- mechanical damage (e.g., bullet wounds)
have provided important insights into the functions of various parts of the brain.
Battlefield injury to the left temporal lobe of the cerebrum interferes with speech.
Example 2: Phineas P. Gage
In 1848, an accidental explosion drove a metal bar completely through the frontal lobes of Phineas P. Gage. Not only did he survive the accident, he never even lost consciousness or any of the clearly-defined functions of the brain. However, over the ensuing years, he underwent a marked change in personality. Formerly described as a reasonable, sober, conscientious person, he became — in the words of those observing him — "thoughtless, irresponsible, fitful, obstinate, and profane". In short, his personality had changed, but his vision, hearing, other sensations, speech, and body coordination were unimpaired. (Similar personality changes have since been often observed in people with injuries to their prefrontal cortex.)
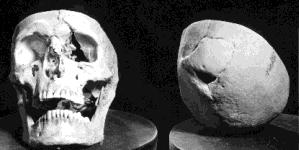
The photograph (courtesy of the Warren Anatomical Museum, Harvard University Medical School) shows Gage's skull where the bar entered (left) and exited (right) in the accident (which occurred 12 years before he died of natural causes in 1861).
Stimulating the exposed brain with electrodes
There are no pain receptors on the surface of the brain, and some humans undergoing brain surgery have volunteered to have their exposed brain stimulated with electrodes during surgery. When not under general anesthesia, they can even report their sensations to the experimenter. Experiments of this sort have revealed a band of cortex running parallel to and just in front of the fissure of Rolando that controls the contraction of skeletal muscles. Stimulation of tiny spots within this motor area causes contraction of the muscles.
The area of motor cortex controlling a body part is not proportional to the size of that part but is proportional to the number of motor neurons running to it. The more motor neurons that activate a structure, the more precisely it can be controlled. Thus the areas of the motor cortex controlling the hands and lips are much larger than those controlling the muscles of the torso and legs. A similar region is located in a parallel band of cortex just behind the fissure of Rolando. This region is concerned with sensation from the various parts of the body. When spots in this sensory area are stimulated, the patient reports sensations in a specific area of the body. A map can be made based on these reports. When portions of the occipital lobe are stimulated electrically, the patient reports light. However, this region is also needed for associations to be made with what is seen. Damage to regions in the occipital lobe results in the person's being perfectly able to see objects but incapable of recognizing them.
The centers of hearing — and understanding what is heard — are located in the temporal lobes .
CT = X-ray C omputed T omography
This is an imaging technique that uses a series of X-ray exposures taken from different angles. Computer software can integrate these to produce a three-dimensional picture of the brain (or other body region). CT scanning is routinely used to quickly diagnose strokes.
PET = P ositron- E mission T omography
This imaging technique requires that the subject be injected with a radioisotope that emits positrons.
- Water labeled with oxygen-15 (H 2 15 O) is used to measure changes in blood flow (which increases in parts of the brain that are active). The short half-life of 15 O (2 minutes) makes it safe to use.
- Deoxyglucose labeled with fluorine-18. The brain has a voracious appetite for glucose (although representing only ~2% of our body weight, the brain receives ~15% of the blood pumped by the heart and consumes ~20% of the energy produced by cellular respiration when we are at rest). When supplied with deoxyglucose, the cells are tricked into taking in this related molecule and phosphorylating it in the first step of glycolysis. But no further processing occurs so it accumulates in the cell. By coupling a short-lived radioactive isotope like 18 F to the deoxyglucose and using a PET scanner, it is possible to visualize active regions of the brain.
The images in fig. 15.8.3.8 (courtesy of Michael E. Phelps from Science 211 :445, 1981) were produced in a PET scanner. The dark areas are regions of high metabolic activity. Note how the metabolism of the occipital lobes (arrows) increases when visual stimuli are received.
Similarly, sounds increase the rate of deoxyglucose uptake in the speech areas of the temporal lobe.
The image in fig. 15.8.3.9 on the right (courtesy of Gary H. Duncan from Talbot, J. D., et. al., Science 251 : 1355, 1991) shows activation of the cerebral cortex by a hot probe (which the subjects describe as painful) applied to the forearm.
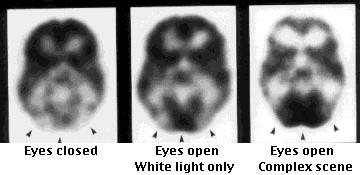
Most cancers consume large amounts of glucose (cellular respiration is less efficient than in normal cells so they must rely more on the inefficient process of glycolysis). Therefore PET scanning with 18 F-fluorodeoxyglucose is commonly used to monitor both the primary tumor and any metastases.
MRI = M agnetic R esonance I maging
This imaging technique uses powerful magnets to detect magnetic molecules within the body. These can be endogenous molecules or magnetic substances injected into a vein.
fMRI = Functional Magnetic Resonance Imaging
fMRI exploits the changes in the magnetic properties of hemoglobin as it carries oxygen. Activation of a part of the brain increases oxygen levels there increasing the ratio of oxyhemoglobin to deoxyhemoglobin.
The probable mechanism:
- The increased demand for neurotransmitters must be met by increased production of ATP.
- Although this consumes oxygen (needed for cellular respiration),
- it also increases the blood flow to the area.
- So there is an increase and not a decrease in the oxygen supply to the region, which provides the signal detected by fMRI.
Magnetoencephalography (MEG)
MEG detects the tiny magnetic fields created as individual neurons "fire" within the brain. It can pinpoint the active region with a millimeter, and can follow the movement of brain activity as it travels from region to region within the brain. MEG is noninvasive requiring only that the subject's head lie within a helmet containing the magnetic sensors.
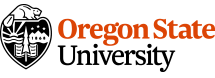
14.5 Sensory and Motor Pathways
Learning objectives.
By the end of this section, you will be able to:
- Describe the pathways that sensory systems follow into the central nervous system
- Differentiate between the two major ascending pathways in the spinal cord
- Describe the pathway of somatosensory input from the face and compare it to the ascending pathways in the spinal cord
- Explain topographical representations of sensory information in at least two systems
- List the components of the basic processing stream for the motor system
- Describe the pathway of descending motor commands from the cortex to the skeletal muscles
- Compare different descending pathways, both by structure and function
- Explain the initiation of movement from the neurological connections
- Describe several reflex arcs and their functional roles
Spinal Nerves
Generally, spinal nerves contain afferent axons from sensory receptors in the periphery, such as from the skin, mixed with efferent axons travelling to the muscles or other effector organs. As the spinal nerve nears the spinal cord, it splits into dorsal and ventral roots. The dorsal root contains only the axons of sensory neurons, whereas the ventral roots contain only the axons of the motor neurons. Some of the branches will synapse with local neurons in the dorsal root ganglion, posterior (dorsal) horn, or even the anterior (ventral) horn, at the level of the spinal cord where they enter. Other branches will travel a short distance up or down the spine to interact with neurons at other levels of the spinal cord. A branch may also turn into the posterior (dorsal) column of the white matter to connect with the brain. For the sake of convenience, we will use the terms ventral and dorsal in reference to structures within the spinal cord that are part of these pathways. This will help to underscore the relationships between the different components. Typically, spinal nerve systems that connect to the brain are contralateral , in that the right side of the body is connected to the left side of the brain and the left side of the body to the right side of the brain.
Cranial Nerves
Cranial nerves convey specific sensory information from the head and neck directly to the brain. For sensations below the neck, the right side of the body is connected to the left side of the brain and the left side of the body to the right side of the brain. Whereas spinal information is contralateral, cranial nerve systems are mostly ipsilateral , meaning that a cranial nerve on the right side of the head is connected to the right side of the brain. Some cranial nerves contain only sensory axons, such as the olfactory, optic, and vestibulocochlear nerves. Other cranial nerves contain both sensory and motor axons, including the trigeminal, facial, glossopharyngeal, and vagus nerves (however, the vagus nerve is not associated with the somatic nervous system). The general senses of somatosensation for the face travel through the trigeminal system.
Sensory Pathways
Specific regions of the CNS coordinate different somatic processes using sensory inputs and motor outputs of peripheral nerves. A simple case is a reflex caused by a synapse between a dorsal sensory neuron axon and a motor neuron in the ventral horn. More complex arrangements are possible to integrate peripheral sensory information with higher processes. The important regions of the CNS that play a role in somatic processes can be separated into the spinal cord brain stem, diencephalon, cerebral cortex, and subcortical structures.
Spinal Cord and Brain Stem
A sensory pathway that carries peripheral sensations to the brain is referred to as an ascending pathway , or ascending tract. The various sensory modalities each follow specific pathways through the CNS. Tactile and other somatosensory stimuli activate receptors in the skin, muscles, tendons, and joints throughout the entire body. However, the somatosensory pathways are divided into two separate systems on the basis of the location of the receptor neurons. Somatosensory stimuli from below the neck pass along the sensory pathways of the spinal cord, whereas somatosensory stimuli from the head and neck travel through the cranial nerves—specifically, the trigeminal system.
The dorsal column system (sometimes referred to as the dorsal column–medial lemniscus) and the spinothalamic tract are two major pathways that bring sensory information to the brain ( Figure 14.5.1 ). The sensory pathways in each of these systems are composed of three successive neurons.
The dorsal column system begins with the axon of a dorsal root ganglion neuron entering the dorsal root and joining the dorsal column white matter in the spinal cord. As axons of this pathway enter the dorsal column, they take on a positional arrangement so that axons from lower levels of the body position themselves medially, whereas axons from upper levels of the body position themselves laterally. The dorsal column is separated into two component tracts, the fasciculus gracilis that contains axons from the legs and lower body, and the fasciculus cuneatus that contains axons from the upper body and arms.
The axons in the dorsal column terminate in the nuclei of the medulla, where each synapses with the second neuron in their respective pathway. The nucleus gracilis is the target of fibers in the fasciculus gracilis, whereas the nucleus cuneatus is the target of fibers in the fasciculus cuneatus. The second neuron in the system projects from one of the two nuclei and then decussates , or crosses the midline of the medulla. These axons then continue to ascend the brain stem as a bundle called the medial lemniscus . These axons terminate in the thalamus, where each synapses with the third neuron in their respective pathway. The third neuron in the system projects its axons to the postcentral gyrus of the cerebral cortex, where somatosensory stimuli are initially processed and the conscious perception of the stimulus occurs.
The spinothalamic tract also begins with neurons in a dorsal root ganglion. These neurons extend their axons to the dorsal horn, where they synapse with the second neuron in their respective pathway. The name “spinothalamic” comes from this second neuron, which has its cell body in the spinal cord gray matter and connects to the thalamus. Axons from these second neurons then decussate within the spinal cord and ascend to the brain and enter the thalamus, where each synapses with the third neuron in its respective pathway. The neurons in the thalamus then project their axons to the spinothalamic tract, which synapses in the postcentral gyrus of the cerebral cortex.
These two systems are similar in that they both begin with dorsal root ganglion cells, as with most general sensory information. The dorsal column system is primarily responsible for touch sensations and proprioception, whereas the spinothalamic tract pathway is primarily responsible for pain and temperature sensations. Another similarity is that the second neurons in both of these pathways are contralateral, because they project across the midline to the other side of the brain or spinal cord. In the dorsal column system, this decussation takes place in the brain stem; in the spinothalamic pathway, it takes place in the spinal cord at the same spinal cord level at which the information entered. The third neurons in the two pathways are essentially the same. In both, the second neuron synapses in the thalamus, and the thalamic neuron projects to the somatosensory cortex.
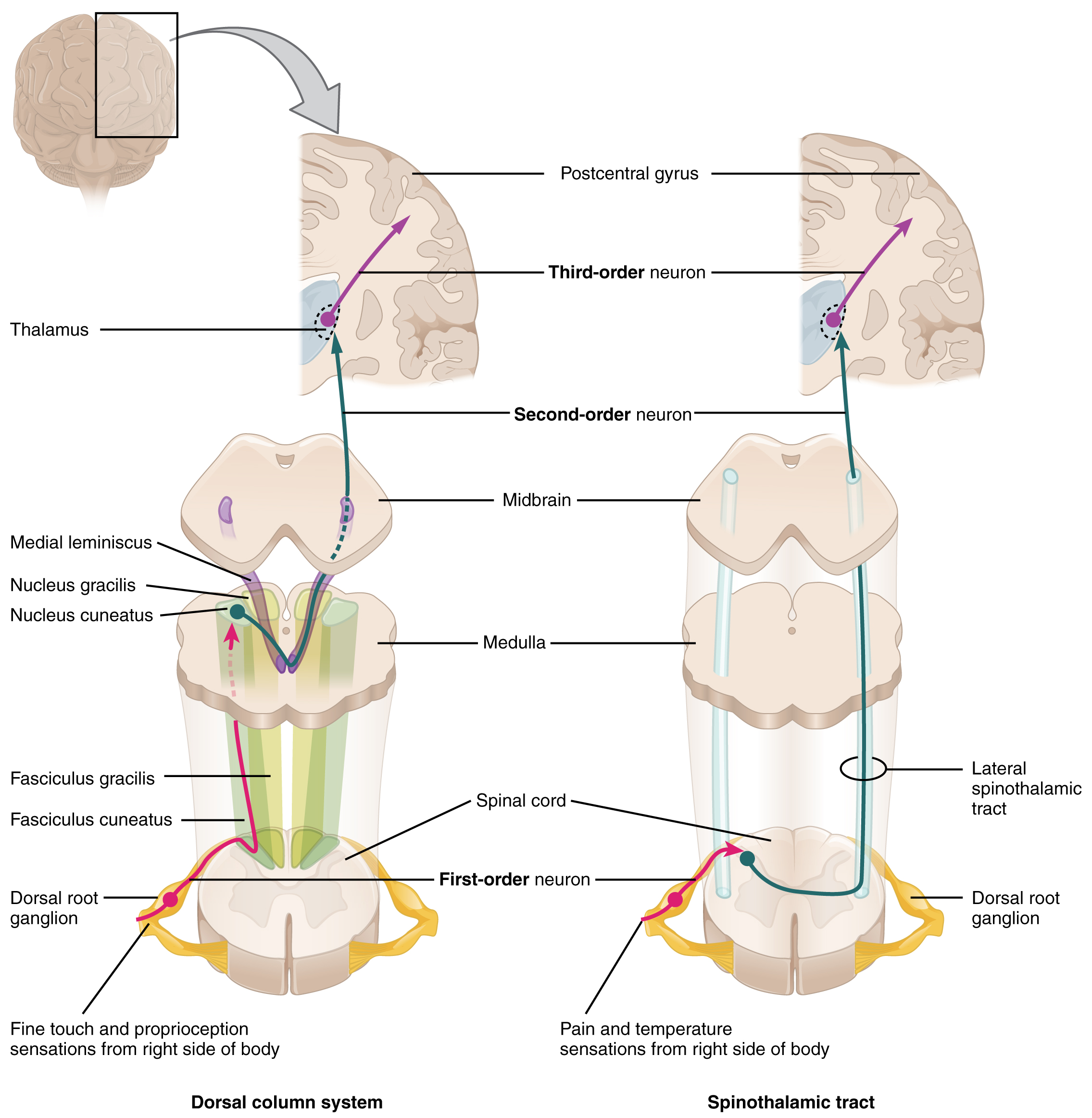
The trigeminal pathway carries somatosensory information from the face, head, mouth, and nasal cavity. As with the previously discussed nerve tracts, the sensory pathways of the trigeminal pathway each involve three successive neurons. First, axons from the trigeminal ganglion enter the brain stem at the level of the pons. These axons project to one of three locations. The spinal trigeminal nucleus of the medulla receives information similar to that carried by spinothalamic tract, such as pain and temperature sensations. Other axons go to either the chief sensory nucleus in the pons or the mesencephalic nuclei in the midbrain. These nuclei receive information like that carried by the dorsal column system, such as touch, pressure, vibration, and proprioception. Axons from the second neuron decussate and ascend to the thalamus along the trigeminothalamic tract. In the thalamus, each axon synapses with the third neuron in its respective pathway. Axons from the third neuron then project from the thalamus to the primary somatosensory cortex of the cerebrum.
Diencephalon
The diencephalon is beneath the cerebrum and includes the thalamus and hypothalamus. In the somatic nervous system, the thalamus is an important relay for communication between the cerebrum and the rest of the nervous system. The hypothalamus has both somatic and autonomic functions. In addition, the hypothalamus communicates with the limbic system, which controls emotions and memory functions.
Sensory input to the thalamus comes from most of the special senses and ascending somatosensory tracts. Each sensory system is relayed through a particular nucleus in the thalamus. The thalamus is a required transfer point for most sensory tracts that reach the cerebral cortex, where conscious sensory perception begins. The one exception to this rule is the olfactory system. The olfactory tract axons from the olfactory bulb project directly to the cerebral cortex, along with the limbic system and hypothalamus.
The thalamus is a collection of several nuclei that can be categorized into three anatomical groups. White matter running through the thalamus defines the three major regions of the thalamus, which are an anterior nucleus, a medial nucleus, and a lateral group of nuclei. The anterior nucleus serves as a relay between the hypothalamus and the emotion and memory-producing limbic system. The medial nuclei serve as a relay for information from the limbic system and basal ganglia to the cerebral cortex. This allows memory creation during learning, but also determines alertness. The special and somatic senses connect to the lateral nuclei, where their information is relayed to the appropriate sensory cortex of the cerebrum.
Cortical Processing
As described earlier, many of the sensory axons are positioned in the same way as their corresponding receptor cells in the body. This allows identification of the position of a stimulus on the basis of which receptor cells are sending information. The cerebral cortex also maintains this sensory topography in the particular areas of the cortex that correspond to the position of the receptor cells. The somatosensory cortex provides an example in which, in essence, the locations of the somatosensory receptors in the body are mapped onto the somatosensory cortex. This mapping is often depicted using a sensory homunculus ( Figure 14.5.2 ).
The term homunculus comes from the Latin word for “little man” and refers to a map of the human body that is laid across a portion of the cerebral cortex. In the somatosensory cortex, the external genitals, feet, and lower legs are represented on the medial face of the gyrus within the longitudinal fissure. As the gyrus curves out of the fissure and along the surface of the parietal lobe, the body map continues through the thighs, hips, trunk, shoulders, arms, and hands. The head and face are just lateral to the fingers as the gyrus approaches the lateral sulcus. The representation of the body in this topographical map is medial to lateral from the lower to upper body. It is a continuation of the topographical arrangement seen in the dorsal column system, where axons from the lower body are carried in the fasciculus gracilis, whereas axons from the upper body are carried in the fasciculus cuneatus. As the dorsal column system continues into the medial lemniscus, these relationships are maintained. Also, the head and neck axons running from the trigeminal nuclei to the thalamus run adjacent to the upper body fibers. The connections through the thalamus maintain topography such that the anatomic information is preserved. Note that this correspondence does not result in a perfectly miniature scale version of the body, but rather exaggerates the more sensitive areas of the body, such as the fingers and lower face. Less sensitive areas of the body, such as the shoulders and back, are mapped to smaller areas on the cortex.
