- Complete List of Animals
- Animals that start with A
- Animals that start with B
- Animals that start with C
- Animals that start with D
- Animals that start with E
- Animals that start with F
- Animals that start with G
- Animals that start with H
- Animals that start with I
- Animals that start with J
- Animals that start with K
- Animals that start with L
- Animals that start with M
- Animals that start with N
- Animals that start with O
- Animals that start with P
- Animals that start with Q
- Animals that start with R
- Animals that start with S
- Animals that start with T
- Animals that start with U
- Animals that start with V
- Animals that start with W
- Animals that start with X
- Animals that start with Y
- Animals that start with Z
- Parks and Zoos
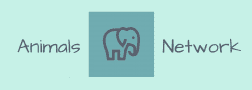
- Diomedeidae
- Diomedea exulans
- Procellariiformes
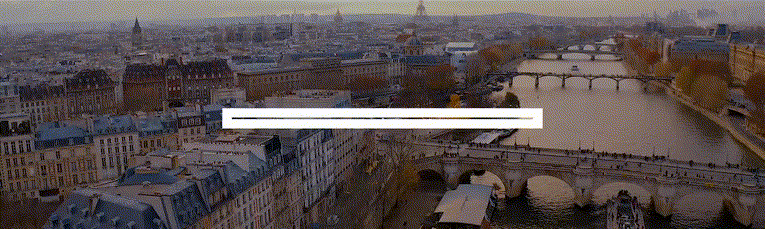
Wandering Albatross
The Wandering Albatross is a massive bird known by many names. In various regions, people call this bird a Snowy Albatross, Goonie, and White Winged Albatross.
Not only are they the largest of the 22 albatross species, but they also have the longest wingspan of any bird. Their wings commonly measure up to 10 ft. across, and the largest confirmed specimen had a wingspan over 12 ft. across! Read on to learn about the Wandering Albatross .
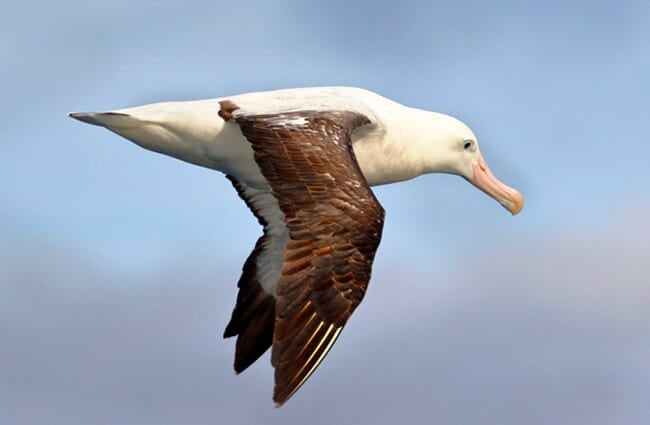
Description of the Wandering Albatross
This species of albatross has white plumage, or feathers, with darker wings. Their wing feathers are black, and speckled with varying degrees of white. Young birds have brown feathers, which become white as they age.
This bird’s wingspan is quite large, and averages 10 feet across, though some individuals are larger. Finally, their beaks are moderately long, with a hook at the end to help grasp fish.
Interesting Facts About the Wandering Albatross
This species has the longest wingspan of any living bird … Ever! However, that is not the only notable thing about the Wandering Albatross.
- Monogamous Mates – Once a Wandering Albatross has found a suitable mate, it continues to breed with that bird for the rest of its life. They are doting parents, and take great care in rearing their chicks. It sometimes takes up to 10 months for the chick to learn how to fly and become independent of its parents.
- Time Constraints – Obviously when it takes 10 months to raise a single chick, it can be difficult to jump right back into parenthood. For this reason, Wandering Albatrosses breed once every 2 years.
- Slow to Mature – Adult albatrosses don’t even begin reproducing until they are about 10 years old on average. They sometimes join the other birds at the breeding colonies and perform mating displays. However, most of the time they do not find a mate and begin to breed until they are around 10 years old.
- Slow Growth – Unfortunately, because these birds are so slow to mature, and they breed at a very slow rate, their populations do not increase quickly. Because of this, when their populations decline it takes a long time for them to make a comeback. Humans pose threats to these birds in a number of different ways, and the IUCN lists the species as Vulnerable .
Habitat of the Wandering Albatross
These birds spend the vast majority their life flying over, or floating on the surface of, the ocean. They inhabit the open ocean, primarily where the waters are deep, and fish are plentiful. The only time they come to land is for the mating season. During this time, colonies of birds land on plateaus, valleys, and plains.
Distribution of the Wandering Albatross
There are several different subspecies of Wandering Albatross, all of which live in the open oceans of the Southern Hemisphere. Outside of the breeding season, they roam the open oceans in between Antarctica and the southern coasts of Africa, South America, and Australia. Their primary breeding colonies are on various islands across the Southern Hemisphere, including South Georgia, Macquarie, Amsterdam Island, and more.
Diet of the Wandering Albatross
This seabird unsurprisingly feeds primarily on fish and other aquatic organisms. They eat fish, octopus, squid, shrimp, and krill.
They also scavenge on the remains of carcasses, as well as feeding on the scraps from commercial fishing operations and other predators. Though they can dive if they need to, they catch most of their food at the surface of the water.
Wandering Albatross and Human Interaction
Unfortunately, humans are extremely detrimental to these birds. Sailors have killed birds, both at sea and in nesting colonies, for decades. In fact, humans are the only known predator of adult albatrosses.
Nowadays it is illegal to harm these birds, though killing does still occur. Sadly, they frequently, and accidentally, become trapped in fishing nets or on fishing lines. Humans have also introduced many different feral animals to their breeding islands, and these animals eat the eggs and chicks.
Domestication
Humans have not domesticated this species of bird in any way.
Does the Wandering Albatross Make a Good Pet
No, the Wandering Albatross does not make a good pet. Their huge wings carry them across open ocean, which would make them a poor household pet. It most places, it is illegal to harm, harass, capture, or kill these birds.
Wandering Albatross Care
These birds do not often find themselves in zoos. The only time any albatross species lives in a zoo or aquarium is when something has severely injured them in some way.
During those times, zoos attempt to heal and rehabilitate the birds, and release them back into the wild if possible. Albatrosses that live in zoos because they cannot survive in the wild act as ambassadors to the plight of their species.
Behavior of the Wandering Albatross
This species is quite social, even outside of the breeding season. While in the open ocean, small groups of Wandering Albatrosses forage together. These groups frequently converge upon one another when feeding opportunities, like bait balls or fishing vessels, arise.
As the breeding season arrives, huge colonies of birds flock to their breeding grounds together. Birds searching for mates perform elaborate courtship displays, and mated pairs renew their bonds.
Reproduction of the Wandering Albatross
Every 2 years a pair breeds and produces a single egg, usually in December. Both the male and the female help incubate the egg, which hatches after 2.5 months. Once the chick hatches the parents alternate between keeping it warm and fishing for food.
After the chick is a month old, both parents leave it alone to hunt for food. It takes between 9 and 10 months for the chick to learn how to fly and gain independence.
RELATED ARTICLES MORE FROM AUTHOR
![Red Angus Closeup of a beautiful Red Angus cowPhoto by: U.S. Department of Agriculture [pubic domain]https://creativecommons.org/licenses/by/2.0/](https://animals.net/wp-content/uploads/2020/03/Red-Angus-4-238x178.jpg)
Paint Horse
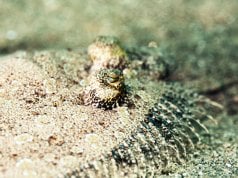
Expert Recommendations
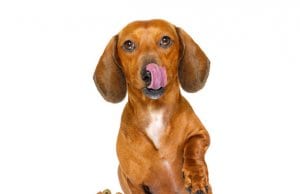
Best Dog Food For Allergies
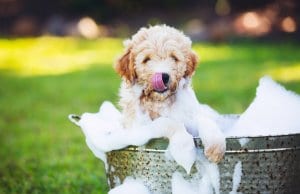
Best Dog Shampoo
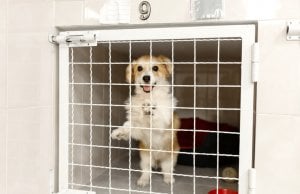
Best Dog Gate
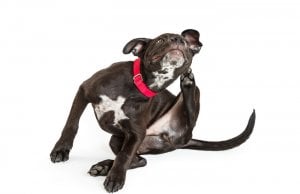
Best Dog Food for Skin Allergies
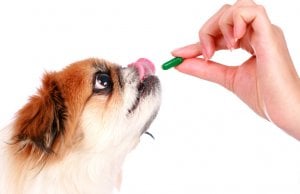
Best Dog Vitamins
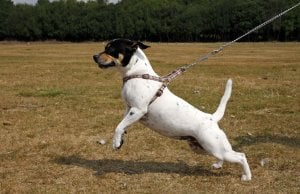
Best No Pull Dog Harness
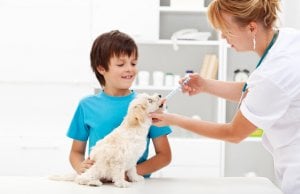
Best Dog Dewormer
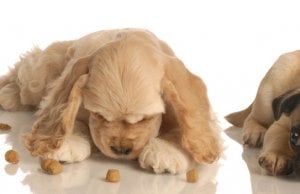
Best Natural Dog Food
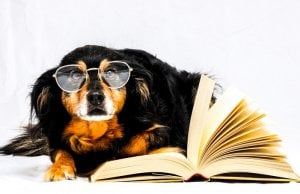
Best Dog Training Books
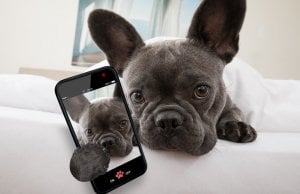
Best Dog Camera
Even more news.