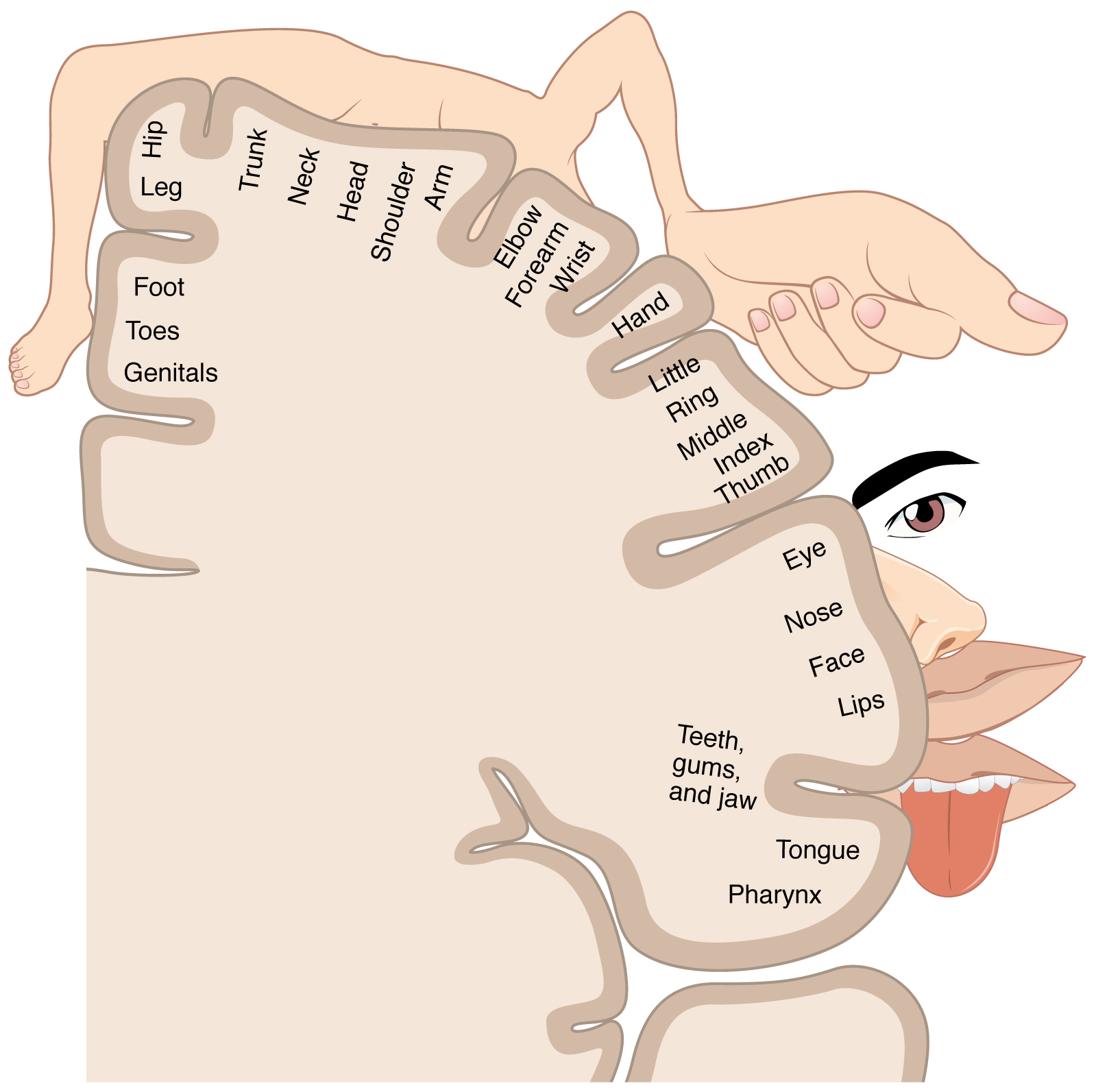
The cortex has been described as having specific regions that are responsible for processing specific information; there is the visual cortex, somatosensory cortex, gustatory cortex, etc. However, our experience of these senses is not divided. Instead, we experience what can be referred to as a seamless percept. Our perceptions of the various sensory modalities—though distinct in their content—are integrated by the brain so that we experience the world as a continuous whole.
In the cerebral cortex, sensory processing begins at the primary sensory cortex , then proceeds to an association area , and finally, into a multimodal integration area . For example, somatosensory information inputs directly into the primary somatosensory cortex in the post-central gyrus of the parietal lobe where general awareness of sensation (location and type of sensation) begins. In the somatosensory association cortex details are integrated into a whole. In the highest level of association cortex details are integrated from entirely different modalities to form complete representations as we experience them.
Motor Responses
The defining characteristic of the somatic nervous system is that it controls skeletal muscles. Somatic senses inform the nervous system about the external environment, but the response to that is through voluntary muscle movement. The term “voluntary” suggests that there is a conscious decision to make a movement. However, some aspects of the somatic system use voluntary muscles without conscious control. One example is the ability of our breathing to switch to unconscious control while we are focused on another task. However, the muscles that are responsible for the basic process of breathing are also utilized for speech, which is entirely voluntary.
Cortical Responses
Let’s start with sensory stimuli that have been registered through receptor cells and the information relayed to the CNS along ascending pathways. In the cerebral cortex, the initial processing of sensory perception progresses to associative processing and then integration in multimodal areas of cortex. These levels of processing can lead to the incorporation of sensory perceptions into memory, but more importantly, they lead to a response. The completion of cortical processing through the primary, associative, and integrative sensory areas initiates a similar progression of motor processing, usually in different cortical areas.
Whereas the sensory cortical areas are located in the occipital, temporal, and parietal lobes, motor functions are largely controlled by the frontal lobe. The most anterior regions of the frontal lobe—the prefrontal areas—are important for executive functions , which are those cognitive functions that lead to goal-directed behaviors. These higher cognitive processes include working memory , which has been called a “mental scratch pad,” that can help organize and represent information that is not in the immediate environment. The prefrontal lobe is responsible for aspects of attention, such as inhibiting distracting thoughts and actions so that a person can focus on a goal and direct behavior toward achieving that goal.
The functions of the prefrontal cortex are integral to the personality of an individual, because it is largely responsible for what a person intends to do and how they accomplish those plans. A famous case of damage to the prefrontal cortex is that of Phineas Gage, dating back to 1848. He was a railroad worker who had a metal spike impale his prefrontal cortex ( Figure 14.5.3 ). He survived the accident, but according to second-hand accounts, his personality changed drastically. Friends described him as no longer acting like himself. Whereas he was a hardworking, amiable man before the accident, he turned into an irritable, temperamental, and lazy man after the accident. Many of the accounts of his change may have been inflated in the retelling, and some behavior was likely attributable to alcohol used as a pain medication. However, the accounts suggest that some aspects of his personality did change. Also, there is new evidence that though his life changed dramatically, he was able to become a functioning stagecoach driver, suggesting that the brain has the ability to recover even from major trauma such as this.
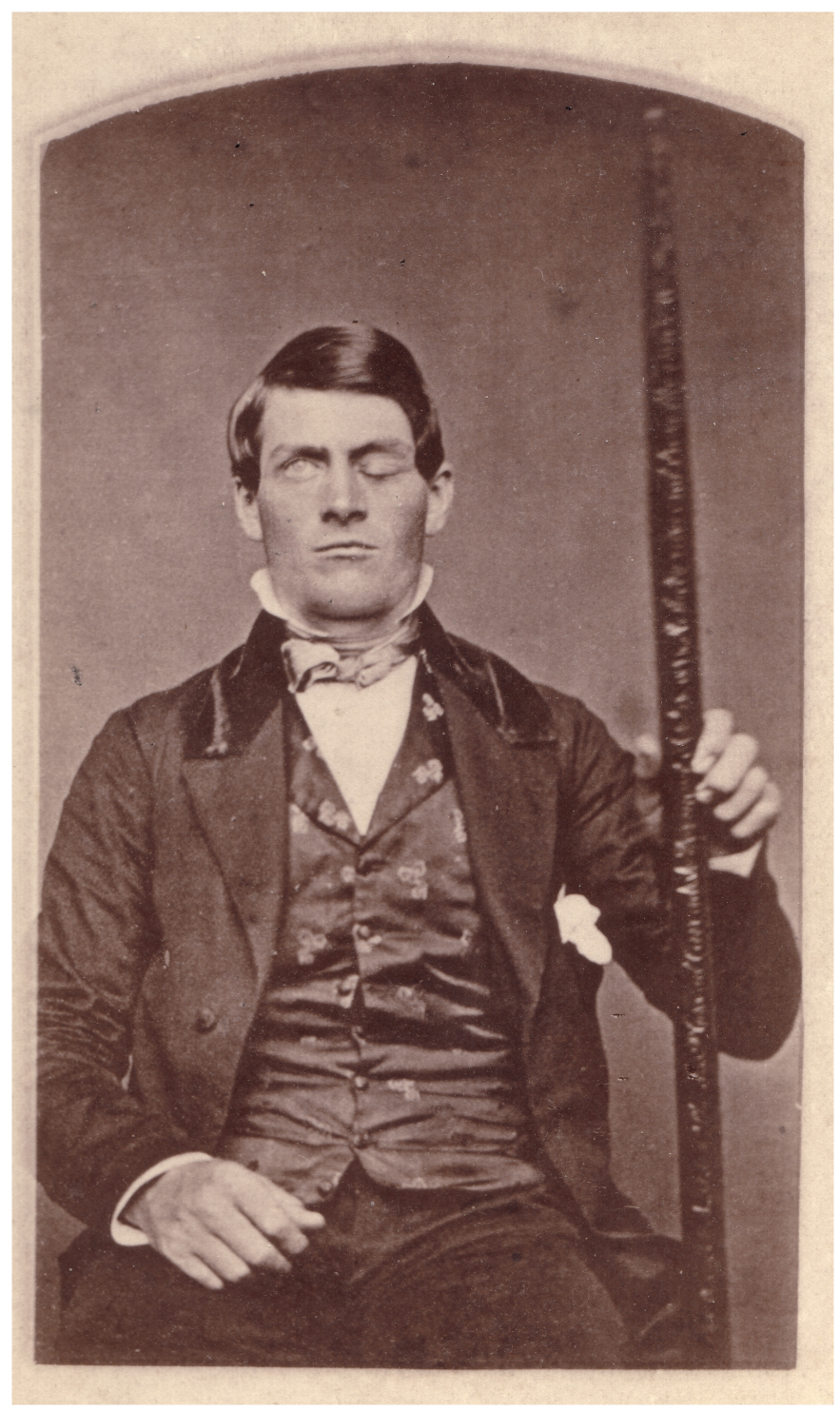
Secondary Motor Cortices
In generating motor responses, the executive functions of the prefrontal cortex will need to initiate actual movements. One way to define the prefrontal area is any region of the frontal lobe that does not elicit movement when electrically stimulated. These are primarily in the anterior part of the frontal lobe. The regions of the frontal lobe that remain are the regions of the cortex that produce movement. The prefrontal areas project into the secondary motor cortices, which include the premotor cortex and the supplemental motor area .
Two important regions that assist in planning and coordinating movements are located adjacent to the primary motor cortex. The premotor cortex is more lateral, whereas the supplemental motor area is more medial and superior. The premotor area aids in controlling movements of the core muscles to maintain posture during movement, whereas the supplemental motor area is hypothesized to be responsible for planning and coordinating movement. The supplemental motor area also manages sequential movements that are based on prior experience (that is, learned movements). Neurons in these areas are most active leading up to the initiation of movement. For example, these areas might prepare the body for the movements necessary to drive a car in anticipation of a traffic light changing.
Adjacent to these two regions are two specialized motor planning centers. The frontal eye fields are responsible for moving the eyes in response to visual stimuli. There are direct connections between the frontal eye fields and the superior colliculus. Also, anterior to the premotor cortex and primary motor cortex is Broca’s area . This area is responsible for controlling movements of the structures of speech production. The area is named after a French surgeon and anatomist who studied patients who could not produce speech. They did not have impairments to understanding speech, only to producing speech sounds, suggesting a damaged or underdeveloped Broca’s area.
Primary Motor Cortex
The primary motor cortex is located in the precentral gyrus of the frontal lobe. A neurosurgeon, Walter Penfield, described much of the basic understanding of the primary motor cortex by electrically stimulating the surface of the cerebrum. Penfield would probe the surface of the cortex while the patient was only under local anesthesia so that he could observe responses to the stimulation. This led to the belief that the precentral gyrus directly stimulated muscle movement. We now know that the primary motor cortex receives input from several areas that aid in planning movement, and its principle output stimulates spinal cord neurons to stimulate skeletal muscle contraction.
The primary motor cortex is arranged in a similar fashion to the primary somatosensory cortex, in that it has a topographical map of the body, creating a motor homunculus (see Chapter 14.2 Figure 14.2.5 ). The neurons responsible for musculature in the feet and lower legs are in the medial wall of the precentral gyrus, with the thighs, trunk, and shoulder at the crest of the longitudinal fissure. The hand and face are in the lateral face of the gyrus. Also, the relative space allotted for the different regions is exaggerated in muscles that have greater enervation. The greatest amount of cortical space is given to muscles that perform fine, agile movements, such as the muscles of the fingers and the lower face that are parts of small motor units. The “power muscles” that perform coarser movements, such as the buttock and back muscles, occupy much less space on the motor cortex.
Descending Pathways
The motor output from the cortex descends into the brain stem and to the spinal cord to control the musculature through motor neurons. Neurons located in the primary motor cortex, named Betz cells , are large cortical neurons that synapse with lower motor neurons in the spinal cord or the brain stem. The two descending pathways travelled by the axons of Betz cells are the corticospinal tract and the corticobulbar tract . Both tracts are named for their origin in the cortex and their targets—either the spinal cord or the brain stem (the term “bulbar” refers to the brain stem as the bulb, or enlargement, at the top of the spinal cord).
These two descending pathways are responsible for the conscious or voluntary movements of skeletal muscles. Any motor command from the primary motor cortex is sent down the axons of the Betz cells to activate upper motor neurons in either the cranial motor nuclei or in the ventral horn of the spinal cord. The axons of the corticobulbar tract are ipsilateral, meaning they project from the cortex to the motor nucleus on the same side of the nervous system. Conversely, the axons of the corticospinal tract are largely contralateral, meaning that they cross the midline of the brain stem or spinal cord and synapse on the opposite side of the body. Therefore, the right motor cortex of the cerebrum controls muscles on the left side of the body, and vice versa.
The corticospinal tract descends from the cortex through the deep white matter of the cerebrum. It then passes between the caudate nucleus and putamen of the basal nuclei as a bundle called the internal capsule . The tract then passes through the midbrain as the cerebral peduncles , after which it burrows through the pons. Upon entering the medulla, the tracts make up the large white matter tract referred to as the pyramids ( Figure 14.5.4 ). The defining landmark of the medullary-spinal border is the pyramidal decussation , which is where most of the fibers in the corticospinal tract cross over to the opposite side of the brain. At this point, the tract separates into two parts, which have control over different domains of the musculature.
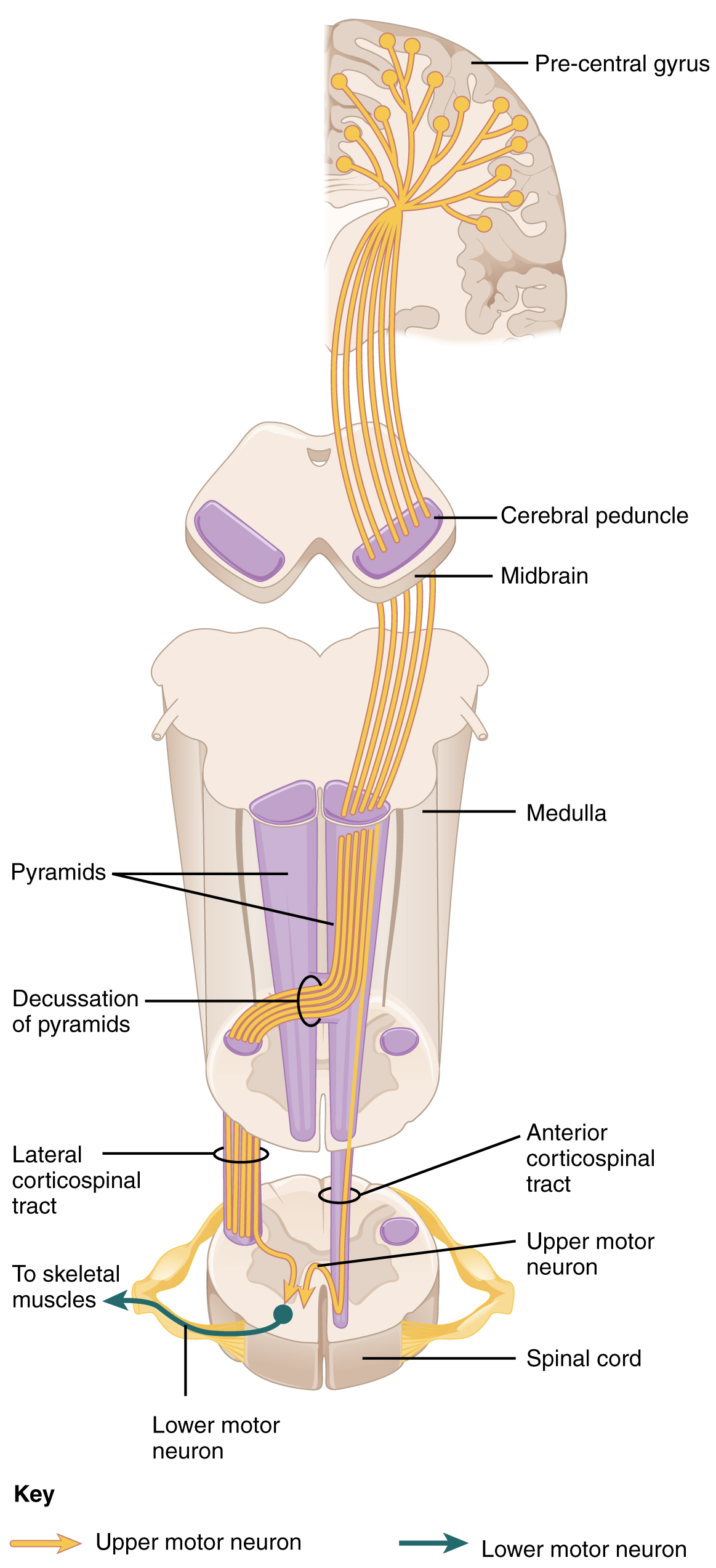
Appendicular Control
The lateral corticospinal tract is composed of the fibers that cross the midline at the pyramidal decussation (see Figure 14.5.4 ). The axons cross over from the anterior position of the pyramids in the medulla to the lateral column of the spinal cord. These axons are responsible for controlling appendicular muscles.