![Red Angus Closeup of a beautiful Red Angus cowPhoto by: U.S. Department of Agriculture [pubic domain]https://creativecommons.org/licenses/by/2.0/](https://animals.net/wp-content/uploads/2020/03/Red-Angus-4-100x75.jpg)
House Spider
Popular category.
- Chordata 694
- Mammalia 247
- Dog Breeds 184
- Actinopterygii 121
- Reptilia 87
- Carnivora 72
- Privacy Policy
- Terms and Conditions

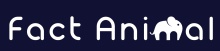
Fact Animal
Facts About Animals
Wandering Albatross Facts
Wandering albatross profile.
In 1961, Dion and the Del Satins had a song from the perspective of an albatross. It wasn’t accurate on many counts, but it did get one thing right: they get around.
The Diomedea exulans, more commonly known as the wandering albatross is perhaps the most accomplished wanderer of any animal, with routine voyages of hundreds of kilometres per day on record-breaking wings.
They are a large seabird with a circumpolar range in the Southern Ocean, and sometimes known as snowy albatross, white-winged albatross or goonie.
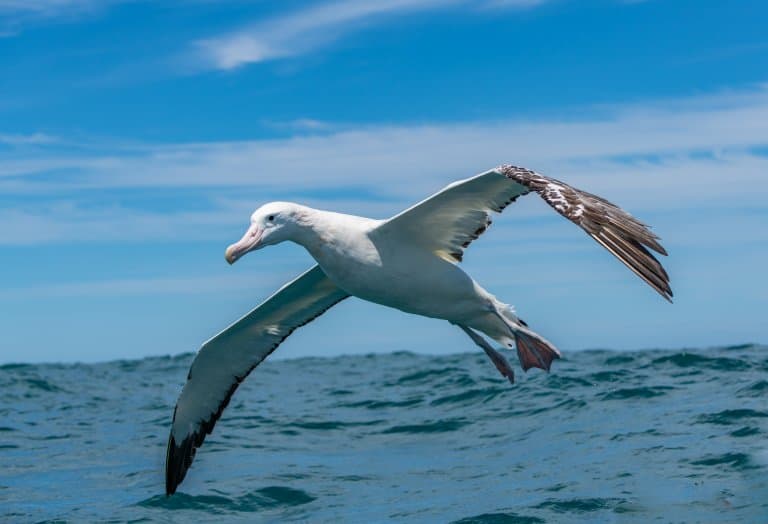
Wandering Albatross Facts Overview
The wandering albatross breeds on islands in the South Atlantic Ocean, such as South Georgia Island, Crozet Islands, Prince Edward Island and others.
They spend most of their life in flight , and land only to breed and feed.
These are phenomenal birds, capable of surviving some of the harshest weather conditions even at the most vulnerable stages of their development.
They are slow to reproduce, spending extra time to develop into one of the biggest and most specialised animals in the air.
Sadly, this is what makes them vulnerable to population declines, and longline fishing vessels are responsible for many adult deaths.
Interesting Wandering Albatross Facts
1. they can travel 120k km (75k) miles in a year.
The Wandering albatross might be the most wide-ranging of all foraging sea birds, and maybe of all animals. They’ve been tracked over 15,000 km in a single foraging trip, capable of speeds of up to 80 kmph and distances of over 900 km per day. 1
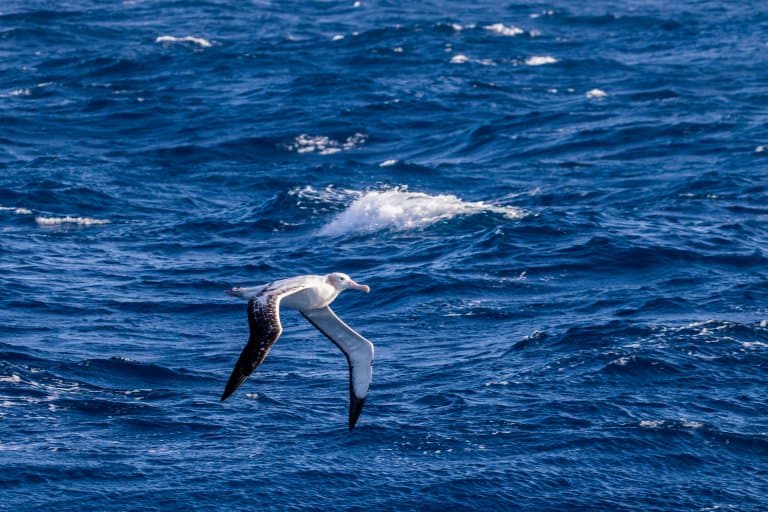
2. They’re monogamous (mostly)
This goes against the entire theme of the Del Satins song and is probably why it’s no longer used as a learning aid in the zoological curriculum.
Contrary to the promiscuous subject of the ‘60s hit, the Wandering Albatrosses mate for life and are (on average) monogamous.
When breeding, they take on incubation shifts, and it’s during these periods when the wanderer goes out on their epic voyages to return with food for their family.
Still, there’s an element of personal preference when it comes to breeding.
Most females will take a year or two off after the long and arduous task of reproduction. During this time the parents will go their separate ways, only to reunite when the time is right.
In these periods, some females will take on a temporary mate, so they can squeeze out one more chick before reuniting with their permanent nesting partner. 2
3. Wandering albatross are active in moonlight
When on these journeys, the albatross is almost constantly active. During the day they spend the entire time in the air, and while they don’t cover much distance at night, they were still recorded almost constantly moving – never stopping for more than 1.6h in the dark.
They appear to travel more on moonlit nights than on darker ones.
All of this data comes from satellite trackers attached to some birds, which are always going to skew the results.
Flying birds are optimised for weight, and trackers add to this weight, so there’s necessarily a negative effect on the individual’s fitness when lumbering them with a tracker.
Still, these subjects were able to outlast the trackers’ batteries on many occasions, and it’s safe to assume they’re capable of even more than we can realistically measure!
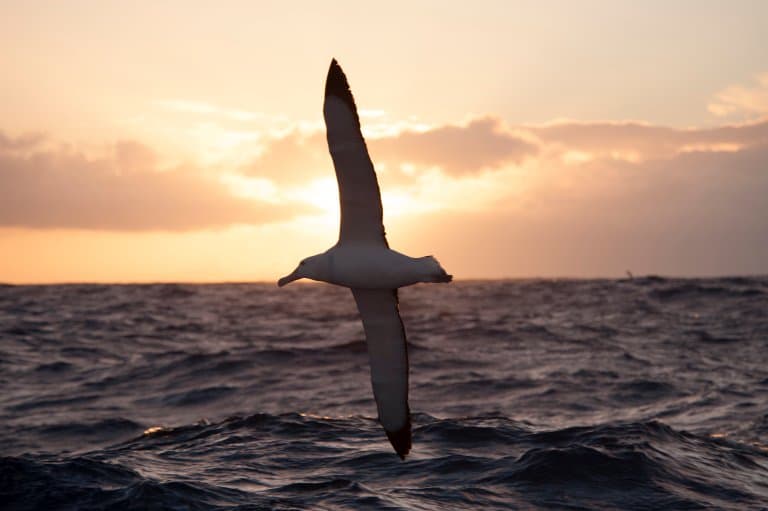
4. They have the largest wingspan of any bird in the world
One advantage that an albatross has over, say, a pigeon, when it comes to carrying a researcher’s hardware, is that it doesn’t need to flap much.