This influence over the appendicular muscles means that the lateral corticospinal tract is responsible for moving the muscles of the arms and legs. The ventral horn in both the lower cervical spinal cord and the lumbar spinal cord both have wider ventral horns, representing the greater number of muscles controlled by these motor neurons. The cervical enlargement is particularly large because there is greater control over the fine musculature of the upper limbs, particularly of the fingers. The lumbar enlargement is not as significant in appearance because there is less fine motor control of the lower limbs.
Axial Control
The anterior corticospinal tract is responsible for controlling the muscles of the body trunk (see Figure 14.5.4 ). These axons do not decussate in the medulla. Instead, they remain in an anterior position as they descend the brain stem and enter the spinal cord. These axons then travel to the spinal cord level at which they synapse with a lower motor neuron. Upon reaching the appropriate level, the axons decussate, entering the ventral horn on the opposite side of the spinal cord from which they entered. In the ventral horn, these axons synapse with their corresponding lower motor neurons. The lower motor neurons are located in the medial regions of the ventral horn, because they control the axial muscles of the trunk.
Because movements of the body trunk involve both sides of the body, the anterior corticospinal tract is not entirely contralateral. Some collateral branches of the tract will project into the ipsilateral ventral horn to control synergistic muscles on that side of the body, or to inhibit antagonistic muscles through interneurons within the ventral horn. Through the influence of both sides of the body, the anterior corticospinal tract can coordinate postural muscles in broad movements of the body. These coordinating axons in the anterior corticospinal tract are often considered bilateral, as they are both ipsilateral and contralateral.
External Website
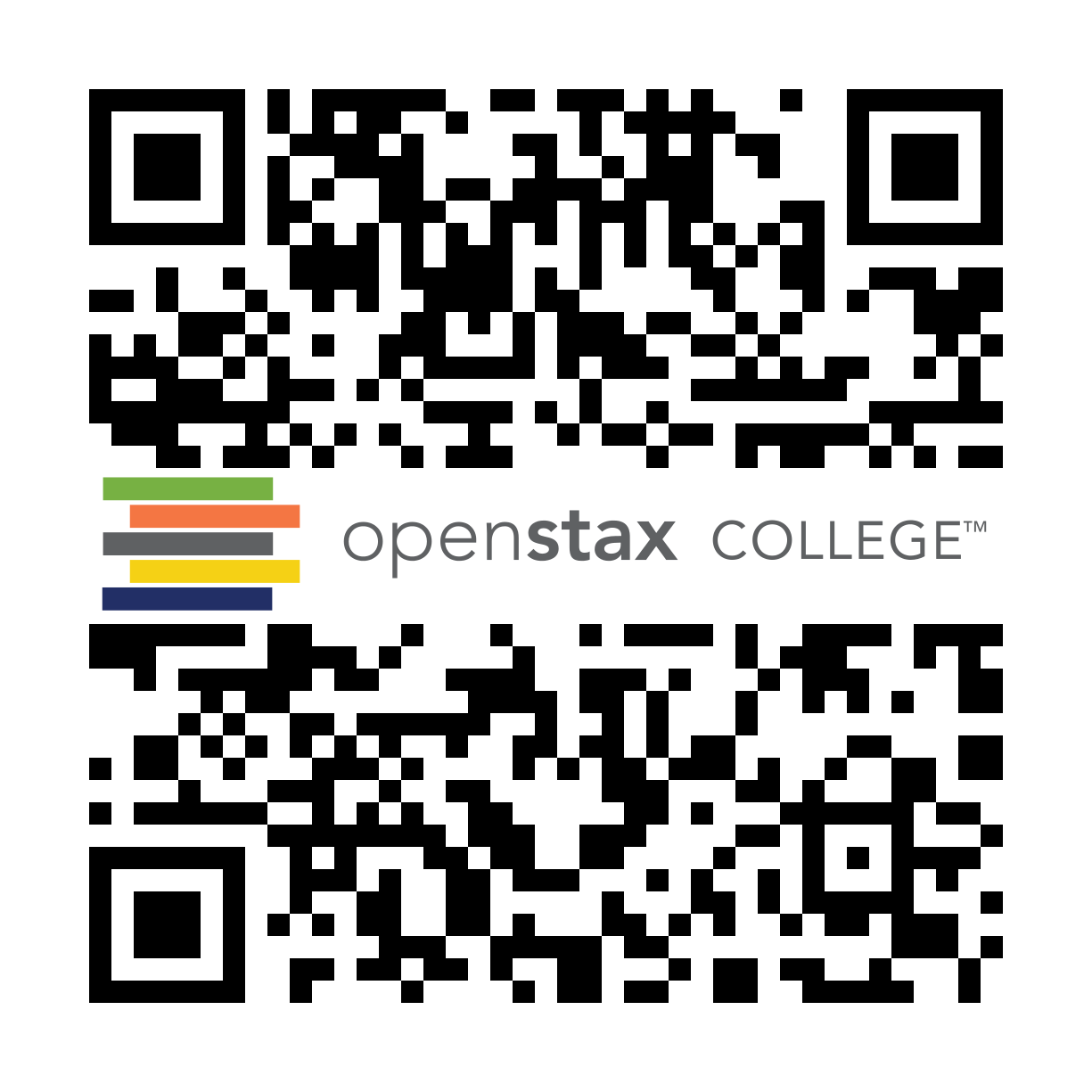
Watch this video to learn more about the descending motor pathway for the somatic nervous system. The autonomic connections are mentioned, which are covered in another chapter. From this brief video, only some of the descending motor pathway of the somatic nervous system is described. Which division of the pathway is described and which division is left out?
Extrapyramidal Controls
Other descending connections between the brain and the spinal cord are called the extrapyramidal system . The name comes from the fact that this system is outside the corticospinal pathway, which includes the pyramids in the medulla. A few pathways originating from the brain stem contribute to this system.
The tectospinal tract projects from the midbrain to the spinal cord and is important for postural movements that are driven by the superior colliculus. The name of the tract comes from an alternate name for the superior colliculus, which is the tectum. The reticulospinal tract connects the reticular system, a diffuse region of gray matter in the brain stem, with the spinal cord. This tract influences trunk and proximal limb muscles related to posture and locomotion. The reticulospinal tract also contributes to muscle tone and influences autonomic functions. The vestibulospinal tract connects the brain stem nuclei of the vestibular system with the spinal cord. This allows posture, movement, and balance to be modulated on the basis of equilibrium information provided by the vestibular system.
The pathways of the extrapyramidal system are influenced by subcortical structures. For example, connections between the secondary motor cortices and the extrapyramidal system modulate spine and cranium movements. The basal nuclei, which are important for regulating movement initiated by the CNS, influence the extrapyramidal system as well as its thalamic feedback to the motor cortex.
The conscious movement of our muscles is more complicated than simply sending a single command from the precentral gyrus down to the proper motor neurons. During the movement of any body part, our muscles relay information back to the brain, and the brain is constantly sending “revised” instructions back to the muscles. The cerebellum is important in contributing to the motor system because it compares cerebral motor commands with proprioceptive feedback. The corticospinal fibers that project to the ventral horn of the spinal cord have branches that also synapse in the pons, which project to the cerebellum. Also, the proprioceptive sensations of the dorsal column system have a collateral projection to the medulla that projects to the cerebellum. These two streams of information are compared in the cerebellar cortex. Conflicts between the motor commands sent by the cerebrum and body position information provided by the proprioceptors cause the cerebellum to stimulate the red nucleus of the midbrain. The red nucleus then sends corrective commands to the spinal cord along the rubrospinal tract . The name of this tract comes from the word for red that is seen in the English word “ruby.”
A good example of how the cerebellum corrects cerebral motor commands can be illustrated by walking in water. An original motor command from the cerebrum to walk will result in a highly coordinated set of learned movements. However, in water, the body cannot actually perform a typical walking movement as instructed. The cerebellum can alter the motor command, stimulating the leg muscles to take larger steps to overcome the water resistance. The cerebellum can make the necessary changes through the rubrospinal tract. Modulating the basic command to walk also relies on spinal reflexes, but the cerebellum is responsible for calculating the appropriate response. When the cerebellum does not work properly, coordination and balance are severely affected. The most dramatic example of this is during the overconsumption of alcohol. Alcohol inhibits the ability of the cerebellum to interpret proprioceptive feedback, making it more difficult to coordinate body movements, such as walking a straight line, or guide the movement of the hand to touch the tip of the nose.
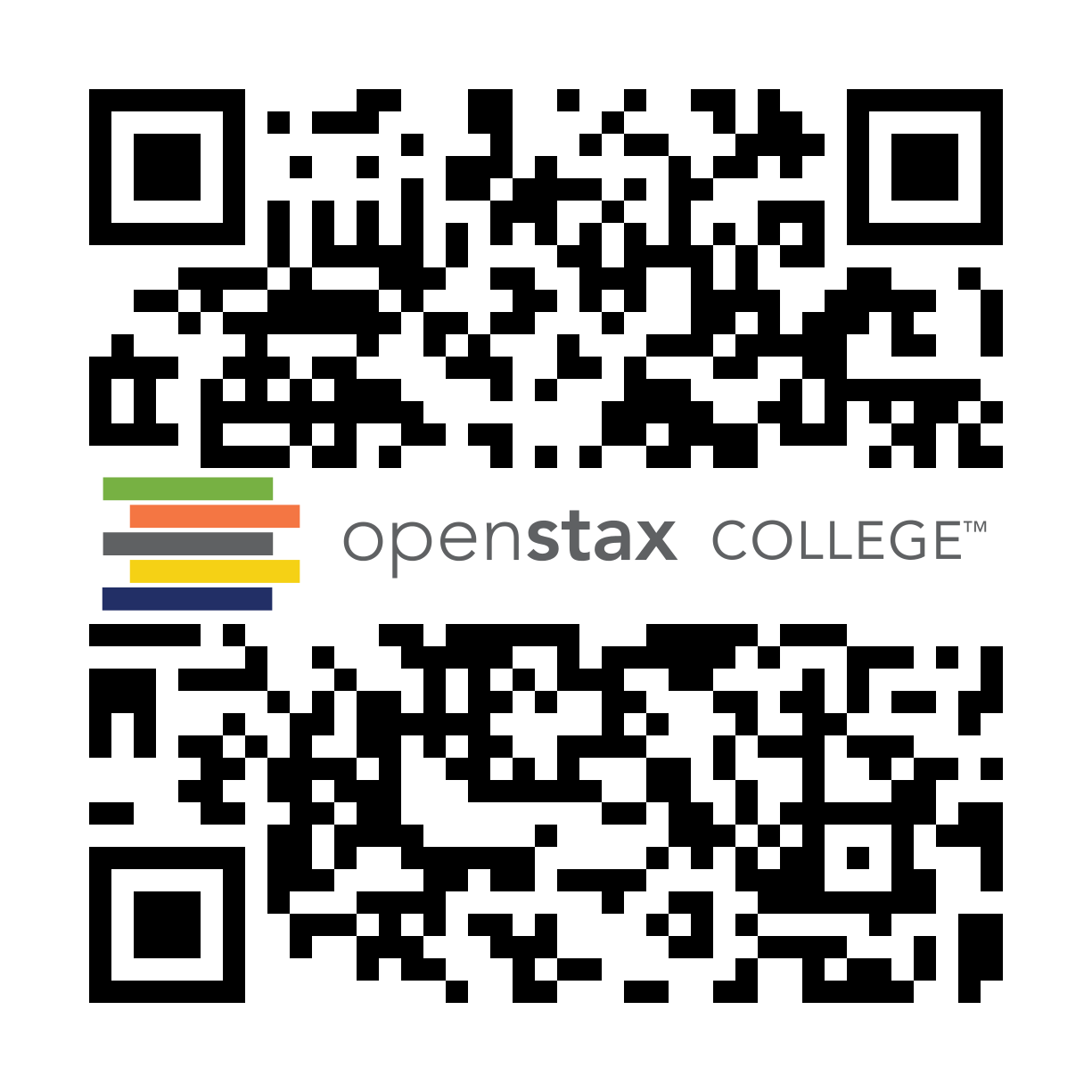
Visit this site to read about an elderly woman who starts to lose the ability to control fine movements, such as speech and the movement of limbs. Many of the usual causes were ruled out. It was not a stroke, Parkinson’s disease, diabetes, or thyroid dysfunction. The next most obvious cause was medication, so her pharmacist had to be consulted. The side effect of a drug meant to help her sleep had resulted in changes in motor control. What regions of the nervous system are likely to be the focus of haloperidol side effects?
The Sensory and Motor Exams
Connections between the body and the CNS occur through the spinal cord. The cranial nerves connect the head and neck directly to the brain, but the spinal cord receives sensory input and sends motor commands out to the body through the spinal nerves. Whereas the brain develops into a complex series of nuclei and fiber tracts, the spinal cord remains relatively simple in its configuration ( Figure 14.5.5 ). From the initial neural tube early in embryonic development, the spinal cord retains a tube-like structure with gray matter surrounding the small central canal and white matter on the surface in three columns. The dorsal, or posterior, horns of the gray matter are mainly devoted to sensory functions whereas the ventral, or anterior, and lateral horns are associated with motor functions. In the white matter, the dorsal column relays sensory information to the brain, and the anterior column is almost exclusively relaying motor commands to the ventral horn motor neurons. The lateral column, however, conveys both sensory and motor information between the spinal cord and brain.
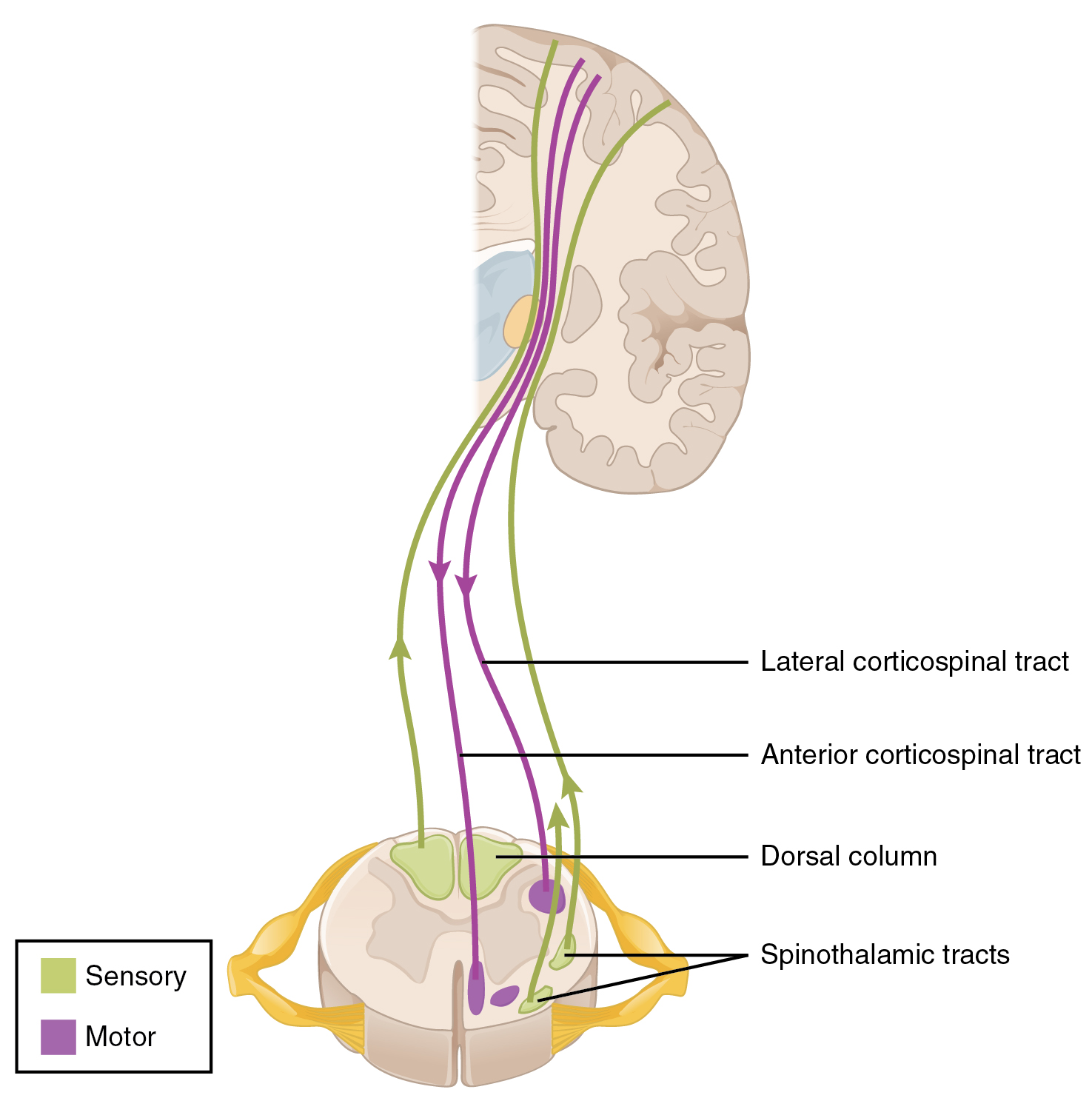
Sensory Modalities and Location
The general senses are distributed throughout the body, relying on nervous tissue incorporated into various organs. Somatic senses are incorporated mostly into the skin, muscles, or tendons, whereas the visceral senses come from nervous tissue incorporated into the majority of organs such as the heart or stomach. The somatic senses are those that usually make up the conscious perception of the how the body interacts with the environment. The visceral senses are most often below the limit of conscious perception because they are involved in homeostatic regulation through the autonomic nervous system.
The sensory exam tests the somatic senses, meaning those that are consciously perceived. Testing of the senses begins with examining the regions known as dermatomes that connect to the cortical region where somatosensation is perceived in the postcentral gyrus. To test the sensory fields, a simple stimulus of the light touch of the soft end of a cotton-tipped applicator is applied at various locations on the skin. The spinal nerves, which contain sensory fibers with dendritic endings in the skin, connect with the skin in a topographically organized manner, illustrated as dermatomes ( Figure 14.5.6 ). For example, the fibers of eighth cervical nerve innervate the medial surface of the forearm and extend out to the fingers. In addition to testing perception at different positions on the skin, it is necessary to test sensory perception within the dermatome from distal to proximal locations in the appendages, or lateral to medial locations in the trunk. In testing the eighth cervical nerve, the patient would be asked if the touch of the cotton to the fingers or the medial forearm was perceptible, and whether there were any differences in the sensations.
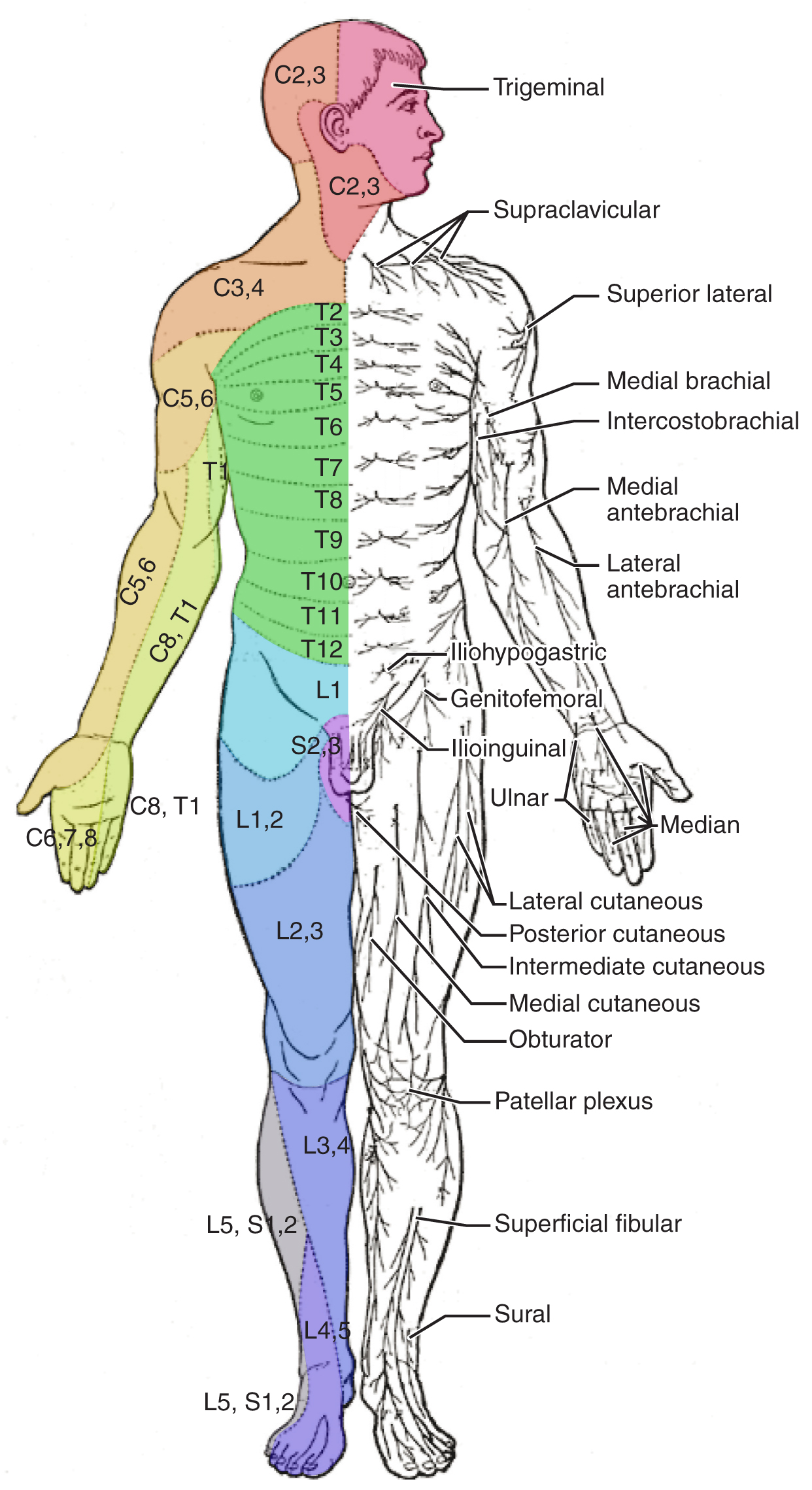
Other modalities of somatosensation can be tested using a few simple tools. The perception of pain can be tested using the broken end of the cotton-tipped applicator. The perception of vibratory stimuli can be testing using an oscillating tuning fork placed against prominent bone features such as the distal head of the ulna on the medial aspect of the elbow. When the tuning fork is still, the metal against the skin can be perceived as a cold stimulus. Using the cotton tip of the applicator, or even just a fingertip, the perception of tactile movement can be assessed as the stimulus is drawn across the skin for approximately 2–3 cm. The patient would be asked in what direction the stimulus is moving. All of these tests are repeated in distal and proximal locations and for different dermatomes to assess the spatial specificity of perception. The sense of position and motion, proprioception, is tested by moving the fingers or toes and asking the patient if they sense the movement. If the distal locations are not perceived, the test is repeated at increasingly proximal joints.
The various stimuli used to test sensory input assess the function of the major ascending tracts of the spinal cord. The dorsal column pathway conveys fine touch, vibration, and proprioceptive information, whereas the spinothalamic pathway primarily conveys pain and temperature. Testing these stimuli provides information about whether these two major ascending pathways are functioning properly. Within the spinal cord, the two systems are segregated. The dorsal column information ascends ipsilateral to the source of the stimulus and decussates in the medulla, whereas the spinothalamic pathway decussates at the level of entry and ascends contralaterally. The differing sensory stimuli are segregated in the spinal cord so that the various subtests for these stimuli can distinguish which ascending pathway may be damaged in certain situations.
Whereas the basic sensory stimuli are assessed in the subtests directed at each submodality of somatosensation, testing the ability to discriminate sensations is important. Pairing the light touch and pain subtests together makes it possible to compare the two submodalities at the same time, and therefore the two major ascending tracts at the same time. Mistaking painful stimuli for light touch, or vice versa, may point to errors in ascending projections, such as in a hemisection of the spinal cord that might come from a motor vehicle accident.
Another issue of sensory discrimination is not distinguishing between different submodalities, but rather location. The two-point discrimination subtest highlights the density of sensory endings, and therefore receptive fields in the skin. The sensitivity to fine touch, which can give indications of the texture and detailed shape of objects, is highest in the fingertips. To assess the limit of this sensitivity, two-point discrimination is measured by simultaneously touching the skin in two locations, such as could be accomplished with a pair of forceps. Specialized calipers for precisely measuring the distance between points are also available. The patient is asked to indicate whether one or two stimuli are present while keeping their eyes closed. The examiner will switch between using the two points and a single point as the stimulus. Failure to recognize two points may be an indication of a dorsal column pathway deficit.
Similar to two-point discrimination, but assessing laterality of perception, is double simultaneous stimulation. Two stimuli, such as the cotton tips of two applicators, are touched to the same position on both sides of the body. If one side is not perceived, this may indicate damage to the contralateral posterior parietal lobe. Because there is one of each pathway on either side of the spinal cord, they are not likely to interact. If none of the other subtests suggest particular deficits with the pathways, the deficit is likely to be in the cortex where conscious perception is based. The mental status exam contains subtests that assess other functions that are primarily localized to the parietal cortex, such as stereognosis and graphesthesia.
A final subtest of sensory perception that concentrates on the sense of proprioception is known as the Romberg test . The patient is asked to stand straight with feet together. Once the patient has achieved their balance in that position, they are asked to close their eyes. Without visual feedback that the body is in a vertical orientation relative to the surrounding environment, the patient must rely on the proprioceptive stimuli of joint and muscle position, as well as information from the inner ear, to maintain balance. This test can indicate deficits in dorsal column pathway proprioception, as well as problems with proprioceptive projections to the cerebellum through the spinocerebellar tract .

Watch this video to see a quick demonstration of two-point discrimination. Touching a specialized caliper to the surface of the skin will measure the distance between two points that are perceived as distinct stimuli versus a single stimulus. The patient keeps their eyes closed while the examiner switches between using both points of the caliper or just one. The patient then must indicate whether one or two stimuli are in contact with the skin. Why is the distance between the caliper points closer on the fingertips as opposed to the palm of the hand? And what do you think the distance would be on the arm, or the shoulder?
Muscle Strength and Voluntary Movement
The skeletomotor system is largely based on the simple, two-cell projection from the precentral gyrus of the frontal lobe to the skeletal muscles. The corticospinal tract represents the neurons that send output from the primary motor cortex. These fibers travel through the deep white matter of the cerebrum, then through the midbrain and pons, into the medulla where most of them decussate, and finally through the spinal cord white matter in the lateral (crossed fibers) or anterior (uncrossed fibers) columns. These fibers synapse on motor neurons in the ventral horn. The ventral horn motor neurons then project to skeletal muscle and cause contraction. These two cells are termed the upper motor neuron (UMN) and the lower motor neuron (LMN). Voluntary movements require these two cells to be active.
The motor exam tests the function of these neurons and the muscles they control. First, the muscles are inspected and palpated for signs of structural irregularities. Movement disorders may be the result of changes to the muscle tissue, such as scarring, and these possibilities need to be ruled out before testing function. Along with this inspection, muscle tone is assessed by moving the muscles through a passive range of motion. The arm is moved at the elbow and wrist, and the leg is moved at the knee and ankle. Skeletal muscle should have a resting tension representing a slight contraction of the fibers. The lack of muscle tone, known as hypotonicity or flaccidity , may indicate that the LMN is not conducting action potentials that will keep a basal level of acetylcholine in the neuromuscular junction.
If muscle tone is present, muscle strength is tested by having the patient contract muscles against resistance. The examiner will ask the patient to lift the arm, for example, while the examiner is pushing down on it. This is done for both limbs, including shrugging the shoulders. Lateral differences in strength—being able to push against resistance with the right arm but not the left—would indicate a deficit in one corticospinal tract versus the other. An overall loss of strength, without laterality, could indicate a global problem with the motor system. Diseases that result in UMN lesions include cerebral palsy or MS, or it may be the result of a stroke. A sign of UMN lesion is a negative result in the subtest for pronator drift . The patient is asked to extend both arms in front of the body with the palms facing up. While keeping the eyes closed, if the patient unconsciously allows one or the other arm to slowly relax, toward the pronated position, this could indicate a failure of the motor system to maintain the supinated position.
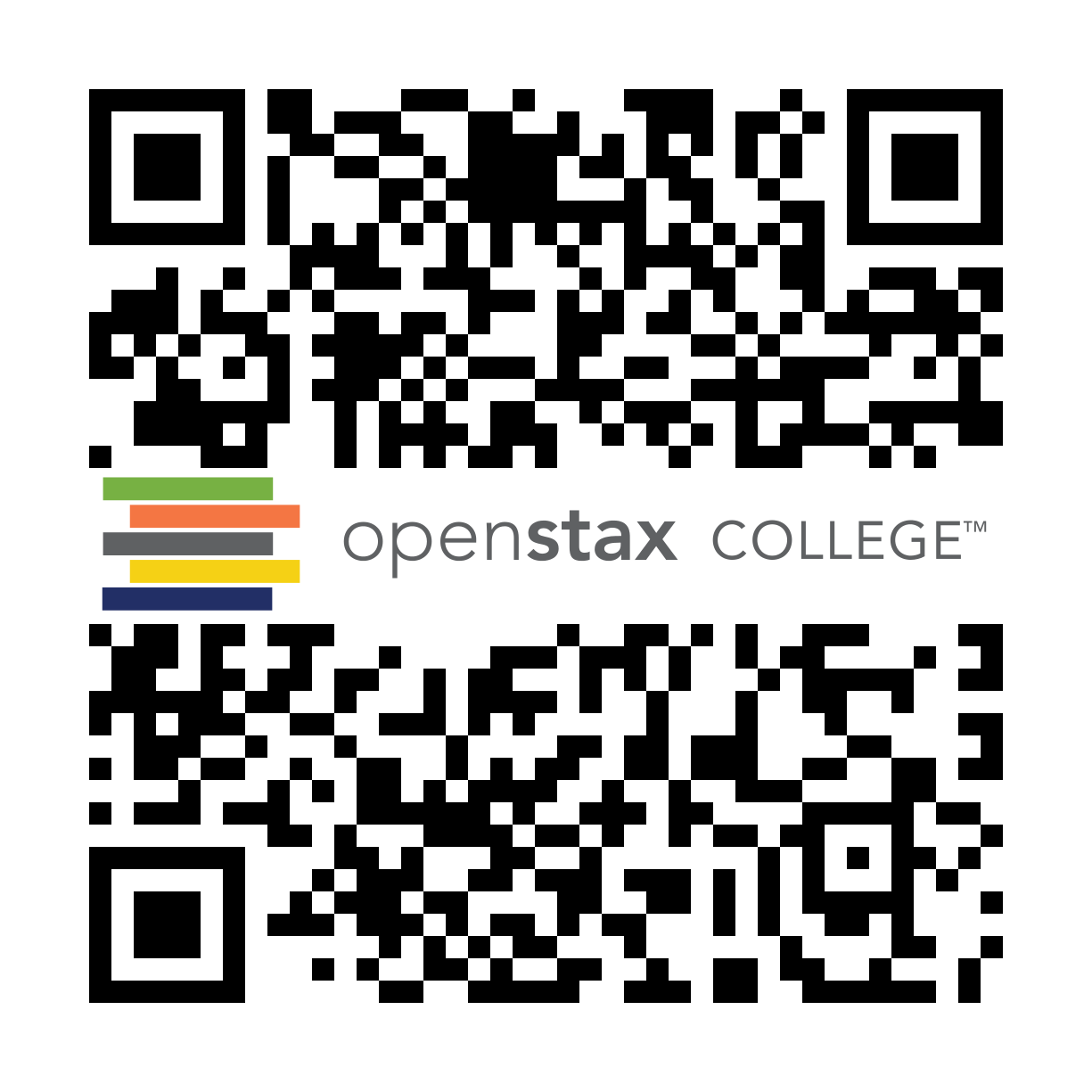
Watch this video to see how to test reflexes in the abdomen. Testing reflexes of the trunk is not commonly performed in the neurological exam, but if findings suggest a problem with the thoracic segments of the spinal cord, a series of superficial reflexes of the abdomen can localize function to those segments. If contraction is not observed when the skin lateral to the umbilicus (belly button) is stimulated, what level of the spinal cord may be damaged?
Comparison of Upper and Lower Motor Neuron Damage
Many of the tests of motor function can indicate differences that will address whether damage to the motor system is in the upper or lower motor neurons. Signs that suggest a UMN lesion include muscle weakness, strong deep tendon reflexes, decreased control of movement or slowness, pronator drift, a positive Babinski sign, spasticity , and the clasp-knife response . Spasticity is an excess contraction in resistance to stretch. It can result in hyperflexia , which is when joints are overly flexed. The clasp-knife response occurs when the patient initially resists movement, but then releases, and the joint will quickly flex like a pocket knife closing.
A lesion on the LMN would result in paralysis, or at least partial loss of voluntary muscle control, which is known as paresis . The paralysis observed in LMN diseases is referred to as flaccid paralysis , referring to a complete or partial loss of muscle tone, in contrast to the loss of control in UMN lesions in which tone is retained and spasticity is exhibited. Other signs of an LMN lesion are fibrillation , fasciculation , and compromised or lost reflexes resulting from the denervation of the muscle fibers.
Disorders of the…Spinal Cord
In certain situations, such as a motorcycle accident, only half of the spinal cord may be damaged in what is known as a hemisection. Forceful trauma to the trunk may cause ribs or vertebrae to fracture, and debris can crush or section through part of the spinal cord. The full section of a spinal cord would result in paraplegia, or loss of voluntary motor control of the lower body, as well as loss of sensations from that point down. A hemisection, however, will leave spinal cord tracts intact on one side. The resulting condition would be hemiplegia on the side of the trauma—one leg would be paralyzed. The sensory results are more complicated.
The ascending tracts in the spinal cord are segregated between the dorsal column and spinothalamic pathways. This means that the sensory deficits will be based on the particular sensory information each pathway conveys. Sensory discrimination between touch and painful stimuli will illustrate the difference in how these pathways divide these functions.
On the paralyzed leg, a patient will acknowledge painful stimuli, but not fine touch or proprioceptive sensations. On the functional leg, the opposite is true. The reason for this is that the dorsal column pathway ascends ipsilateral to the sensation, so it would be damaged the same way as the lateral corticospinal tract. The spinothalamic pathway decussates immediately upon entering the spinal cord and ascends contralateral to the source; it would therefore bypass the hemisection.
The motor system can indicate the loss of input to the ventral horn in the lumbar enlargement where motor neurons to the leg are found, but motor function in the trunk is less clear. The left and right anterior corticospinal tracts are directly adjacent to each other. The likelihood of trauma to the spinal cord resulting in a hemisection that affects one anterior column, but not the other, is very unlikely. Either the axial musculature will not be affected at all, or there will be bilateral losses in the trunk.
Sensory discrimination can pinpoint the level of damage in the spinal cord. Below the hemisection, pain stimuli will be perceived in the damaged side, but not fine touch. The opposite is true on the other side. The pain fibers on the side with motor function cross the midline in the spinal cord and ascend in the contralateral lateral column as far as the hemisection. The dorsal column will be intact ipsilateral to the source on the intact side and reach the brain for conscious perception. The trauma would be at the level just before sensory discrimination returns to normal, helping to pinpoint the trauma. Whereas imaging technology, like magnetic resonance imaging (MRI) or computed tomography (CT) scanning, could localize the injury as well, nothing more complicated than a cotton-tipped applicator can localize the damage. That may be all that is available on the scene when moving the victim requires crucial decisions be made.
The Coordination and Gait Exams
Location and connections of the cerebellum.
The cerebellum is located in apposition to the dorsal surface of the brain stem, centered on the pons. The name of the pons is derived from its connection to the cerebellum. The word means “bridge” and refers to the thick bundle of myelinated axons that form a bulge on its ventral surface. Those fibers are axons that project from the gray matter of the pons into the contralateral cerebellar cortex. These fibers make up the middle cerebellar peduncle (MCP) and are the major physical connection of the cerebellum to the brain stem ( Figure 14.5.7 ). Two other white matter bundles connect the cerebellum to the other regions of the brain stem. The superior cerebellar peduncle (SCP) is the connection of the cerebellum to the midbrain and forebrain. The inferior cerebellar peduncle (ICP) is the connection to the medulla.