The albatross is the bird with the longest wingspan of any flying animal – growing up to 3.2 m (10.5 ft), and these wings are meticulously adapted for soaring.
The Guiness Book of Records claims the largest wingspan of any living species of bird was a wandering albatross with a wingspan of 3.63m (11 ft 11) caught in 1965 by scientists on the Antarctic research ship USNS Eltanin in the Tasman Sea.
Research has suggested that these wings function best against slight headwinds, and act like the sails of a boat, allowing the bird to cover more ground by “tacking”, like a sailboat: zig-zagging across the angle of the wind to make forward progress into it. 3
5. Fat chicks
As mentioned, these voyages are usually a result of foraging trips for their chicks.
The environment for a growing albatross is one of the least conducive for life. Freezing winter storms and exposed ledges make for a hilly upbringing for the baby birds.
Fed on a healthy diet of regurgitated squid, these albatross chicks grow to enormous sizes. On nesting sites, it’s not uncommon to find a fluffy baby albatross weighing up to 10kg.
These chicks are heavier than their parents, and they need the extra mass to protect them from the Winter season while they grow into fledglings. They’re also such big birds that they take longer than a season to reach maturity.
It takes around ten months of feeding, back and forth from the ocean every few days, for the parents to grow a healthy adult offspring.
6. Being a parent takes practice
When inexperienced parents were compared with those who’d brought up chicks before, it was found that their chicks are a little slower to fatten up, at least in the first few months.
Parents would feed less regularly, but with much larger amounts, and it seems to take a while to get the routine down.
By the end of the breeding season, these differences disappeared and the parents became fully qualified.
7. 25% of chicks die when they leave the colony
The huge chicks have one of the longest rearing periods of any bird, and this is after an 11-month incubation period! And if they survive all this, they still have a long way to go.
There’s a period of 3 to 7 years during which the young chick will leave the colony alone and spend the entire time at sea.
During the first two months of this learning phase, 25% of chicks die. This is a critical time for the young birds, but if they survive, they’ll return to the colony and find a mate. 4
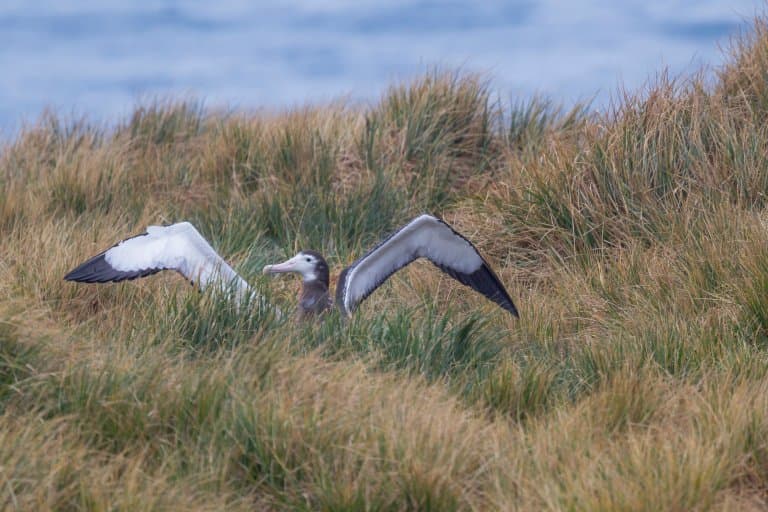
8. They’re good sniffers
These birds feed primarily on smelly things like squid, and they’ve developed a very keen sense of smell to find them from downwind.
Wandering Albatrosses have one of the largest olfactory bulbs of any bird and they’re honed to fishy aromas.
They combine this sense with strong vision to identify productive areas of the ocean for hunting and foraging. 5
9. They are part of a ‘species complex’
When multiple species are so similar in appearance and other features, it makes their boundaries unclear and this group is known as a species complex.
The wandering albatross was long considered the same species as the Tristan albatross and the Antipodean albatross. Along with the Amsterdam albatross, they form a species complex.
Taxonomy of animals in general is tricky, and some researchers still describe them as the same species.
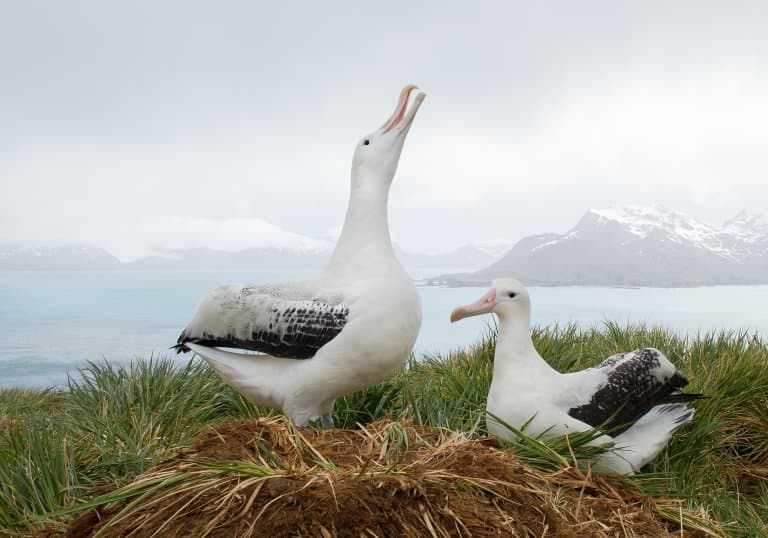
10. The wandering albatross is vulnerable
The ICUN has classified the wandering albatross as vulnerable, and the last study of their population size in 2007 indicated there were an estimated 25,000 birds.
The biggest threat to their survival is fishing, in particular longline fishing. This is where a long mainline is used with baited hooks, and they are prone to accidental catching of birds, as well as dolphins, sharks, turtles and other sea creatures. Pollution, mainly from plastics and fishing hooks is also a problem for birds such as the wandering albatross.
Convervation efforts are underway to reduce bycatch of albatrosses and some breeding islands are now classified as nature reserves.
Wandering Albatross Fact-File Summary
Scientific classification, fact sources & references.
- Jouventin, P., Weimerskirch, H (1990), “ Satellite tracking of Wandering albatrosses “, Nature.
- GrrlScientist (2022), “ Divorce Is More Common In Albatross Couples With Shy Males, Study Finds “, Forbes.
- Richardson, P. L., Wakefield, E. D., & Phillips, R. A. (2018), “ Flight speed and performance of the wandering albatross with respect to wind “, Movement Ecology.
- Weimerskirch, H., Cherel, Y., Delord, K., Jaeger, A., Patrick, S. C., & Riotte-Lambert, L. (2014), “ Lifetime foraging patterns of the wandering albatross: Life on the move! “, Journal of Experimental Marine Biology and Ecology.
- Nevitt, G. A., Losekoot, M., & Weimerskirch, H. (2008), “ Evidence for olfactory search in wandering albatross, Diomedea exulans “, Proceedings of the National Academy of Sciences.
{{ searchResult.title }}
Wandering Albatross
Diomedea exulans
Known for its majestic wingspan and far-ranging travels, the Wandering Albatross is a captivating presence in the Southern Ocean's expanse. As the bird with the widest wingspan globally, this remarkable creature glides effortlessly across vast oceanic distances, its brilliant white plumage and solitary habits making it a unique symbol of the wild, open sea.
On this page
Appearance and Identification
Vocalization and sounds, behavior and social structure, distribution and habitat, lifespan and life cycle, conservation status, similar birds.
Males and females have similar plumage
Primary Color
Primary color (juvenile), secondary colors.
Black, Grey
Secondary Colors (female)
Secondary colors (juvenile).
White, Grey
Secondary Colors (seasonal)
Wing color (juvenile).
Large, Hooked
Beak Color (juvenile)
Leg color (juvenile), distinctive markings.
Black wings, white tail, large pink beak
Distinctive Markings (juvenile)
Darker than adults, brown beak
Tail Description
White with black edges
Tail Description (juvenile)
Brown with white edges
Size Metrics
107cm to 135cm
250cm to 350cm
6.72kg to 12kg
Click on an image below to see the full-size version
Pair of Wandering Albatrosses
Juvenile Wandering Albatross
Wandering Albatross resting on the sea
Wandering Albatross in-flight over the ocean
Wandering Albatross at nest with downy chick
Primary Calls
Series of grunts and whistles
Call Description
Most vocal on breeding grounds, otherwise silent
Alarm Calls
Loud, harsh squawks
Daily Activities
Active during day, rests on water surface at night
Social Habits
Solitary at sea, social on breeding grounds
Territorial Behavior
Defends nest site during breeding season
Migratory Patterns
Non-migratory but wanders widely at sea
Interaction with Other Species
Occasionally forms loose flocks at sea
Primary Diet
Fish, Squid
Feeding Habits
Surface seizes and scavenges
Feeding Times
Day and night
Prey Capture Method
Plunge-diving, surface-seizing
Diet Variations
May eat carrion
Special Dietary Needs (if any)
Nesting location.
On ground on isolated islands
Nest Construction
Mound of mud and vegetation
Breeding Season
Every other year
Number of clutches (per breeding season)
Once every two years
Egg Appearance
White, oval
Clutch Characteristics
Incubation period.
Around 80 days
Fledgling Period
Approximately 9 months
Parental Care
Both parents incubate and feed chick
Geographic Range
Circumpolar in Southern Ocean
Habitat Description
Open ocean, breeds on remote islands
Elevation Range
Migration patterns, climate zones.
Polar, Temperate
Distribution Map
Please note, this range and distribution map is a high-level overview, and doesn't break down into specific regions and areas of the countries.
Non-breeding
Lifespan range (years)
Average lifespan, maturity age.
7-10 year(s)
Breeding Age
Reproductive behavior.
Monogamous, long-term pair bonds
Age-Related Changes
Younger birds are darker, gain white plumage with age
Current Status
Vulnerable (IUCN Red List)
Major Threats
Longline fishing, plastic ingestion, climate change
Conservation Efforts
Protected under international law, conservation programs on breeding islands
Population Trend
Slow but steady population decrease due to threats
Royal Albatross
Diomedea epomophora
Classification
Other names:
Snowy Albatross, White-winged Albatross
Population size:
Population trend:
Conservation status:
IUCN Red List
Get the best of Birdfact
Brighten up your inbox with our exclusive newsletter , enjoyed by thousands of people from around the world.
Your information will be used in accordance with Birdfact's privacy policy . You may opt out at any time.
© 2024 - Birdfact. All rights reserved. No part of this site may be reproduced without our written permission.
Albatrosses are threatened with extinction – and climate change could put their nesting sites at risk
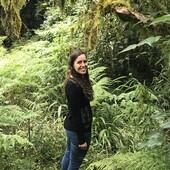
Postdoctoral research fellow, Department of Plant and Soil Science, University of Pretoria
Disclosure statement
Mia Momberg does not work for, consult, own shares in or receive funding from any company or organisation that would benefit from this article, and has disclosed no relevant affiliations beyond their academic appointment.
University of Pretoria provides funding as a partner of The Conversation AFRICA.
View all partners
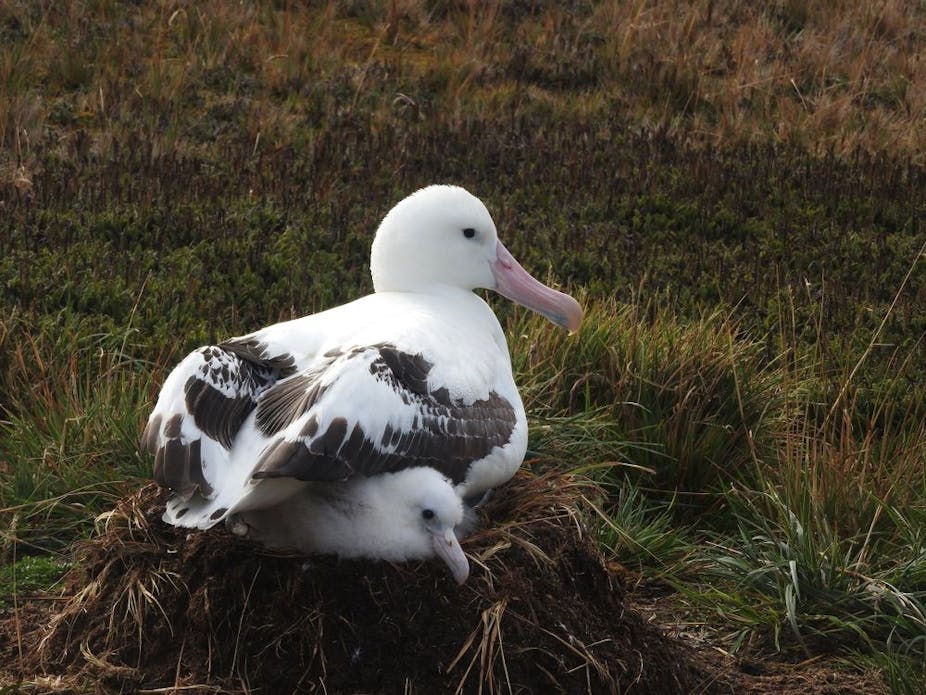
The wandering albatross ( Diomedea exulans ) is the world’s largest flying bird , with a wingspan reaching an incredible 3.5 metres. These birds are oceanic nomads: they spend most of their 60 years of life at sea and only come to land to breed approximately every two years once they have reached sexual maturity.
Their playground is the vast Southern Ocean – the region between the latitude of 60 degrees south and the continent of Antarctica – and the scattered islands within this ocean where they make their nests.
Marion Island and Prince Edward Island , about 2,300km south of South Africa, are some of the only land masses for thousands of kilometres in the Southern Ocean.
Together, these two islands support about half of the entire world’s wandering albatross breeding population, estimated at around 20,000 mature individuals . Every year scientists from South African universities survey Marion Island to locate and record each wandering albatross nest.
The species, listed as vulnerable by the International Union for Conservation of Nature , faces huge risks while in the open ocean, in particular due to bycatch from longline fishing trawlers. This makes it important to understand their breeding ecology to ensure that the population remains stable.
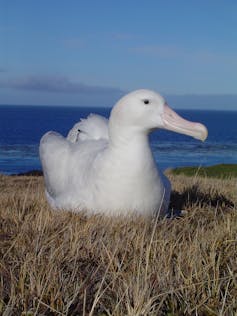
I was part of a study during 2021 to investigate which environmental variables affect the birds’ choice of nest site on Marion Island. The birds make their nests – a mound of soil and vegetation – on the ground. We looked at wind characteristics, vegetation and geological characteristics at nest locations from three breeding seasons.
Elevation turned out to be the most important variable – the albatrosses preferred a low (warmer) site and coastal vegetation. But these preferences also point to dangers for the birds from climate change. The greatest risk to the availability of nesting sites will be a much smaller suitable nesting range in future than at present. This could be devastating to the population.
Variables influencing nest site selection
Marion Island is of volcanic origin and has a rough terrain. Some areas are covered in sharp rock and others are boggy, with very wet vegetation. There is rain and strong wind on most days. Conducting research here requires walking long distances in all weathers – but the island is ideal for studying climate change, because the Southern Ocean is experiencing some of the largest global changes in climate and it is relatively undisturbed by humans.
Using GPS coordinate nest data from the entire breeding population on Marion Island, we aimed to determine which factors affected where the birds breed. With more than 1,900 nests, and 10,000 randomly generated points where nests are not present, we extracted:
elevation (which on this island is also a proxy for temperature)
terrain ruggedness
distance to the coast
vegetation type
wind turbulence
underlying geology.
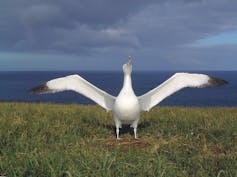
The variables were ranked according to their influence on the statistical model predicting the likelihood of a nest being present under the conditions found at a certain point.
The most important variable was elevation. The majority of the nests were found close to the coast, where the elevation is lower. These areas are warmer, which means that the chicks would be less exposed to very cold temperatures on their open nests.
The probability of nests being present also declined with distance from the coast, probably because there are more suitable habitats closer to the coast.
Vegetation type was strongly determined by elevation and distance from the coast. This was an important factor, as the birds use vegetation to build their nests. In addition, dead vegetation contributes to the soil formation on the island, which is also used in nest construction.