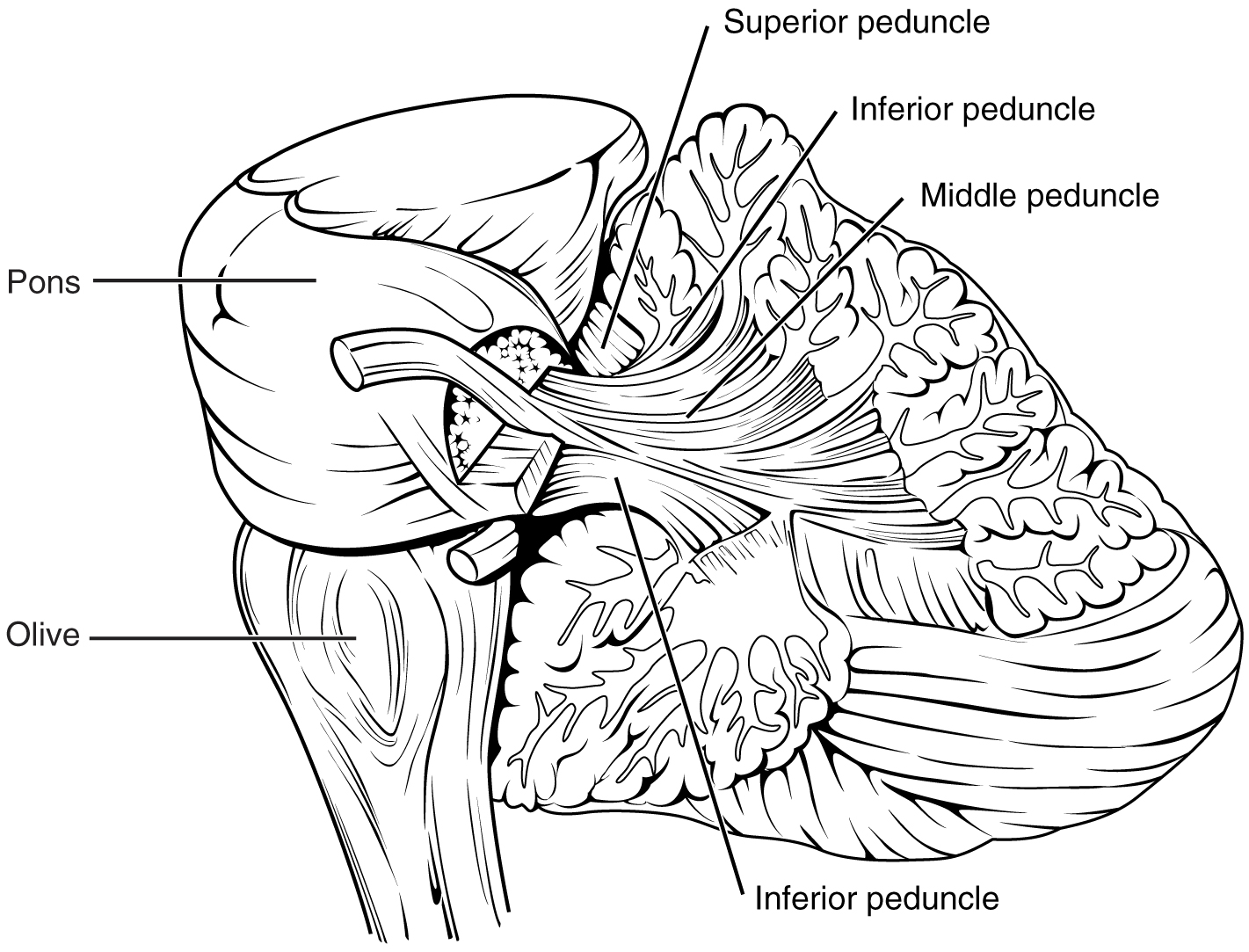
These connections can also be broadly described by their functions. The ICP conveys sensory input to the cerebellum, partially from the spinocerebellar tract, but also through fibers of the inferior olive . The MCP is part of the cortico-ponto-cerebellar pathway that connects the cerebral cortex with the cerebellum and preferentially targets the lateral regions of the cerebellum. It includes a copy of the motor commands sent from the precentral gyrus through the corticospinal tract, arising from collateral branches that synapse in the gray matter of the pons, along with input from other regions such as the visual cortex. The SCP is the major output of the cerebellum, divided between the red nucleus in the midbrain and the thalamus, which will return cerebellar processing to the motor cortex. These connections describe a circuit that compares motor commands and sensory feedback to generate a new output. These comparisons make it possible to coordinate movements. If the cerebral cortex sends a motor command to initiate walking, that command is copied by the pons and sent into the cerebellum through the MCP. Sensory feedback in the form of proprioception from the spinal cord, as well as vestibular sensations from the inner ear, enters through the ICP. If you take a step and begin to slip on the floor because it is wet, the output from the cerebellum—through the SCP—can correct for that and keep you balanced and moving. The red nucleus sends new motor commands to the spinal cord through the rubrospinal tract .
The cerebellum is divided into regions that are based on the particular functions and connections involved. The midline regions of the cerebellum, the vermis and flocculonodular lobe , are involved in comparing visual information, equilibrium, and proprioceptive feedback to maintain balance and coordinate movements such as walking, or gait , through the descending output of the red nucleus ( Figure 15.5.8 ). The lateral hemispheres are primarily concerned with planning motor functions through frontal lobe inputs that are returned through the thalamic projections back to the premotor and motor cortices. Processing in the midline regions targets movements of the axial musculature, whereas the lateral regions target movements of the appendicular musculature. The vermis is referred to as the spinocerebellum because it primarily receives input from the dorsal columns and spinocerebellar pathways. The flocculonodular lobe is referred to as the vestibulocerebellum because of the vestibular projection into that region. Finally, the lateral cerebellum is referred to as the cerebrocerebellum , reflecting the significant input from the cerebral cortex through the cortico-ponto-cerebellar pathway.
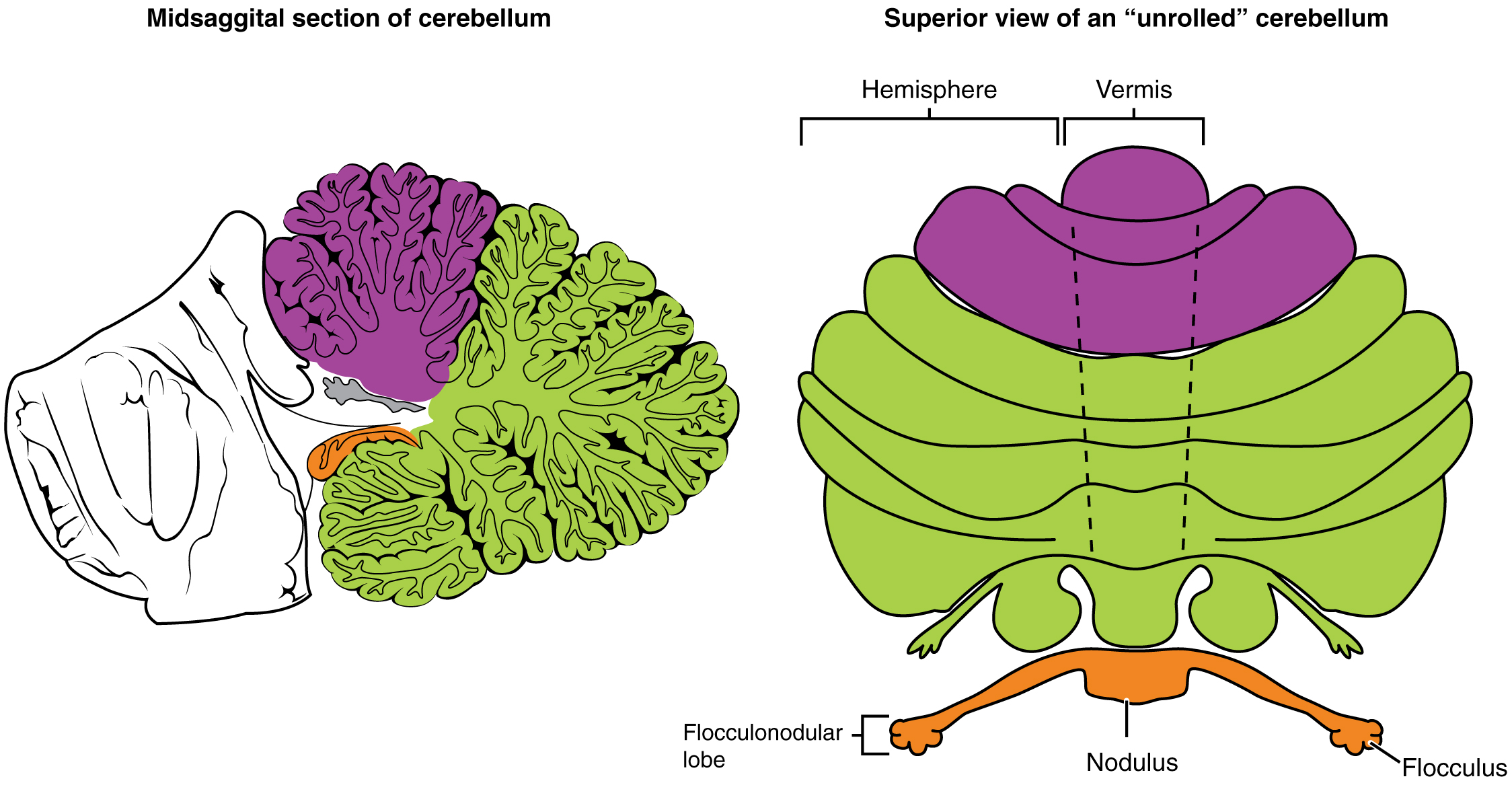
Coordination and Alternating Movement
Testing for cerebellar function is the basis of the coordination exam. The subtests target appendicular musculature, controlling the limbs, and axial musculature for posture and gait. The assessment of cerebellar function will depend on the normal functioning of other systems addressed in previous sections of the neurological exam. Motor control from the cerebrum, as well as sensory input from somatic, visual, and vestibular senses, are important to cerebellar function.
The subtests that address appendicular musculature, and therefore the lateral regions of the cerebellum, begin with a check for tremor. The patient extends their arms in front of them and holds the position. The examiner watches for the presence of tremors that would not be present if the muscles are relaxed. By pushing down on the arms in this position, the examiner can check for the rebound response, which is when the arms are automatically brought back to the extended position. The extension of the arms is an ongoing motor process, and the tap or push on the arms presents a change in the proprioceptive feedback. The cerebellum compares the cerebral motor command with the proprioceptive feedback and adjusts the descending input to correct. The red nucleus would send an additional signal to the LMN for the arm to increase contraction momentarily to overcome the change and regain the original position.
The check reflex depends on cerebellar input to keep increased contraction from continuing after the removal of resistance. The patient flexes the elbow against resistance from the examiner to extend the elbow. When the examiner releases the arm, the patient should be able to stop the increased contraction and keep the arm from moving. A similar response would be seen if you try to pick up a coffee mug that you believe to be full but turns out to be empty. Without checking the contraction, the mug would be thrown from the overexertion of the muscles expecting to lift a heavier object.
Several subtests of the cerebellum assess the ability to alternate movements, or switch between muscle groups that may be antagonistic to each other. In the finger-to-nose test, the patient touches their finger to the examiner’s finger and then to their nose, and then back to the examiner’s finger, and back to the nose. The examiner moves the target finger to assess a range of movements. A similar test for the lower extremities has the patient touch their toe to a moving target, such as the examiner’s finger. Both of these tests involve flexion and extension around a joint—the elbow or the knee and the shoulder or hip—as well as movements of the wrist and ankle. The patient must switch between the opposing muscles, like the biceps and triceps brachii, to move their finger from the target to their nose. Coordinating these movements involves the motor cortex communicating with the cerebellum through the pons and feedback through the thalamus to plan the movements. Visual cortex information is also part of the processing that occurs in the cerebrocerebellum while it is involved in guiding movements of the finger or toe.
Rapid, alternating movements are tested for the upper and lower extremities. The patient is asked to touch each finger to their thumb, or to pat the palm of one hand on the back of the other, and then flip that hand over and alternate back-and-forth. To test similar function in the lower extremities, the patient touches their heel to their shin near the knee and slides it down toward the ankle, and then back again, repetitively. Rapid, alternating movements are part of speech as well. A patient is asked to repeat the nonsense consonants “lah-kah-pah” to alternate movements of the tongue, lips, and palate. All of these rapid alternations require planning from the cerebrocerebellum to coordinate movement commands that control the coordination.
Posture and Gait
Gait can either be considered a separate part of the neurological exam or a subtest of the coordination exam that addresses walking and balance. Testing posture and gait addresses functions of the spinocerebellum and the vestibulocerebellum because both are part of these activities. A subtest called station begins with the patient standing in a normal position to check for the placement of the feet and balance. The patient is asked to hop on one foot to assess the ability to maintain balance and posture during movement. Though the station subtest appears to be similar to the Romberg test, the difference is that the patient’s eyes are open during station. The Romberg test has the patient stand still with the eyes closed. Any changes in posture would be the result of proprioceptive deficits, and the patient is able to recover when they open their eyes.
Subtests of walking begin with having the patient walk normally for a distance away from the examiner, and then turn and return to the starting position. The examiner watches for abnormal placement of the feet and the movement of the arms relative to the movement. The patient is then asked to walk with a few different variations. Tandem gait is when the patient places the heel of one foot against the toe of the other foot and walks in a straight line in that manner. Walking only on the heels or only on the toes will test additional aspects of balance.
A movement disorder of the cerebellum is referred to as ataxia . It presents as a loss of coordination in voluntary movements. Ataxia can also refer to sensory deficits that cause balance problems, primarily in proprioception and equilibrium. When the problem is observed in movement, it is ascribed to cerebellar damage. Sensory and vestibular ataxia would likely also present with problems in gait and station.
Ataxia is often the result of exposure to exogenous substances, focal lesions, or a genetic disorder. Focal lesions include strokes affecting the cerebellar arteries, tumors that may impinge on the cerebellum, trauma to the back of the head and neck, or MS. Alcohol intoxication or drugs such as ketamine cause ataxia, but it is often reversible. Mercury in fish can cause ataxia as well. Hereditary conditions can lead to degeneration of the cerebellum or spinal cord, as well as malformation of the brain, or the abnormal accumulation of copper seen in Wilson’s disease.
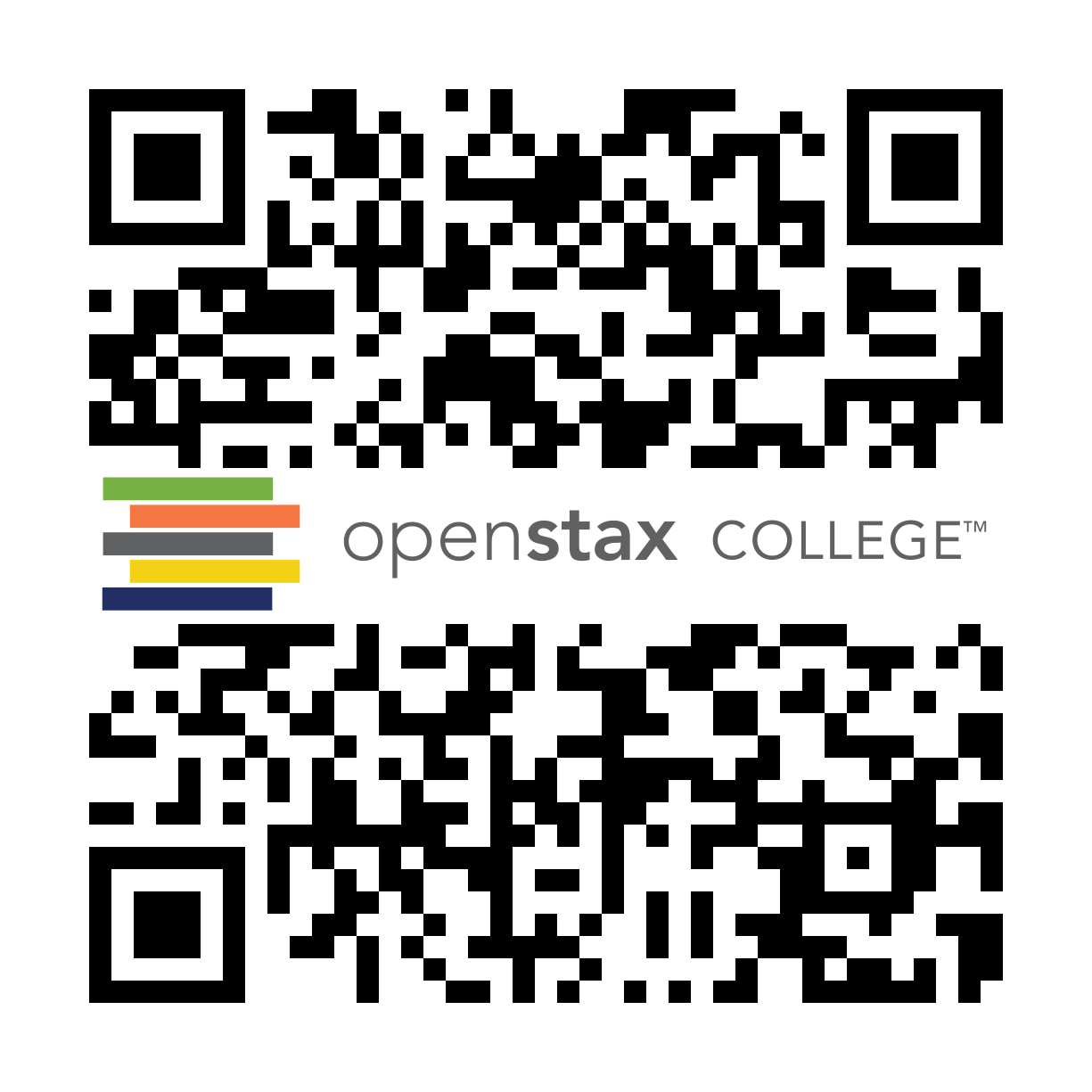
Watch this short video to see a test for station. Station refers to the position a person adopts when they are standing still. The examiner would look for issues with balance, which coordinates proprioceptive, vestibular, and visual information in the cerebellum. To test the ability of a subject to maintain balance, asking them to stand or hop on one foot can be more demanding. The examiner may also push the subject to see if they can maintain balance. An abnormal finding in the test of station is if the feet are placed far apart. Why would a wide stance suggest problems with cerebellar function?
Everyday Connections – The Field Sobriety Test
The neurological exam has been described as a clinical tool throughout this chapter. It is also useful in other ways. A variation of the coordination exam is the Field Sobriety Test (FST) used to assess whether drivers are under the influence of alcohol. The cerebellum is crucial for coordinated movements such as keeping balance while walking, or moving appendicular musculature on the basis of proprioceptive feedback. The cerebellum is also very sensitive to ethanol, the particular type of alcohol found in beer, wine, and liquor.
Walking in a straight line involves comparing the motor command from the primary motor cortex to the proprioceptive and vestibular sensory feedback, as well as following the visual guide of the white line on the side of the road. When the cerebellum is compromised by alcohol, the cerebellum cannot coordinate these movements effectively, and maintaining balance becomes difficult.
Another common aspect of the FST is to have the driver extend their arms out wide and touch their fingertip to their nose, usually with their eyes closed. The point of this is to remove the visual feedback for the movement and force the driver to rely just on proprioceptive information about the movement and position of their fingertip relative to their nose. With eyes open, the corrections to the movement of the arm might be so small as to be hard to see, but proprioceptive feedback is not as immediate and broader movements of the arm will probably be needed, particularly if the cerebellum is affected by alcohol.
Reciting the alphabet backwards is not always a component of the FST, but its relationship to neurological function is interesting. There is a cognitive aspect to remembering how the alphabet goes and how to recite it backwards. That is actually a variation of the mental status subtest of repeating the months backwards. However, the cerebellum is important because speech production is a coordinated activity. The speech rapid alternating movement subtest is specifically using the consonant changes of “lah-kah-pah” to assess coordinated movements of the lips, tongue, pharynx, and palate. But the entire alphabet, especially in the nonrehearsed backwards order, pushes this type of coordinated movement quite far. It is related to the reason that speech becomes slurred when a person is intoxicated.
Chapter Review
Sensory input to the brain enters through pathways that travel through either the spinal cord (for somatosensory input from the body) or the brain stem (for everything else, except the visual and olfactory systems) to reach the diencephalon. In the diencephalon, sensory pathways reach the thalamus. This is necessary for all sensory systems to reach the cerebral cortex, except for the olfactory system that is directly connected to the frontal and temporal lobes.
The two major tracts in the spinal cord, originating from sensory neurons in the dorsal root ganglia, are the dorsal column system and the spinothalamic tract. The major differences between the two are in the type of information that is relayed to the brain and where the tracts decussate. The dorsal column system primarily carries information about touch and proprioception and crosses the midline in the medulla. The spinothalamic tract is primarily responsible for pain and temperature sensation and crosses the midline in the spinal cord at the level at which it enters. The trigeminal nerve adds similar sensation information from the head to these pathways.
The motor components of the somatic nervous system begin with the frontal lobe of the brain, where the prefrontal cortex is responsible for higher functions such as working memory. The integrative and associate functions of the prefrontal lobe feed into the secondary motor areas, which help plan movements. The premotor cortex and supplemental motor area then feed into the primary motor cortex that initiates movements. Large Betz cells project through the corticobulbar and corticospinal tracts to synapse on lower motor neurons in the brain stem and ventral horn of the spinal cord, respectively. These connections are responsible for generating movements of skeletal muscles.
The extrapyramidal system includes projections from the brain stem and higher centers that influence movement, mostly to maintain balance and posture, as well as to maintain muscle tone. The superior colliculus and red nucleus in the midbrain, the vestibular nuclei in the medulla, and the reticular formation throughout the brain stem each have tracts projecting to the spinal cord in this system. Descending input from the secondary motor cortices, basal nuclei, and cerebellum connect to the origins of these tracts in the brain stem.
All of these motor pathways project to the spinal cord to synapse with motor neurons in the ventral horn of the spinal cord. These lower motor neurons are the cells that connect to skeletal muscle and cause contractions. These neurons project through the spinal nerves to connect to the muscles at neuromuscular junctions. One motor neuron connects to multiple muscle fibers within a target muscle. The number of fibers that are innervated by a single motor neuron varies on the basis of the precision necessary for that muscle and the amount of force necessary for that motor unit. The quadriceps, for example, have many fibers controlled by single motor neurons for powerful contractions that do not need to be precise. The extraocular muscles have only a small number of fibers controlled by each motor neuron because moving the eyes does not require much force, but needs to be very precise.
Reflexes are the simplest circuits within the somatic nervous system. A withdrawal reflex from a painful stimulus only requires the sensory fiber that enters the spinal cord and the motor neuron that projects to a muscle. Antagonist and postural muscles can be coordinated with the withdrawal, making the connections more complex. The simple, single neuronal connection is the basis of somatic reflexes. The corneal reflex is contraction of the orbicularis oculi muscle to blink the eyelid when something touches the surface of the eye. Stretch reflexes maintain a constant length of muscles by causing a contraction of a muscle to compensate for a stretch that can be sensed by a specialized receptor called a muscle spindle.
Interactive Link Questions
The video only describes the lateral division of the corticospinal tract. The anterior division is omitted.
The movement disorders were similar to those seen in movement disorders of the extrapyramidal system, which would mean the basal nuclei are the most likely source of haloperidol side effects. In fact, haloperidol affects dopamine activity, which is a prominent part of the chemistry of the basal nuclei.
Watch this video to learn more about the reflex arc of the corneal reflex. When the right cornea senses a tactile stimulus, what happens to the left eye? Explain your answer.
The left eye also blinks. The sensory input from one eye activates the motor response of both eyes so that they both blink.
Watch this video to learn more about newborn reflexes. Newborns have a set of reflexes that are expected to have been crucial to survival before the modern age. These reflexes disappear as the baby grows, as some of them may be unnecessary as they age. The video demonstrates a reflex called the Babinski reflex, in which the foot flexes dorsally and the toes splay out when the sole of the foot is lightly scratched. This is normal for newborns, but it is a sign of reduced myelination of the spinal tract in adults. Why would this reflex be a problem for an adult?
While walking, the sole of the foot may be scraped or scratched by many things. If the foot still reacted as in the Babinski reflex, an adult might lose their balance while walking.
Glossary (sensory)
Glossary (motor).
This work, Anatomy & Physiology, is adapted from Anatomy & Physiology by OpenStax , licensed under CC BY . This edition, with revised content and artwork, is licensed under CC BY-SA except where otherwise noted.
Images, from Anatomy & Physiology by OpenStax , are licensed under CC BY except where otherwise noted.
Access the original for free at https://openstax.org/books/anatomy-and-physiology/pages/1-introduction .
Anatomy & Physiology Copyright © 2019 by Lindsay M. Biga, Staci Bronson, Sierra Dawson, Amy Harwell, Robin Hopkins, Joel Kaufmann, Mike LeMaster, Philip Matern, Katie Morrison-Graham, Kristen Oja, Devon Quick, Jon Runyeon, OSU OERU, and OpenStax is licensed under a Creative Commons Attribution-ShareAlike 4.0 International License , except where otherwise noted.
Section 6: The Central Nervous System
Introduction to sensory and motor pathways.
Having looked at the components of nervous tissue, and the basic anatomy of the nervous system, next comes an understanding of how nervous tissue is capable of communicating within the nervous system. Before getting to the nuts and bolts of how this works, an illustration of how the components come together will be helpful.
Imagine you are about to take a shower in the morning before going to school. You have turned on the faucet to start the water as you prepare to get in the shower. After a few minutes, you expect the water to be a temperature that will be comfortable to enter. So you put your hand out into the spray of water. What happens next depends on how your nervous system interacts with the stimulus of the water temperature and what you do in response to that stimulus. Figure 1 shows one possible path you may follow.
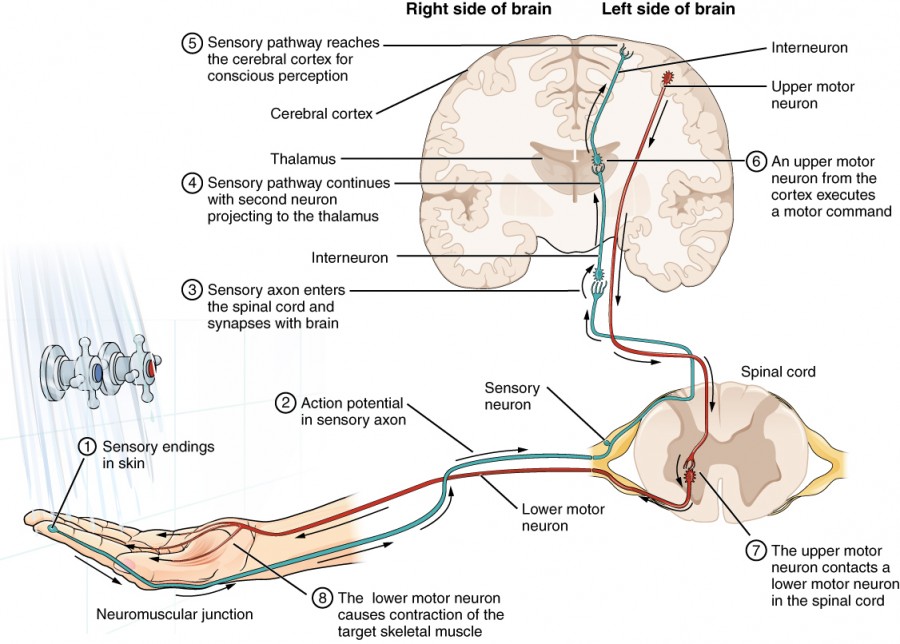
Figure 1. Testing the Water
Here’s a more detailed breakdown of the processes found in Figure 1:
- The sensory neuron has endings in the skin that sense a stimulus such as water temperature. The strength of the signal that starts here is dependent on the strength of the stimulus.
- The graded potential from the sensory endings, if strong enough, will initiate an action potential at the initial segment of the axon (which is immediately adjacent to the sensory endings in the skin).
- The axon of the peripheral sensory neuron enters the spinal cord and contacts another neuron in the gray matter. The contact is a synapse where another graded potential is caused by the release of a chemical signal from the axon terminals.
- An action potential is initiated at the initial segment of this neuron and travels up the sensory pathway to a region of the brain called the thalamus. Another synapse passes the information along to the next neuron.
- The sensory pathway ends when the signal reaches the cerebral cortex.
- After integration with neurons in other parts of the cerebral cortex, a motor command is sent from the precentral gyrus of the frontal cortex.
- The upper motor neuron sends an action potential down to the spinal cord. The target of the upper motor neuron is the dendrites of the lower motor neuron in the gray matter of the spinal cord.
- The axon of the lower motor neuron emerges from the spinal cord in a nerve and connects to a muscle through a neuromuscular junction to cause contraction of the target muscle.
Found in the skin of your fingers or toes is a type of sensory receptor that is sensitive to temperature, called a thermoreceptor . When you place your hand under the shower (Figure 2), the cell membrane of the thermoreceptors changes its electrical state (voltage).
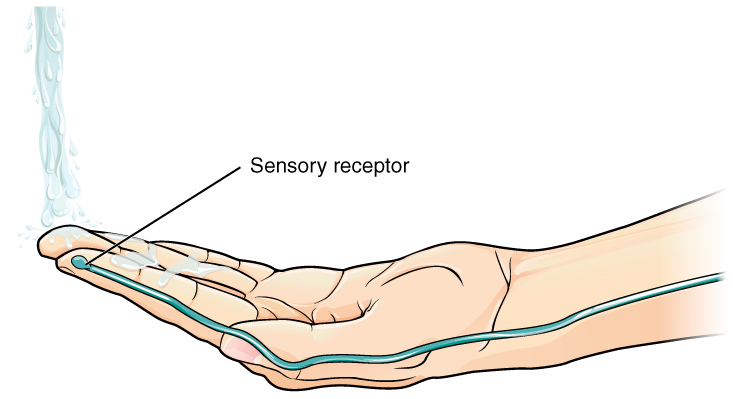
Figure 2. The Sensory Input Receptors in the skin sense the temperature of the water.
The amount of change is dependent on the strength of the stimulus (how hot the water is). This is called a graded potential . If the stimulus is strong, the voltage of the cell membrane will change enough to generate an electrical signal that will travel down the axon. You have learned about this type of signaling before, with respect to the interaction of nerves and muscles at the neuromuscular junction. The voltage at which such a signal is generated is called the threshold , and the resulting electrical signal is called an action potential . In this example, the action potential travels—a process known as propagation —along the axon from the axon hillock to the axon terminals and into the synaptic end bulbs. When this signal reaches the end bulbs, it causes the release of a signaling molecule called a neurotransmitter .
The neurotransmitter diffuses across the short distance of the synapse and binds to a receptor protein of the target neuron. When the molecular signal binds to the receptor, the cell membrane of the target neuron changes its electrical state and a new graded potential begins. If that graded potential is strong enough to reach threshold, the second neuron generates an action potential at its axon hillock. The target of this neuron is another neuron in the thalamus of the brain, the part of the CNS that acts as a relay for sensory information. At another synapse, neurotransmitter is released and binds to its receptor. The thalamus then sends the sensory information to the cerebral cortex , the outermost layer of gray matter in the brain, where conscious perception of that water temperature begins.
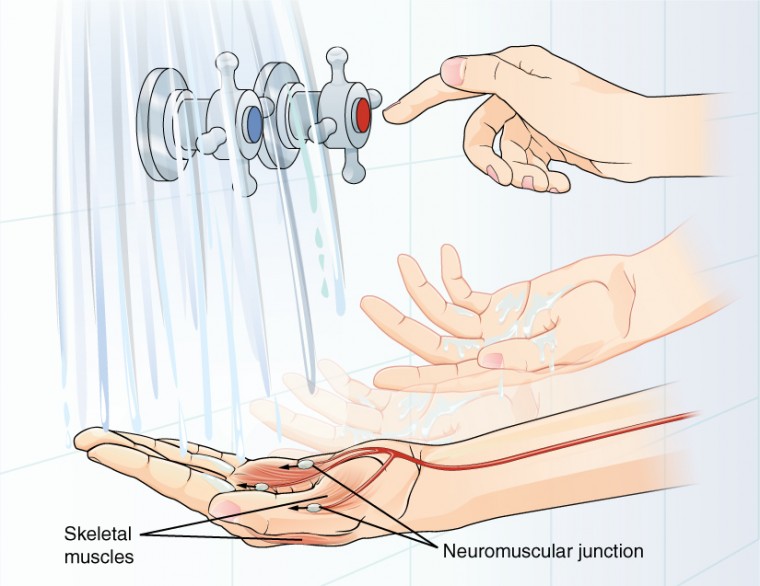
Figure 3. The Motor Response On the basis of the sensory input and the integration in the CNS, a motor response is formulated and executed.
Within the cerebral cortex, information is processed among many neurons, integrating the stimulus of the water temperature with other sensory stimuli, with your emotional state (you just aren’t ready to wake up; the bed is calling to you), memories (perhaps of the lab notes you have to study before a quiz). Finally, a plan is developed about what to do, whether that is to turn the temperature up, turn the whole shower off and go back to bed, or step into the shower. To do any of these things, the cerebral cortex has to send a command out to your body to move muscles (Figure 3).
A region of the cortex is specialized for sending signals down to the spinal cord for movement. The upper motor neuron is in this region, called the precentral gyrus of the frontal cortex , which has an axon that extends all the way down the spinal cord. At the level of the spinal cord at which this axon makes a synapse, a graded potential occurs in the cell membrane of a lower motor neuron . This second motor neuron is responsible for causing muscle fibers to contract. In the manner described in the chapter on muscle tissue, an action potential travels along the motor neuron axon into the periphery. The axon terminates on muscle fibers at the neuromuscular junction. Acetylcholine is released at this specialized synapse, which causes the muscle action potential to begin, following a large potential known as an end plate potential. When the lower motor neuron excites the muscle fiber, it contracts. All of this occurs in a fraction of a second, but this story is the basis of how the nervous system functions.
Career Connections: Neurophysiologist
Understanding how the nervous system works could be a driving force in your career. Studying neurophysiology is a very rewarding path to follow. It means that there is a lot of work to do, but the rewards are worth the effort.
The career path of a research scientist can be straightforward: college, graduate school, postdoctoral research, academic research position at a university. A Bachelor’s degree in science will get you started, and for neurophysiology that might be in biology, psychology, computer science, engineering, or neuroscience. But the real specialization comes in graduate school. There are many different programs out there to study the nervous system, not just neuroscience itself. Most graduate programs are doctoral, meaning that a Master’s degree is not part of the work. These are usually considered five-year programs, with the first two years dedicated to course work and finding a research mentor, and the last three years dedicated to finding a research topic and pursuing that with a near single-mindedness. The research will usually result in a few publications in scientific journals, which will make up the bulk of a doctoral dissertation. After graduating with a Ph.D., researchers will go on to find specialized work called a postdoctoral fellowship within established labs. In this position, a researcher starts to establish their own research career with the hopes of finding an academic position at a research university.
Other options are available if you are interested in how the nervous system works. Especially for neurophysiology, a medical degree might be more suitable so you can learn about the clinical applications of neurophysiology and possibly work with human subjects. An academic career is not a necessity. Biotechnology firms are eager to find motivated scientists ready to tackle the tough questions about how the nervous system works so that therapeutic chemicals can be tested on some of the most challenging disorders such as Alzheimer’s disease or Parkinson’s disease, or spinal cord injury.
Others with a medical degree and a specialization in neuroscience go on to work directly with patients, diagnosing and treating mental disorders. You can do this as a psychiatrist, a neuropsychologist, a neuroscience nurse, or a neurodiagnostic technician, among other possible career paths.