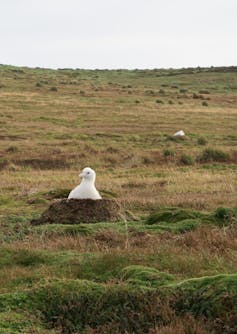
The probability of encountering nests is lower as the terrain ruggedness increases since these birds need a runway of flat space to use for take-off and landing. During incubation, the adults take turns to remain on the nest. Later they will leave the chick on its own for up to 10 days at a time. They continue to feed the chick for up to 300 days.
Areas with intermediate wind speeds were those most likely to have a nest. At least some wind is needed for flight, but too much wind may cause chicks to blow off the nests or become too cold.
Delicate balance
Changing climates may upset this delicate balance. Human-driven changes will have impacts on temperature, rainfall and wind speeds, which in turn affect vegetation and other species distribution patterns .
By 2003, Marion Island’s temperature had increased by 1.2°C compared to 50 years before. Precipitation had decreased by 25% and cloud cover also decreased, leading to an increase in sunshine hours . The permanent snowline which was present in the 1950s no longer exists . These changes have continued in the 20 years since their initial documentation, and are likely to continue.
Strong vegetation shifts were already documented in the sub-Antarctic years ago. Over 40 years, many species have shifted their ranges to higher elevations where the temperatures remain cooler. Wind speeds have also already increased in the Southern Ocean and are predicted to continue doing so, which may have effects on the size of areas suitable for nesting.
If nesting sites move to higher elevations on Marion Island as temperatures warm, and some areas become unsuitable due to changes in vegetation or wind speeds, it is likely that the suitable nesting area on the island will shrink considerably.
Our study adds to what is known about the elements affecting nest-site selection in birds. Notably, we add knowledge of wind, an underexplored element, influencing nest-site selection in a large oceanic bird. The results could also provide insights that apply to other surface-nesting seabirds.
- Climate change
- Southern ocean
- Natural world

Sydney Horizon Educators (Identified)

Senior Disability Services Advisor

Deputy Social Media Producer

Associate Professor, Occupational Therapy

GRAINS RESEARCH AND DEVELOPMENT CORPORATION CHAIRPERSON
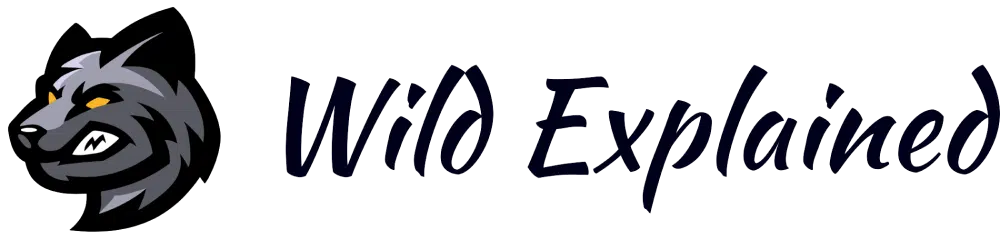
Animal encyclopedia
Exploring the magnificent wandering albatross.
September 4, 2023

John Brooks
September 4, 2023 / Reading time: 6 minutes

Sophie Hodgson
We adhere to editorial integrity are independent and thus not for sale. The article may contain references to products of our partners. Here's an explanation of how we make money .
Why you can trust us
Wild Explained was founded in 2021 and has a long track record of helping people make smart decisions. We have built this reputation for many years by helping our readers with everyday questions and decisions. We have helped thousands of readers find answers.
Wild Explained follows an established editorial policy . Therefore, you can assume that your interests are our top priority. Our editorial team is composed of qualified professional editors and our articles are edited by subject matter experts who verify that our publications, are objective, independent and trustworthy.
Our content deals with topics that are particularly relevant to you as a recipient - we are always on the lookout for the best comparisons, tips and advice for you.
Editorial integrity
Wild Explained operates according to an established editorial policy . Therefore, you can be sure that your interests are our top priority. The authors of Wild Explained research independent content to help you with everyday problems and make purchasing decisions easier.
Our principles
Your trust is important to us. That is why we work independently. We want to provide our readers with objective information that keeps them fully informed. Therefore, we have set editorial standards based on our experience to ensure our desired quality. Editorial content is vetted by our journalists and editors to ensure our independence. We draw a clear line between our advertisers and editorial staff. Therefore, our specialist editorial team does not receive any direct remuneration from advertisers on our pages.
Editorial independence
You as a reader are the focus of our editorial work. The best advice for you - that is our greatest goal. We want to help you solve everyday problems and make the right decisions. To ensure that our editorial standards are not influenced by advertisers, we have established clear rules. Our authors do not receive any direct remuneration from the advertisers on our pages. You can therefore rely on the independence of our editorial team.
How we earn money
How can we earn money and stay independent, you ask? We'll show you. Our editors and experts have years of experience in researching and writing reader-oriented content. Our primary goal is to provide you, our reader, with added value and to assist you with your everyday questions and purchasing decisions. You are wondering how we make money and stay independent. We have the answers. Our experts, journalists and editors have been helping our readers with everyday questions and decisions for over many years. We constantly strive to provide our readers and consumers with the expert advice and tools they need to succeed throughout their life journey.
Wild Explained follows a strict editorial policy , so you can trust that our content is honest and independent. Our editors, journalists and reporters create independent and accurate content to help you make the right decisions. The content created by our editorial team is therefore objective, factual and not influenced by our advertisers.
We make it transparent how we can offer you high-quality content, competitive prices and useful tools by explaining how each comparison came about. This gives you the best possible assessment of the criteria used to compile the comparisons and what to look out for when reading them. Our comparisons are created independently of paid advertising.
Wild Explained is an independent, advertising-financed publisher and comparison service. We compare different products with each other based on various independent criteria.
If you click on one of these products and then buy something, for example, we may receive a commission from the respective provider. However, this does not make the product more expensive for you. We also do not receive any personal data from you, as we do not track you at all via cookies. The commission allows us to continue to offer our platform free of charge without having to compromise our independence.
Whether we get money or not has no influence on the order of the products in our comparisons, because we want to offer you the best possible content. Independent and always up to date. Although we strive to provide a wide range of offers, sometimes our products do not contain all information about all products or services available on the market. However, we do our best to improve our content for you every day.
Table of Contents
The Wandering Albatross is a truly remarkable bird that captivates the imagination of wildlife enthusiasts and researchers alike. With its impressive wingspan and majestic flight, this magnificent creature has a unique story to tell. In this article, we will delve into the world of the Wandering Albatross, exploring its characteristics, habitat, life cycle, diet, threats, conservation efforts, and even its role in culture and literature.
Understanding the Wandering Albatross
The Wandering Albatross, a majestic seabird, is a fascinating creature that captures the imagination with its impressive size and unique characteristics . Let’s delve deeper into the defining features and habitat of this remarkable bird.
Defining Characteristics of the Wandering Albatross
With a wingspan of up to 11 feet, the Wandering Albatross boasts the largest wingspan of any bird in the world. This remarkable wingspan allows it to glide effortlessly over the vast open oceans it calls home. As it soars through the air, its wingspan creates a mesmerizing spectacle, showcasing the bird’s incredible adaptability to its environment.
The Wandering Albatross is easily recognizable by its distinctive white feathers , sleek body, and long, slender wings . These defining features not only contribute to its graceful appearance but also serve a purpose in its survival. The white feathers help camouflage the bird against the bright sunlight reflecting off the ocean’s surface, while the sleek body and long wings enable it to navigate the winds with precision.
The Albatross’s Unique Habitat
These graceful birds are found primarily in the southern oceans, particularly around the Antarctic region. The vast expanse of the Southern Ocean provides an ideal environment for the Wandering Albatross to thrive. With its ability to cover immense distances, the bird utilizes the strong winds to its advantage, effortlessly gliding across the ocean in search of food and suitable breeding grounds.
During their long journeys, Wandering Albatrosses traverse various oceanic regions, from the sub-Antarctic to as far as the coast of South America. Their nomadic lifestyle allows them to explore different ecosystems , adapting to the ever-changing conditions of the open ocean.
When on land, the Wandering Albatross prefers remote and isolated islands for nesting. These islands provide the perfect breeding environment, away from human disturbance and terrestrial predators. Here, amidst the rugged cliffs and pristine beaches, the albatrosses establish their colonies, creating a spectacle of life in the midst of the vast ocean.
These incredible birds are known to return to the same nesting sites year after year, demonstrating their strong site fidelity . The remote islands become their sanctuary, where they engage in courtship rituals, build nests, and raise their young. It is a testament to their resilience and adaptability that they have managed to maintain these nesting sites for generations, despite the challenges they face in the ever-changing world.