- Anatomy & Physiology. Authored by : OpenStax College. Provided by : Rice University. Located at : http://cnx.org/contents/[email protected] . License : CC BY: Attribution . License Terms : Download for free at http://cnx.org/contents/[email protected]

Tips and real-world stories
to live your life to the fullest with limited mobility

The Spinal Cord: Three Types of Signals That It Sends
- Health & Assessment
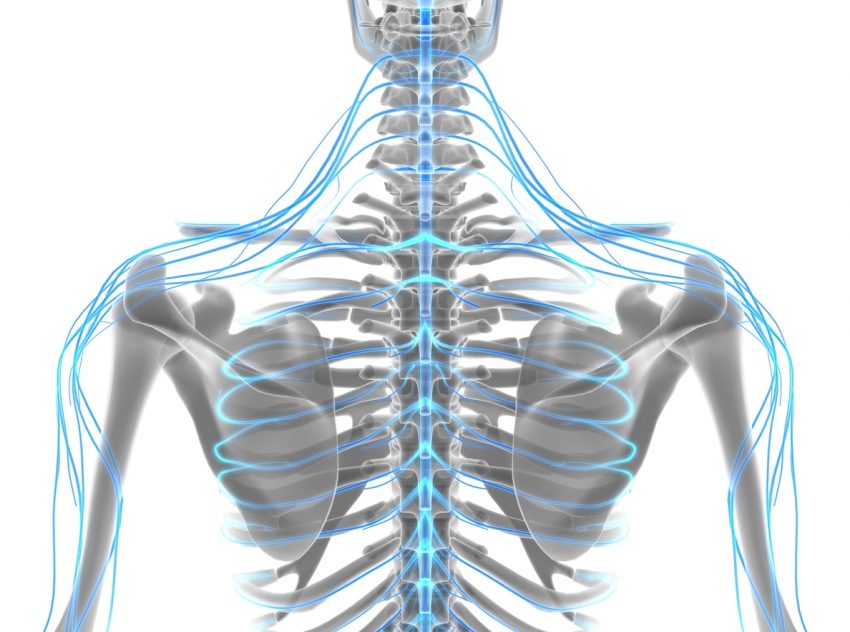
There are three types of signals that are carried from your body to your brain through your spinal cord. The three signals are:
- Sensory- signals that evoke feelings like temperature, touch, pain, and pressure
- Motor- signals that cause voluntary movements
- Reflex- signals that cause involuntary movements.
The different types of signals are sent out and received in different ways.
Spinal Cord Signals: Sensory
Sensory signals alert us to feelings both inside and outside the body. These feelings include temperature, touch, pain, and pressure. These signals tell us when we are hot or cold, injured or safe. The nerves in the body send signals along their pathway to the spinal cord, then to the brain. Once the signals reach your brain, your body registers the sensation, and you are able to feel it. Sensory signals travel so fast that it seems like you get the message instantaneously.
Another thing that sensory signals do is they tell your brain what your body position is in space. That means it tells you if you are standing, sitting, leaning, etc. They also tell you where your body parts are in relation to the rest of your body. They are the reason you can reach out with your arm and move the right distance to grab something without going too far or coming up short.

Spinal Cord Signals: Motor
Motor signals cause voluntary movement. They tell your muscles to move when you want them to. The signals also tell your muscles specifically when and how to move. These signals are not like sensory signals. These signals don’t begin at the nerve endings; they begin inside the brain. From there, they travel through the spinal cord, out to the spinal nerves, and then on to the parts of the body.
These signals also occur so quickly that you feel like your body gets the message instantaneously. In fact, you don’t even realize you are consciously choosing to send the message to your body to move. An example of this would be when you reach out to hug someone.
Spinal Cord Signals: Reflex
Reflex signals cause involuntary movements. This means that the movement was not conscious. You did not decide to make it. A muscle spasm is a good example of this type of movement. Reflex signals that cause movement do not come from your brain.
A reflex signal comes from the nerves in your body, like sensory signals, but instead of going to your brain, they stop at the spinal cord . Once they reach the spinal cord, they loop through and go straight back to the body part they came from. Reflex signals are designed to protect your body. This is why they happen so quickly. They do not have to wait to reach the brain and then wait for the brain to choose a reaction.
Reflex signals are initiated when the nerves in a muscle are irritated by being stretched or pushed on. This triggers the nerves to send a message to the spinal cord . When the signal reaches the spinal cord, it goes back through at the same level it came in, returning to the muscle that initiated the signal. Once it gets back to the starting point, the signal causes the muscle to react by squeezing or contracting.
Spinal Cord Damage
When the spinal cord is damaged, it prevents these signals from being sent correctly. That is why a spinal cord injury causes loss of movement, sensation, and reflexes. The areas affected are the parts of the body that connects to the spinal cord at the level of the injury or lower. Some people have partial injury, where they still have nerves that are working below the injury, and some people have complete injury. Sometimes people even lose voluntary motion in a body part but still retain reflex motion.
Every injury is unique. It is good to talk to your doctor about your function to determine what types of signals your body has the capability of sending and receiving. Then you can work to optimize your movement to the best of your ability.
Author: Annie Beth Donahue is a professional writer with a health and disability focus.
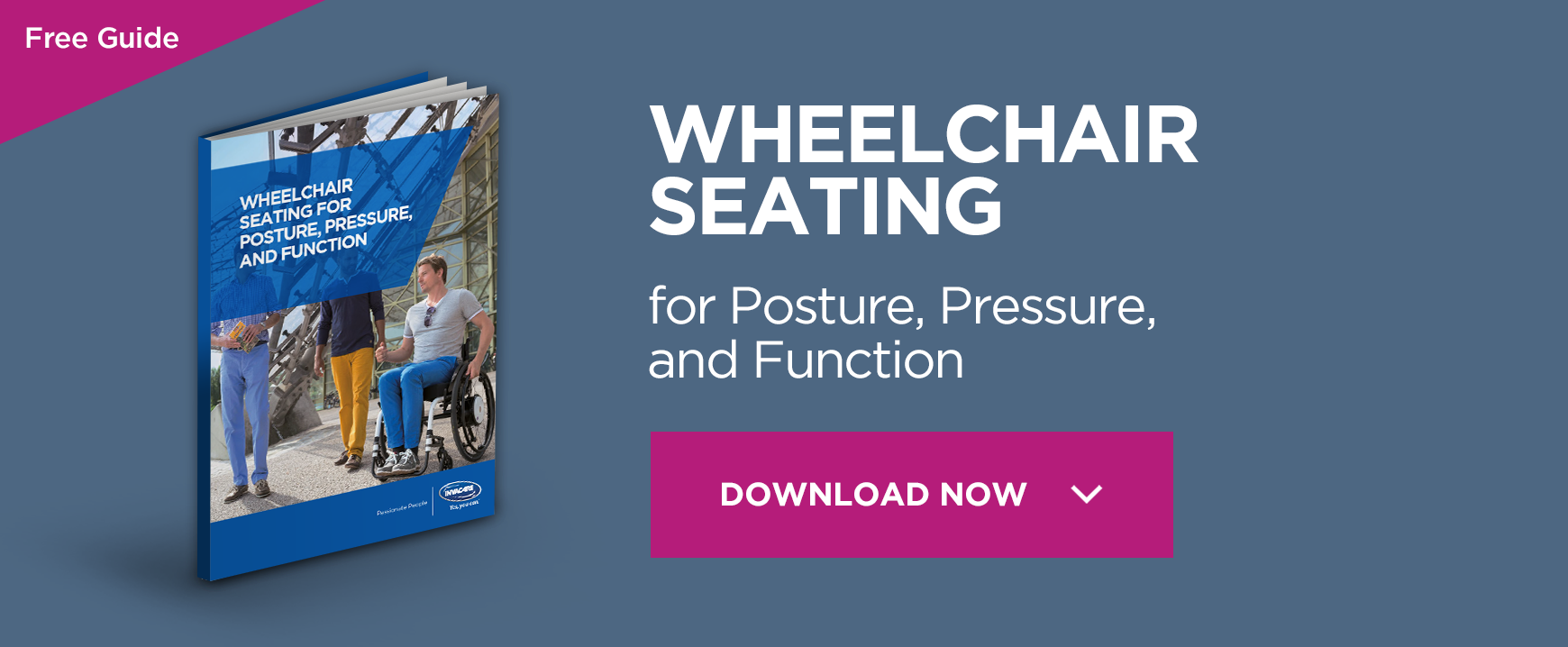
Keep reading
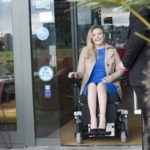
How does the brain transfer signals to each body part to move? Yulissa, 11, Virginia
Dear Yulissa,
Your brain weighs less than 3 pounds but has the power to move your whole body. That’s because it’s part of your nervous system .
Your brain and the spinal cord that runs down your back make up your central nervous system. You also have a peripheral nervous system made up of nerve cells. These connect your brain and spinal cord to all the other parts of your body.
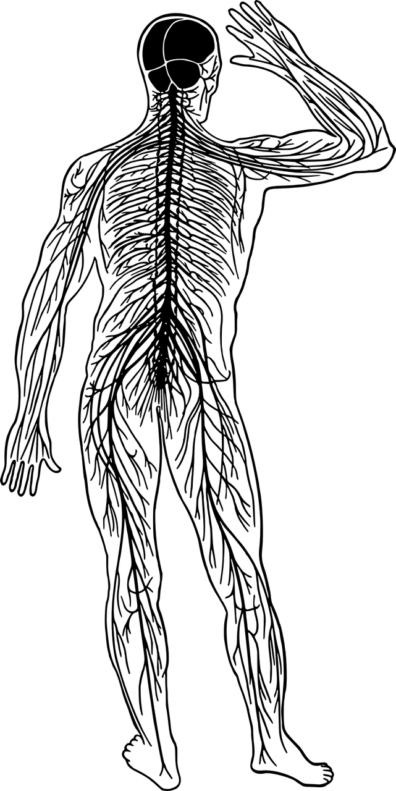
I talked about how your brain signals your body to move with my friend Samantha Gizerian , professor of Integrative Physiology and Neuroscience at Washington State University.
She told me that the brain processes movement in three steps.
First, your senses tell your brain what’s going on around you and what position your body is in. Next, your brain uses that sensory input to plan how to move. Then, your brain sends signals that tell your muscles to contract.
One part of the brain that’s involved in movement is the motor cortex .
“It sits right before your ears but up at the top,” Gizerian said. “It’s the part that sends the signals down to your muscles to tell them to move. But it also has a map. So, there’s a particular part of motor cortex that moves your head. There’s another part that moves your hands and your fingers. There’s another part that moves your arms, your legs, your feet.”
The map is called your homunculus . That means “little man” because the map can be drawn to look like a person with huge hands. The size of the body part in the drawing shows how much of your motor cortex is dedicated to moving that part of your body.
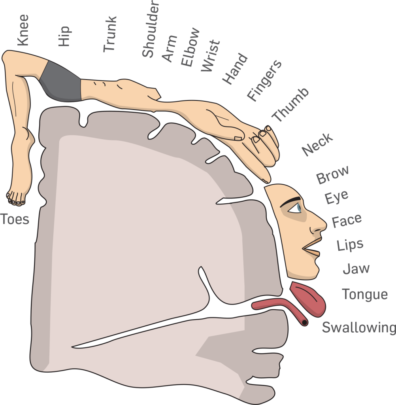
So, let’s say you want to write. How does the motor cortex tell your hand to move?
“The part of the motor cortex that’s responsible for the parts of the hand that will be moving fires a signal,” Gizerian said. “Those signals travel down through the brain stem and spinal cord and pass that signal on to a neuron that goes out to the muscles. Then the muscles contract.”
Neurons are nerves cells. The ones that come from your brain and spinal cord out to your muscles are called motor neurons—because they motor you around. You have about 500,000 motor neurons in your body!
Neurons use electrical and chemical signals to tell your muscles to contract or relax. That makes your muscles pull on your bones, which is how your body moves.
To make sure your movements are smooth, another part of your brain steps in. The cerebellum is the bump at the back of your brain. The signals your motor cortex sends to your muscles are copied there. It helps correct your motion and provides muscle memory.
An activity like writing is what neuroscientists call a motor program. When you run a motor program over and over, your brain gets lots of feedback from your joints, skin and muscles. It uses that feedback to make tiny corrections in your motor program. Eventually, writing is easy and beautiful.
It’s all thanks to your brain .
Dr. Universe
Electromyography (EMG) and Nerve Conduction Studies
- What are EMG and nerve conduction studies? |
- Why would I need an EMG or nerve conduction study? |
- What happens during an EMG or nerve conduction study? |
- What are the problems with an EMG or nerve conduction study? |
Your brain tells your muscles what to do by sending electrical signals to them. The signals travel down your spinal cord and then through different nerves to your muscles.
What are EMG and nerve conduction studies?
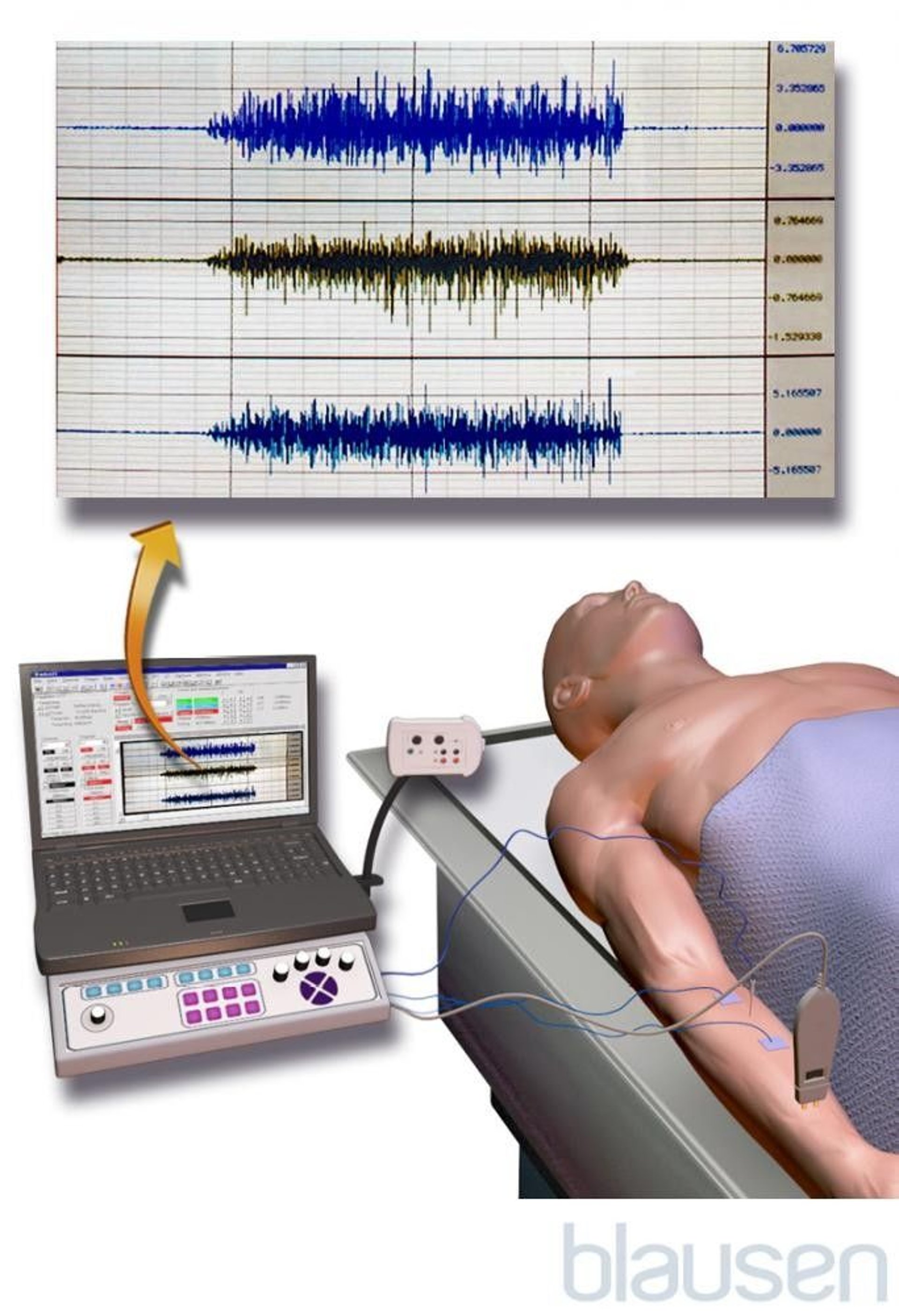
EMG and nerve conduction studies are tests to see if you have muscle weakness or loss of feeling from an injury to your spinal cord, muscles, or nerves.
To do an EMG, doctors insert small needles into a muscle to record your muscle’s electrical activity when it’s resting and when it’s active
To do nerve conduction studies, doctors use skin sensors or needles to send small electric shocks through different nerves to see how well your nerves work
Why would I need an EMG or nerve conduction study?
Doctors may do an EMG, nerve conduction study, or both if you have symptoms like tingling or muscle weakness. These symptoms can be caused by many problems in different parts of your body. An EMG or nerve conduction study can help your doctor tell if your symptoms are caused by nerve or muscle problems such as:
Muscular dystrophy
Carpal tunnel syndrome
Amyotrophic lateral sclerosis (ALS)
What happens during an EMG or nerve conduction study?
During an EMG:
Doctors put needles into your muscle
The needles are connected by wires to a machine that records your muscle's electrical activity while you move it and relax it
During a nerve conduction study:
Doctors put a sticky sensor on your skin over the nerve they are testing
They stimulate another part of the nerve with a small electric shock
A machine measures how fast the electrical signal travels down the nerve
What are the problems with an EMG or nerve conduction study?
The needles and electric shocks can be uncomfortable or hurt. You may have some bruises afterward.

- Cookie Preferences

Copyright © 2024 Merck & Co., Inc., Rahway, NJ, USA and its affiliates. All rights reserved.
Boost Your Brain's Performance
Browse our selection of books, supplements, and natural remedies to maximize your brain's health and performance potential, the spinal cord.
Table of Contents
The spinal cord is essentially the headquarters of the peripheral nervous system (PNS). It is responsible for the transmission of information between the PNS and the central nervous system (CNS). Curiously, with the spinal cord alone, many autonomic functions and even voluntary movements can occur. Not all actions of the body necessarily need the brain to be carried out! Within the spinal cord are nerve tracts that carry sensory information to regulate motor control. These nerve tracts even play a role in consciousness and awareness. Here, we’ll take a look at the anatomy and functions that make up the purpose of the spinal cord.
Neuronal Pathways in the Spinal Cord
There are many different collections of nerve tracts that run up and down the spinal cord, inputting information to the thalamus, cerebral cortex, and cerebellum. These tracts are responsible for the transmission of sensory information as it relates to pain, temperature, touch, pressure, proprioception, and more. Once the action potentials, or neuronal transmissions, reached their ultimate destination in the brain, the signal can then be interpreted to create and execute a motor plan.
For example, let’s look at the pathway for pain and temperature: starting at the bottom of the spinal cord (or particular vertebral level, depending on where sensory information is being transmitted from), sensory neurons transmit neuronal information into the spinal cord at the point of decussation.