As we continue to explore and understand the Wandering Albatross, we uncover more about its remarkable adaptations, behaviors, and interactions with its environment. The more we learn, the more we appreciate the intricate web of life that exists in the vast oceans, where these magnificent birds reign supreme.
The Life Cycle of the Wandering Albatross
Breeding and nesting patterns.
The breeding season for the Wandering Albatross begins in the austral summer months, with courtship rituals that involve intricate displays of dance and vocalizations . These courtship displays are not only a way for the albatrosses to attract a mate but also a means of establishing dominance within their colony. The males showcase their impressive wingspan and perform elaborate dances, while the females respond with their own graceful movements.
Once a pair bonds, they establish a nest on the chosen island and begin the process of reproduction. The nests are carefully constructed using a combination of soil, grass, and other materials found on the island. The albatrosses take great care in selecting the perfect location for their nest, ensuring it is protected from the harsh elements and predators.
The female typically lays a single egg, which both parents take turns incubating. Incubation lasts for approximately 60 days, during which the parents rotate shifts to keep the egg warm and protected. This shared responsibility is a testament to the strong bonds formed between Wandering Albatross pairs. The parents take turns leaving the nest to search for food, returning to regurgitate the nutrient-rich meal for their growing chick.
During the incubation period, the albatrosses face numerous challenges. They must withstand strong winds, freezing temperatures, and potential threats from predators . Despite these difficulties, the dedicated parents remain vigilant, ensuring the survival of their offspring.
Growth and Development Stages
After hatching, the chicks are cared for and fed by both parents. The parents regurgitate a nutrient-rich oil that provides essential nourishment for the growing chick. This feeding process continues for several months until the chick becomes independent enough to forage on its own. The oil not only provides the necessary nutrients but also helps to strengthen the chick’s immune system, protecting it from potential diseases.
As the chick grows, it undergoes various developmental stages. Its downy feathers gradually give way to juvenile plumage, which is darker in coloration. The chick’s beak also undergoes changes, becoming stronger and more adapted to catching prey. During this time, the parents continue to provide guidance and protection, teaching the chick essential survival skills.
It takes years for a Wandering Albatross chick to reach maturity. During this time, they undergo a remarkable transformation, gradually developing their characteristic white plumage and mastering their flight skills. The albatrosses spend a significant portion of their juvenile years at sea, honing their flying abilities and exploring vast oceanic territories. It is during this period that they face various challenges, including encounters with other seabirds and potential threats from human activities.
It is this lengthy growth period that contributes to the vulnerability of this species and its slow population recovery. The Wandering Albatross faces numerous threats, including habitat loss, climate change, and accidental capture in fishing gear. Conservation efforts are crucial to ensure the survival of these magnificent birds and their unique life cycle.
The Wandering Albatross’s Diet and Hunting Techniques
Preferred prey and hunting grounds.
The Wandering Albatross is primarily a scavenger, feeding on a variety of marine organisms, including squid, fish, and crustaceans. They use their keen eyesight to spot potential prey items floating on the ocean surface, and once sighted, they plunge-dived from great heights to capture their meal. Additionally, these birds are known to scavenge carrion and exploit fishing vessels for an easy meal.
Adaptations for Hunting in the Open Ocean
Surviving in the harsh oceanic environment requires specialized adaptations, and the Wandering Albatross is well-equipped for the task. Its long wings enable it to glide effortlessly for long periods, conserving energy during hours of flight. The bird’s keen sense of smell allows it to locate food sources, even from great distances. These adaptations make the Wandering Albatross a formidable hunter and a vital component of the oceanic ecosystem.
Threats and Conservation Efforts
Human impact on the wandering albatross.
Despite their grace and beauty, Wandering Albatrosses face numerous threats that have contributed to their decline. One of the main challenges is the destructive impact of longline fishing operations, where the birds mistakenly become hooked or tangled in the fishing gear. Additionally, pollution, habitat degradation, and climate change further jeopardize the survival of these birds.
Current Conservation Strategies and Their Effectiveness
To safeguard the future of the Wandering Albatross, concerted conservation efforts are underway. Several measures have been implemented, including the establishment of protected areas and marine reserves, the development of guidelines for responsible fishing practices, and public awareness campaigns to promote the importance of nurturing this iconic species. While progress has been made, continued efforts are required to ensure the recovery and long-term survival of the Wandering Albatross.
The Role of the Wandering Albatross in Culture and Literature
Symbolism and significance in various cultures.
Throughout history, the Wandering Albatross has held deep cultural significance in many communities. In some cultures, these birds are considered symbols of loyalty, freedom, and endurance. They are often associated with seafaring traditions and are believed to bring good fortune to sailors.
The Albatross in Classic and Contemporary Literature
The haunting imagery of the Wandering Albatross has inspired numerous works of literature. Perhaps the most famous reference is found in Samuel Taylor Coleridge’s poem, “The Rime of the Ancient Mariner,” where an albatross is depicted as a harbinger of both good and ill fortune. Furthermore, many modern authors have woven the essence of the Wandering Albatross into their stories, capturing its mystique and its role as a symbol of the natural world.
In conclusion, the Wandering Albatross is a remarkable bird with a captivating presence. From its unique characteristics to its adaptations for survival in the open ocean , this magnificent creature enthralls all who encounter it. However, its existence is threatened by human activities and environmental changes. Through ongoing conservation efforts and a deeper appreciation of its cultural significance, we can work towards ensuring a future where the Wandering Albatross continues to grace the skies above the vast southern oceans.
Related articles
- Fresh Food for Cats – The 15 best products compared
- The Adorable Zuchon: A Guide to This Cute Hybrid Dog
- Exploring the Unique Characteristics of the Zorse
- Meet the Zonkey: A Unique Hybrid Animal
- Uncovering the Secrets of the Zokor: A Comprehensive Overview
- Understanding the Zebu: An Overview of the Ancient Cattle Breed
- Uncovering the Fascinating World of Zebrafish
- Watch Out! The Zebra Spitting Cobra is Here
- The Fascinating Zebra Tarantula: A Guide to Care and Maintenance
- The Yellow-Bellied Sapsucker: A Closer Look
- Uncovering the Mystery of the Zebra Snake
- The Amazing Zebra Pleco: All You Need to Know
- Discovering the Fascinating Zebra Shark
- Understanding the Impact of Zebra Mussels on Freshwater Ecosystems
- Caring for Your Zebra Finch: A Comprehensive Guide
- The Fascinating World of Zebras
- The Adorable Yorkshire Terrier: A Guide to Owning This Lovable Breed
- The Adorable Yorkie Poo: A Guide to This Popular Dog Breed
- The Adorable Yorkie Bichon: A Perfect Pet for Any Home
- The Adorable Yoranian: A Guide to This Sweet Breed
- Discover the Deliciousness of Yokohama Chicken
- Uncovering the Mystery of the Yeti Crab
- Catching Yellowtail Snapper: A Guide to the Best Fishing Spots
- The Brightly Colored Yellowthroat: A Guide to Identification
- Identifying and Dealing with Yellowjacket Yellow Jackets
- The Yellowish Cuckoo Bumblebee: A Formerly Endangered Species
- The Yellowhammer: A Symbol of Alabama’s Pride
- The Benefits of Eating Yellowfin Tuna
- The Yellow-Faced Bee: An Overview
- The Majestic Yellow-Eyed Penguin
- The Yellow-Bellied Sea Snake: A Fascinating Creature
- The Benefits of Keeping a Yellow Tang in Your Saltwater Aquarium
- The Beautiful Black and Yellow Tanager: A Closer Look at the Yellow Tanager
- The Fascinating Yellow Spotted Lizard
- What You Need to Know About the Yellow Sac Spider
- Catching Yellow Perch: Tips for a Successful Fishing Trip
- The Growing Problem of Yellow Crazy Ants
- The Rare and Beautiful Yellow Cobra
- The Yellow Bullhead Catfish: An Overview
- Caring for a Yellow Belly Ball Python
- The Impact of Yellow Aphids on Agriculture
- Catching Yellow Bass: Tips and Techniques for Success
- The Striking Beauty of the Yellow Anaconda
- Understanding the Yarara: A Guide to This Unique Reptile
- The Yakutian Laika: An Overview of the Ancient Arctic Dog Breed
- The Fascinating World of Yaks: An Introduction
- Everything You Need to Know About Yabbies
- The Xoloitzcuintli: A Unique Breed of Dog
- Uncovering the Mystery of Xiongguanlong: A Newly Discovered Dinosaur Species
- Uncovering the Mysteries of the Xiphactinus Fish
- Camp Kitchen
- Camping Bags
- Camping Coolers
- Camping Tents
- Chair Rockers
- Emergency Sets
- Flashlights & Lanterns
- Grills & Picnic
- Insect Control
- Outdoor Electrical
- Sleeping Bags & Air Beds
- Wagons & Carts
- Beds and furniture
- Bowls and feeders
- Cleaning and repellents
- Collars, harnesses and leashes
- Crates, gates and containment
- Dental care and wellness
- Flea and tick
- Food and treats
- Grooming supplies
- Health and wellness
- Litter and waste disposal
- Toys for cats
- Vitamins and supplements
- Dog apparel
- Dog beds and pads
- Dog collars and leashes
- Dog harnesses
- Dog life jackets
- Dog travel gear
- Small dog gear
- Winter dog gear
© Copyright 2024 | Imprint | Privacy Policy | About us | How we work | Editors | Advertising opportunities
Certain content displayed on this website originates from Amazon. This content is provided "as is" and may be changed or removed at any time. The publisher receives affiliate commissions from Amazon on eligible purchases.
January 12, 2024
How Does the World’s Largest Seabird Know Where to Fly?
Wandering albatrosses navigate thousands of miles using “the voice of the sea.”
By Joseph Polidoro
A pair of wandering albatrosses.
Getty Images/Imazins
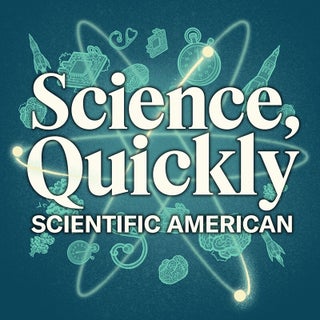
Joseph Polidoro: Imagine for a moment that you’re a very hungry bird soaring over 30-foot ocean swells in high winds, with no land for thousands of miles.
How do you know where you’re going?
If you’re a wandering albatross, you listen .
On supporting science journalism
If you're enjoying this article, consider supporting our award-winning journalism by subscribing . By purchasing a subscription you are helping to ensure the future of impactful stories about the discoveries and ideas shaping our world today.
[CLIP: Music]
According to a new finding in October’s Proceedings of the National Association of Sciences USA, this seabird navigates using sounds below our thresholds for hearing .
For Science, Quickly, I’m Joseph Polidoro.
The wandering albatross thrives in the circumpolar band of ocean north of Antarctica—a windswept region that the world’s best sailors say has the most inhospitable seas on the planet.
On the Southern Ocean’s islands where they nest and brood, one wandering albatross parent tends the nest while its partner takes to the sea, traveling as much as 10,000 kilometers as it forages for scattered prey. The bird must eat enough to fuel its turn on the nest, which can be a long time ...
Samantha Patrick: Birds might go for, perhaps, a minimum of four or five days, up to 30 days.
Polidoro: Samantha Patrick is a marine ecologist at the University of Liverpool in England and a co-author of the study.
Wandering albatrosses actually gain weight on these long trips because they’re extremely efficient flyers.
Sophie de Grissac: It almost never beats its wings. It’s quite fascinating to see them flying in the winds. When they’re flying, their heartbeat is the same as when they’re resting.
Polidoro: That’s Sophie de Grissac , an ornithologist and a researcher at the French National Museum of Natural History in Paris, who wasn’t involved in the study.
With their long wingspan—the longest of any bird, maxing out at nearly 12 feet—wandering albatrosses use wind, air pressure gradients, and gravity above the swells and waves to soar for thousands of miles, reaching top speeds of 45 miles an hour .
Basically, wandering albatrosses don’t fly. They soar.
De Grissac: The more distance you cover, the more you may find food.
Polidoro: The wandering albatross’s keen senses of sight and smell help it locate prey. But these senses are good for about 100 kilometers—a distance the bird can travel in as little as an hour and a half. So how does the albatross know where to soar toward?
Patrick: There does seem to be this large gap in information that they’re able to access.
Polidoro: A clue came in a chance encounter on the way to the Crozet Islands, part of the French Southern and Antarctic Territories, where Patrick was headed to study albatrosses.
Patrick: On the same vessel were some researchers from the [United Nations]. They were going to work with the hydrophone station that’s used to monitor nuclear tests. It also gathers infrasound data. And we came up with the question of whether seabirds could use infrasound. And it was clear that no one had really thought about this before, and that’s where the idea for the project came from.
Polidoro: Infrasound is any sound below 20 hertz, where human hearing starts to drop off. At the very low end of the infrasound spectrum are microbaroms—very low-frequency sounds between 0.1 and 0.6 Hz that are detectable across thousands of miles.
Natasha Gillies: Microbaroms are generated by the collision of ocean waves.
Polidoro: Natasha Gillies is a seabird ecologist at the University of Liverpool and a co-author of the study.
The constant hum of microbarom infrasound is called “the voice of the sea.” It’s present everywhere, all the time. But it’s unevenly distributed.
Gillies: Where you have more energy in the ocean system because you have wavier areas or windy areas, then you get louder microbarom regions.
Polidoro: Ideal soaring conditions for wandering albatrosses.
Patrick: But it also gives them information about standing ocean waves, and this is often caused by things like storms. So it would enable birds to try and gauge where storms are, potentially. So this might be be cause they want to move toward windier areas that could be optimal, or they might want to move away from windy areas if they’re too strong, and they want to try and avoid storms.
Polidoro: Directly testing this apex predator’s hearing is not an option. So Natasha and her colleagues arrived at a creative experimental solution: Get a large enough sample of wandering albatross flight paths. Then, using wind and infrasound data, create a sound map of the total flight area—a map of microbaroms across space and time. Send out another set of albatrosses equipped with sensors to field check the sound map. Finally, overlay the birds’ flight paths on the sound map.
Gillies: So essentially what we can get is: if you put an albatross at point X in space and on this day in time, what infrasound would it be likely to hear and experience?
Patrick: We didn’t have an expectation at the beginning that they would move toward louder or quieter areas.
Polidoro: What the team found is that wandering albatrosses aren’t exactly wandering. Instead they seem to use microbaroms to head toward ideal wind conditions.
Ventura: Looking at the soundscape and how the birds move, you know, almost following this wave of sound, I found that beautiful.
My name is Francesco Ventura , and I’m a postdoc at Woods Hole Oceanographic Institution.
Polidoro: He wasn’t involved in the study either.
Ventura: It’s another world–that’s the thing. It’s something that we cannot fully understand, I think; we are humans and we just cannot even imagine how that would work for us. But it seems to be working fine for them because they have been doing it for a long time.
They seem to be [reading] what’s going on and kind of orienting toward that. You know that is something that is…it’s SciFi.
Gillies: We know that there is something about infrasound that they want to move toward, that they like, that is beneficial to them in some way.
Ventura: It was kind of a badly needed paper at this point because it sheds some new light into a fundamental question that is at the core of a lot of marine megafauna research in general but also at the core of seabird research, which is: “How do they manage to find food in such a vast area?”
Polidoro: This reliance on infrasound may actually extend to other species, too.
Gillies: Most seabirds are highly dependent on wind for movement. It seems to be involved in animal behavior in a lot of contexts, in a lot of different species.
Polidoro: They include whales, elephants, pigeons and peacocks.
Gillies: So I would be very surprised if this was in any way unique to wandering albatrosses.
De Grissac: So albatrosses have had a very long time to evolve ways of feeling the environment—lots of ways they can perceive what’s around them. And I think because they really need this condition, this stormy conditions, these winds, it makes perfect sense that it would have evolved more than one way of finding them.
Gillies: I think it’s a really nice reminder of the different sources of information animals might be using—especially in this sort of environment that is so featureless—and how animals can still extract so much information and context out of that despite there seemingly not being much there.
De Grissac: Evolution in animals is almost always very surprising. When you study the evolution of the animal closely, you find remarkable things, remarkable inventions.
Polidoro: Science, Quickly is produced by Tulika Bose and Jeff DelViscio. Our music is composed by Dominic Smith.
Subscribe to Science, Quickly wherever you get your podcasts. If you like the show, give us a rating or review.

Smithsonian Ocean
Wandering albatross.

A wandering albatross has the largest wingspan of any bird, 3.5 meters (11.5 feet) tip to wing tip.