(Decussation is the crossing over of nerves from one side of the spinal cord’s midline to the other. The particular bundles of nerve fibers that do this crossing are called commissures.) The information must first have synapsed onto an interneuron in order to enter the spinal cord and decussate.
(A quick distinction: An interneuron is known as such because the whole of its anatomical structure – cell body and axon – is located within the CNS, in the spinal cord and/or brain. On the other hand, a ganglion is a collection of neuron cell bodies outside of the CNS. Their axons then travel into the CNS for transmitting neuronal signals.)

The masses of interneurons within the spinal cord are what make up the gray matter, the butterfly shape in the center of the spinal cord – remember that this gray matter is made up of cell bodies. The white matter surrounding the butterfly is a mass of myelinated nerve fibers.
After the information is passed through a synapse in either the dorsal or ventral horns (gray matter), the interneuron decussates across the spinal cord and ascends the spinal tract, to ultimately pass this information to the brain. Once the interneurons make the transition of traveling either up or down to transmit information, they then become known as intersegmental neurons. Otherwise, they are called intra segmental neurons – staying within the same singular segment.
The bundle of nerve fibers that travel up and down the spinal cord is called the spinothalamic tract. This tract passes through the medulla oblongata on its way up to the thalamus (most sensory information passes through the thalamus before anywhere else in the brain). The tract then exits the thalamus, where it transitions into mostly white matter (myelin-sheathed axons) to ultimately synapse in the primary sensory level of the cerebral cortex.
Anatomy of the Vertebrae and Spinal Cord
The vertebrae, along with the cerebrospinal fluid (CSF) which flows throughout the central canal along the entire length of the spinal cord, are essential to the safety structure of the spinal cord. The CSF supplies a buffer of sorts that absorbs any shock that may result from possible impact, while the vertebrae surround the spinal cord, maintaining its structure and providing a hard exterior for increased protection.
The spine – the poster portion of the vertebral column – is made up of the posterior, or dorsal, parts of the vertebrae called spinous processes (the pokey parts of the vertebrae that create the bumps you see on your back when you bend over). The main bodies of the vertebrae are on the anterior, or ventral, side of the vertebral column. The center of the vertebral column through which the spinal cord passes is known as the vertebral foramen, or the vertebral canal.
Surrounding the spinal cord and the brain are three meninges, or membranes, called the dura mater (the outermost layer), the arachnoid (middle layer), and the pia mater (the innermost membrane). These, in addition to the CSF, function not only for the protection of the spinal cord, but also the regulation of the neuronal environment by removing toxins, circulating nutrients, and containing blood vessels vital to the organs of the nervous system. The space between the pia mater and the arachnoid membrane is called the subarachnoid space and is also filled with CSF.
Nerves of the Spinal Cord

The spinal nerves, the lifeline of communication for the PNS, originating from the spinal cord are – listed from the top, down, or superior to inferior – 8 pairs of cervical nerves (C1-C8), 12 pairs of thoracic spinal nerves (T1-T12), 5 pairs of lumbar spinal nerves (L1-L5), 5 pairs of sacral spinal nerves (S1-S5), and one single coccygeal nerve, all of them being “mixed nerves,” both sensory and motor.
The nerves emerging from the spinal cord are not simply extensions of the spinal nerve, rather, a “reorganization” of the axons of those nerves that ultimately follow different routes. When axons of different spinal nerves join they form what is called a systemic nerve. Systemic nerves are formed at four places along the entirety of the vertebral column – these sites are referred to individually as a nerve plexus (“plexus” is typically used in reference to describe a network of nerve fibers that have no associated cell bodies).
Two of these nerve plexuses are located at the cervical level, one at the lumbar level, and one at the sacral level.
- Cervical Plexus : made up of axons from spinal nerves C1-C5, axons of this plexus innervate the posterior neck and head and also connects to the phrenic nerve (controls the diaphragm).
- Brachial Plexus : made up of axons from spinal nerves C4-T1, this plexus innervates the arms. The radial nerve comes from this plexus and gives rise to the axillary nerves that innervate the armpit. (More on this another time.)
- Lumbar Plexus : comprised of all the lumbar spinal nerves and innervates the pelvic region and anterior leg. A major nerve from this plexus is the femoral nerve.
- Sacral Plexus : this plexus comes is composed of the lower lumbar nerves, L4 and L5, and sacral nerves, S1 to S4. The most prominent nerve to arise from this plexus is the sciatic nerve.
Inside the Spinal Cord
Let’s do one of those image interpretation tests: When you look at the center of the spinal cord, what do you see? Most people see this shape as either an “H” or a butterfly – I’m here to tell you that if you see an “H,” you need to work on your handwriting. The wings of the butterfly make up the portion of the gray matter known as the lateral gray horn. This gray matter is surrounded by white matter that doesn’t go by any particular name but is responsible for transmitting information up and down the spinal cord, to and from the brain.
There are two branches that project from either side of the spinal cord: the branch on the anterior, or ventral, side is known as the ventral root, and the one on the dorsal, or posterior side, is called the dorsal root. Located within the dorsal root is a structure called the dorsal root ganglion. Sensory information is passed through the dorsal root ganglion after passing through the point at which the two branches meet. This joining of the dorsal and ventral branches creates a spinal nerve that can be both types of sensory nerves: general sensory and motor (because of this, they are called “mixed nerves”).
(The dorsal root ganglion functions as the “sensory branch” of the spinal nerve and is therefore known as the “spinal ganglion” as well. Within this ganglion are the cell bodies of somatic and visceral sensory nerve fibers. The ventral root, on the other hand, functions as the motor branch of the spinal nerve, sending signals down from the brain based on information gathered from sensory neurons.)
The anatomical regions of the gray matter butterfly each are known as the following:
- The horns extending toward the dorsal side of the butterfly are called the dorsal gray horns . This is where sensory nerve fibers synapse onto interneurons.
- There are two processes in between the two butterfly wings pointing outward called the lateral gray horns . Now, these are quite unique in that they are only located at the thoracic and lumbar levels of the spinal cord. They are also the home of autonomic neuron cell bodies.
- Lastly, the horns that point toward the ventral side of the spinal cord are known as the ventral gray horns . This is where the cell bodies of somatic motor neurons, also known as alpha motor neurons or lower motor neurons, are located.
- In the very center of the gray butterfly is the central canal . As previously mentioned, through this canal runs CSF which distributes nutrients and removes toxins from the neuronal environment and provides a buffer for any possible traumatic impact to the spinal cord or vertebral column. CSF goes on to distribute throughout the rest of the body by exiting the central canal at the bottom of the spinal cord.
- The area just above the central canal is called the posterior gray commissure , where the nerve fibers decussate (remember, this is to cross from one side of the midline to the other). Opposite of this is the anterior gray commissure.
Inside the spinal nerve (the point at which the dorsal and ventral roots join) are both sensory neurons, which send information to the CNS, as well as motor neurons, which send commands out to the skeletal muscles (effectors) from the brain or spinal cord, depending on the type of movement and muscle to which the command is being sent. The sensory neurons pass through the dorsal root branch, and the motor neurons pass through the ventral root branch.
As the transitional structure between the CNS and PNS, the spinal cord is another absolutely essential part of the nervous system that has many different functions in distributing information for sensory and motor functions, including continuous autonomic processes. The extent of its reach in functionality throughout the entire human body is enormous and still being explored!
- Biga, L. M., Dawson, S., Harwell, A., Hopkins, R., Kaufmann, J., LeMaster, M., … Matern, P. (n.d.). 13.3 spinal and cranial nerves – Anatomy & physiology. Retrieved from https://open.oregonstate.education/aandp/chapter/13-3-spinal-and-cranial-nerves/
- Fink. (2013, January 17). The spinal cord & spinal tracts; part 1 by Professor Fink [Video file]. Retrieved from https://www.bing.com/videos/search?q=youtube+the+spinal+cord&view=detail&mid=7D51F1D2918F665B2CC97D51F1D2918F665B2CC9&FORM=VIREFink. (2013, January 17). The spinal cord & spinal tracts; part 2 by Professor Fink [Video file]. Retrieved from https://www.bing.com/videos/search?q=youtube+the+spinal+cord&&view=detail&mid=D0413958C915777BBCEBD0413958C915777BBCEB&&FORM=VDRVRV
Image source
http://vanat.cvm.umn.edu/neurLab2/SpinalPath.html
Share this:
Neuroscientists Discover That Spinal Cords Can Make Their Own Memories
Y our spinal cord can make its own memories independent of your brain, neuroscientists have found. The discovery opens up new avenues for treating patients in recovery from spinal cord injuries and challenges what we thought we knew about neural circuits outside the brain.
It's been known for some time that the spinal cord can initiate reflex movements without the brain's involvement. We have even seen in observational studies that the spinal cord appears to learn and adjust movements based on previous experience. However, the neuronal mechanisms underlying these observations have so far been a mystery.
"Learning and memory is often attributed as functions of the brain exclusively," Aya Takeoka, a principal investigator at Japan's RIKEN Center for Brain Science, told Newsweek . "Although scientists knew for more than a century that the spinal cord could learn and adapt movements in the absence of brain input, we did not know how the spinal cord learns and memorize what is has learned.
"Gaining insights into the underlying mechanism is essential if we want to understand the foundations of movement automaticity in healthy people and use this knowledge to improve recovery after spinal cord injury."
To explore the roles of these neurons in reflexive learning, Takeoka and her colleagues first set about demonstrating whether the spinal cord's cells can adapt to sensory inputs in the absence of signals from the brain.
To do so, the team used a pair of mice that had received a spinal cord transection, meaning their spinal cord was cut off from their brains. One mouse was designated as the "control," the other "experimental." Both were then dangled from a harness.
When the experimental mouse's legs dangled too low, it received a brief electric shock. The control mouse received the same shock at the same time, regardless of its leg position.
After just 10 minutes, the legs of the experimental mouse remained high up to avoid any electrical stimulation. The legs of the control mouse, meanwhile, continued to dangle. The next day, the test was repeated, but the mice were reversed (the control mouse was not given shocks when its legs dangled down).
The original experimental mouse still held its legs up high, indicating that its spinal cord had retained the memory from its previous experience. In other words, the spinal cord had learned and remembered to hold the mouse's legs up high to avoid unpleasant stimulation, independently from the animal's brain.
So far, the researchers had confirmed that the transected spinal cord could learn and remember independently of the brain, but exactly what neuronal circuitry was at play was not known. To answer this question, the team used another cohort of mice, this time a group of genetically modified mice, each with one of six different sets of spinal neurons disabled. These mice, which had also received a spinal cord transection, then underwent the same protocol as before.
Those mice that had the neurons toward the tops of their spinal cords disabled did not adapt to avoid the electrical stimulation. However, when it came to remembering the electrical stimulation the next day, a group of neurons at the bottom of the spinal cord appeared to be the most critical.
In other words, there appears to be a division of labor when it comes to learning and memory among the neurons of the spinal cord. "The two groups of nerve cells have distinct functions; learning cells are not needed for recalling what the spinal cord had learned, and memory cells were not needed for learning," Takeoka said.
The team hope that their findings, published in the journal Science , will help in the development of rehabilitative training methods for patients recovering from spinal cord damage.
"Not only do these results challenge the prevailing notion that motor learning and memory are solely confined to brain circuits, but we showed that we could manipulate spinal cord motor recall, which has implications for therapies designed to improve recovery after spinal cord damage," Takeoka said.
Is there a health problem that's worrying you? Let us know via [email protected]. We can ask experts for advice, and your story could be featured in Newsweek .
Related Articles
- Alzheimer's Breakthrough as 'Protective' Gene May Open Up New Treatments
- How Your Personality Type May Put You at Risk of Depression
- Scientists Discover Possible New Treatment for Alzheimer's
Start your unlimited Newsweek trial
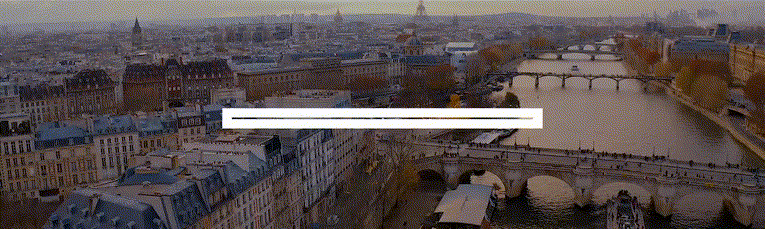
IMAGES
VIDEO
COMMENTS
These motor signals travel down the internal capsule, and then cross over to the other side of the body in the brain stem. From there, these messages are sent to the anterior horn and the lateral and anterior tracts of the spinal cord. The motor message exits the spinal cord through the ventral root (the front portion) of the spinal nerves.
The spinal cord is a long, tube-like band of tissue. It connects your brain to your lower back. Your spinal cord carries nerve signals from your brain to your body and vice versa. These nerve signals help you feel sensations and move your body. Any damage to your spinal cord can affect your movement or function.
Impulses travel up (to the brain) or down (from the brain) the spinal cord through distinct pathways (tracts). Each tract carries a different type of nerve signal either going to or from the brain. The following are examples: Lateral spinothalamic tract: Signals about pain and temperature, received by the sensory horn, travel through this tract ...
The spinal cord consists of ascending and descending tracts.The ascending tracts are sensory pathways that travel through the white matter of the spinal cord, carrying somatosensory information up to the brain. They allow you to feel sensations from the external environment (exteroceptive) such as pain, temperature, touch, as well as proprioceptive information from muscles and joints.
The upper and lower motor neurons form a two-neuron circuit. The upper motor neurons originate in the cerebral cortex and travel down to the brain stem or spinal cord, while the lower motor neurons begin in the spinal cord and go on to innervate muscles and glands throughout the body.
Neuromuscular junction (Synapsis neuromuscularis) At its simplest, the neuromuscular junction is a type of synapse where neuronal signals from the brain or spinal cord interact with skeletal muscle fibers, causing them to contract. The activation of many muscle fibers together causes muscles to contract, which in turn can produce movement.
EMG and nerve conduction studies are tests to see if you have muscle weakness or loss of feeling from an injury to your spinal cord, muscles, or nerves. To do an EMG, doctors insert small needles into a muscle to record your muscle's electrical activity when it's resting and when it's active. To do nerve conduction studies, doctors use ...
The upper motor neuron is in this region, called the precentral gyrus of the frontal cortex, which has an axon that extends all the way down the spinal cord. At the level of the spinal cord at which this axon makes a synapse, a graded potential occurs in the cell membrane of a lower motor neuron. This second motor neuron is responsible for ...
Anatomy. The spinal cord is part of the central nervous system (CNS). It is situated inside the vertebral canal of the vertebral column. During development, there's a disproportion between spinal cord growth and vertebral column growth. The spinal cord finishes growing at the age of 4, while the vertebral column finishes growing at age 14-18.
Spinal Cord Communication. The spinal cord acts as a conduit for information traveling up and down its length. But because most of this information has to either exit the spinal cord to send signals to peripheral tissues (efferent transmission), or information from peripheral tissues needs to be carried into the spinal cord (afferent transmission), there must be appropriate structures for ...
When you want to move your arms and legs, signals travel down your spinal cord, which is enclosed in your spine. Nerves carry the signals from the spinal cord to the muscles you want to move. Advertisement. ... Muscles attach to protrusions on the vertebrae called processes. When the muscles contract, they pull on these leverlike surfaces, and ...
When the muscle experiences an increase in tension, the collagen fibrils squeeze the 1b sensory axon, causing a signal to travel down the 1b axon toward the spinal cord. 'Golgi tendon organ' by Valerie Hedges is licensed under a Creative Commons Attribution Non-Commercial Share-Alike (CC BY-NC-SA) 4.0 International License.
The spinal cord carries out two main functions: It connects a large part of the peripheral nervous system to the brain. Information (nerve impulses) reaching the spinal cord through sensory neurons are transmitted up into the brain. Signals arising in the motor areas of the brain travel back down the cord and leave in the motor neurons.
The spinal cord is a nervous system structure dedicated to relaying information from the periphery to the brain and back, as well as carrying out certain levels of integration, such as those found in many reflexes. The structure of the spinal cord aids it in carrying out these relaying and integrative functions. Gross Anatomy of the Spinal Cord
These axons then travel to the spinal cord level at which they synapse with a lower motor neuron. ... resulting in activation of the muscle spindle that sends a signal into the spinal cord through the dorsal root. ... The examiner watches for the presence of tremors that would not be present if the muscles are relaxed. By pushing down on the ...
They arise from the cerebral cortex or brainstem and travel down the spinal cord to synapse in the anterior gray horn. Second-order neurons, or lower motor neurons (LMN), travel from the spinal cord to skeletal muscles, innervating them. This article will describe the anatomy and function of the descending tracts of the spinal cord.
The sensory pathway ends when the signal reaches the cerebral cortex. After integration with neurons in other parts of the cerebral cortex, a motor command is sent from the precentral gyrus of the frontal cortex. The upper motor neuron sends an action potential down to the spinal cord. The target of the upper motor neuron is the dendrites of ...
The signals also tell your muscles specifically when and how to move. These signals are not like sensory signals. These signals don't begin at the nerve endings; they begin inside the brain. From there, they travel through the spinal cord, out to the spinal nerves, and then on to the parts of the body. ... Spinal Cord Signals: Reflex.
"The part of the motor cortex that's responsible for the parts of the hand that will be moving fires a signal," Gizerian said. "Those signals travel down through the brain stem and spinal cord and pass that signal on to a neuron that goes out to the muscles. Then the muscles contract." Neurons are nerves cells.
EMG and nerve conduction studies are tests to see if you have muscle weakness or loss of feeling from an injury to your spinal cord, muscles, or nerves. To do an EMG, doctors insert small needles into a muscle to record your muscle's electrical activity when it's resting and when it's active. To do nerve conduction studies, doctors use ...
The spinal nerves, the lifeline of communication for the PNS, originating from the spinal cord are - listed from the top, down, or superior to inferior - 8 pairs of cervical nerves (C1-C8), 12 pairs of thoracic spinal nerves (T1-T12), 5 pairs of lumbar spinal nerves (L1-L5), 5 pairs of sacral spinal nerves (S1-S5), and one single coccygeal nerve, all of them being "mixed nerves," both ...
Story by Pandora Dewan. • 6h • 3 min read. Your spinal cord can make its own memories independent of your brain, neuroscientists have found. The discovery opens up new avenues for treating ...
Upper motor neurons originate primarily in the cerebral cortex (primary motor cortex) and extend towards the brainstem or spinal cord, where they synapse. Upper motor neurons travel down specific pathways, including the pyramidal, extrapyramidal, rubrospinal, tectospinal and reticulospinal tracts. Glutamate is used as a neurotransmitter.