- Make Way for Whales
- Sharks & Rays
- Invertebrates
- Plants & Algae
- Coral Reefs
- Coasts & Shallow Water
- Census of Marine Life
- Tides & Currents
- Waves, Storms & Tsunamis
- The Seafloor
- Temperature & Chemistry
- Ancient Seas
- Extinctions
- The Anthropocene
- Habitat Destruction
- Invasive Species
- Acidification
- Climate Change
- Gulf Oil Spill
- Solutions & Success Stories
- Get Involved
- Books, Film & The Arts
- Exploration
- History & Cultures
- At The Museum
Search Smithsonian Ocean
Wandering Albatross
These remarkably efficient gliders, named after the Greek hero Diomedes, have the largest wingspan of any bird on the planet
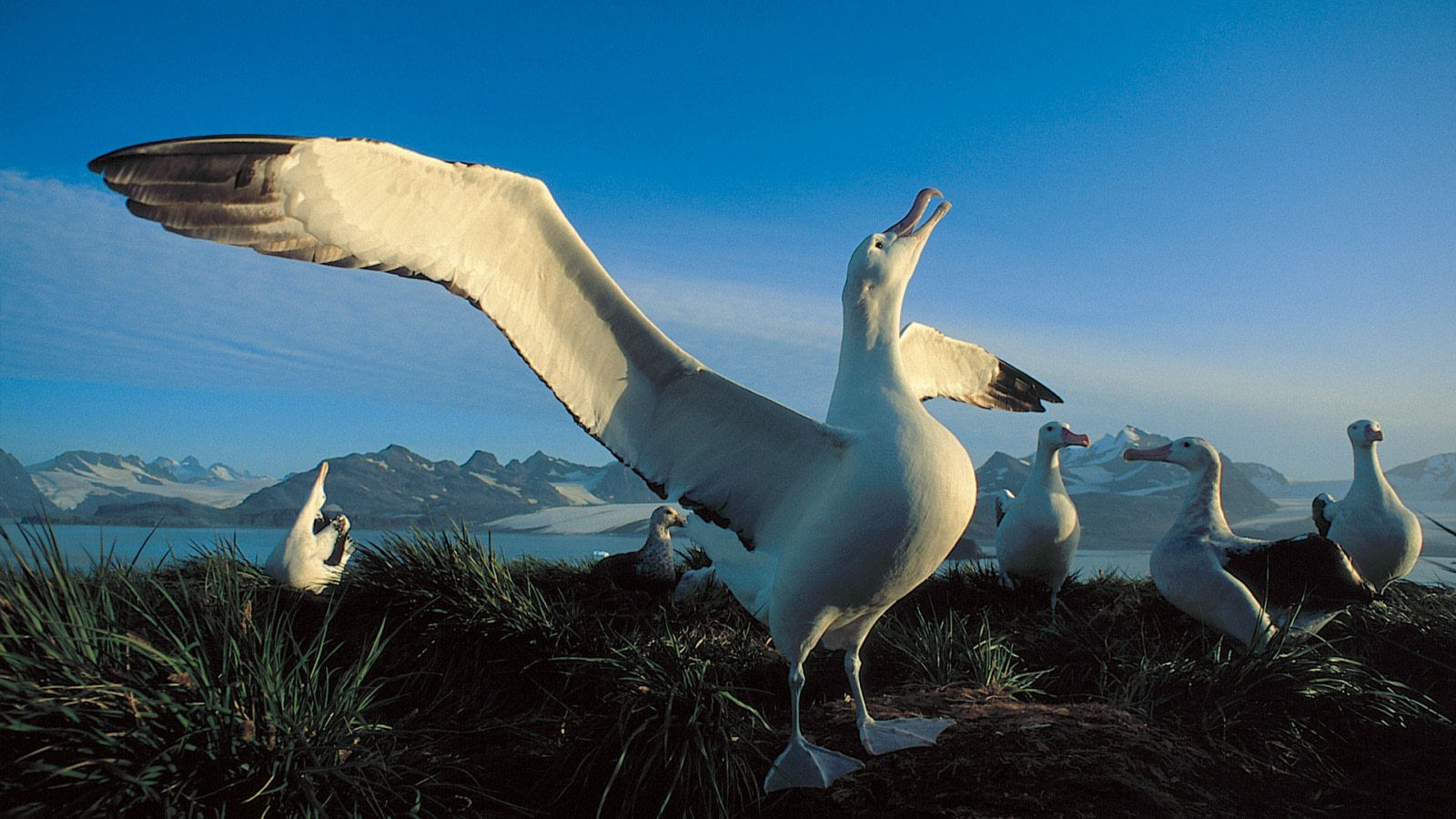
Region: Antarctica
Destinations: Bouvet Island, Antarctic Peninsula, South Georgia
Name : Wandering Albatross, Snowy Albatross, White-winged Albatross ( Diomedea exulans )
Length: Up to 135 cm.
Weight : 6 to 12kg.
Location : All oceans except in the North Atlantic.
Conservation status : Vulnerable.
Diet : Cephalopods, small fish, crustaceans.
Appearance : White with grey-black wings, hooked bill.
How do Wandering Albatrosses feed?
Wandering Albatrosses make shallow dives when hunting. They’ll also attempt to eat almost anything they come across and will follow ships in the hopes of feeding on its garbage. They can gorge themselves so much that they become unable to fly and just have to float on the water.
How fast do Wandering Albatrosses fly?
Wandering Albatrosses can fly up to 40 km per hour.
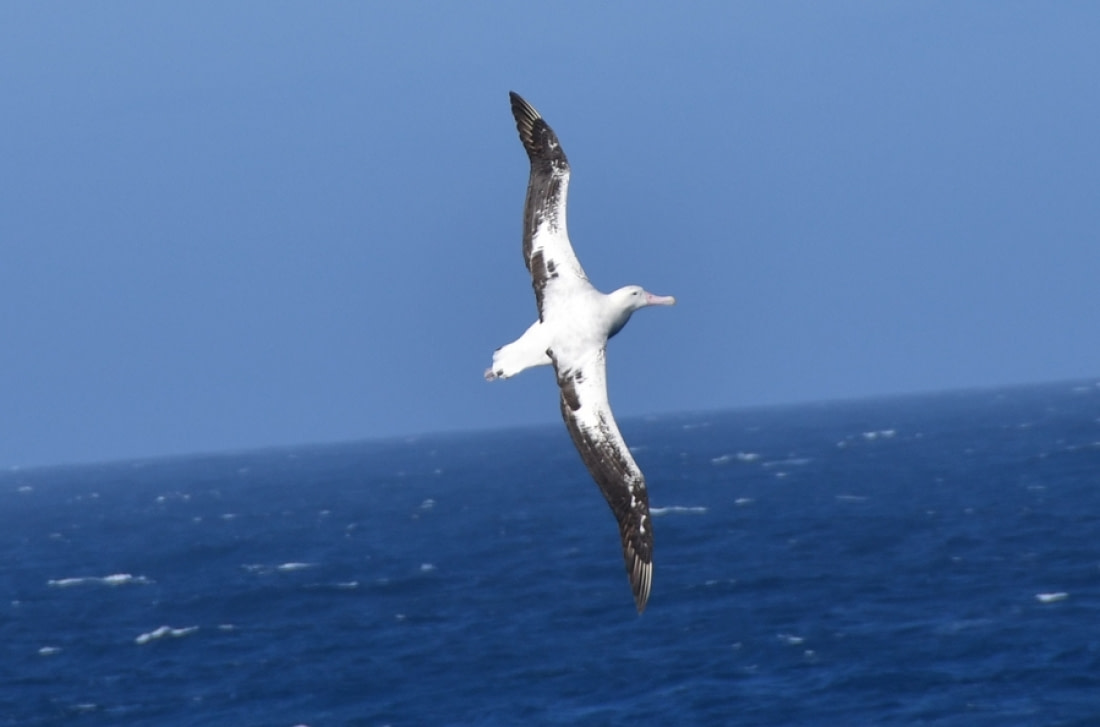
What are Wandering Albatross mating rituals like?
Wandering Albatrosses mature sexually around 11 years of age. When courting, the male Wandering Albatross will spread his wings, wave his head around, and rap his bills against that of the female while making a braying noise. The pair will mate for life, breeding every 2 years. Mating season starts in early November with the Albatrosses creating nests of mud and grass on one of the Sub-Antarctic islands. The female will lay 1 egg about 10 cm long, sometime between the middle of December and early January. Incubation takes around 11 weeks, the parents taking turns. Once the chick is born the adults switch off between hunting and staying to care for the chick. The hunting parent returns to regurgitate stomach oil for the chick to feed on. Eventually both parents will start to hunt at the same time, visiting with the chick at widening intervals.
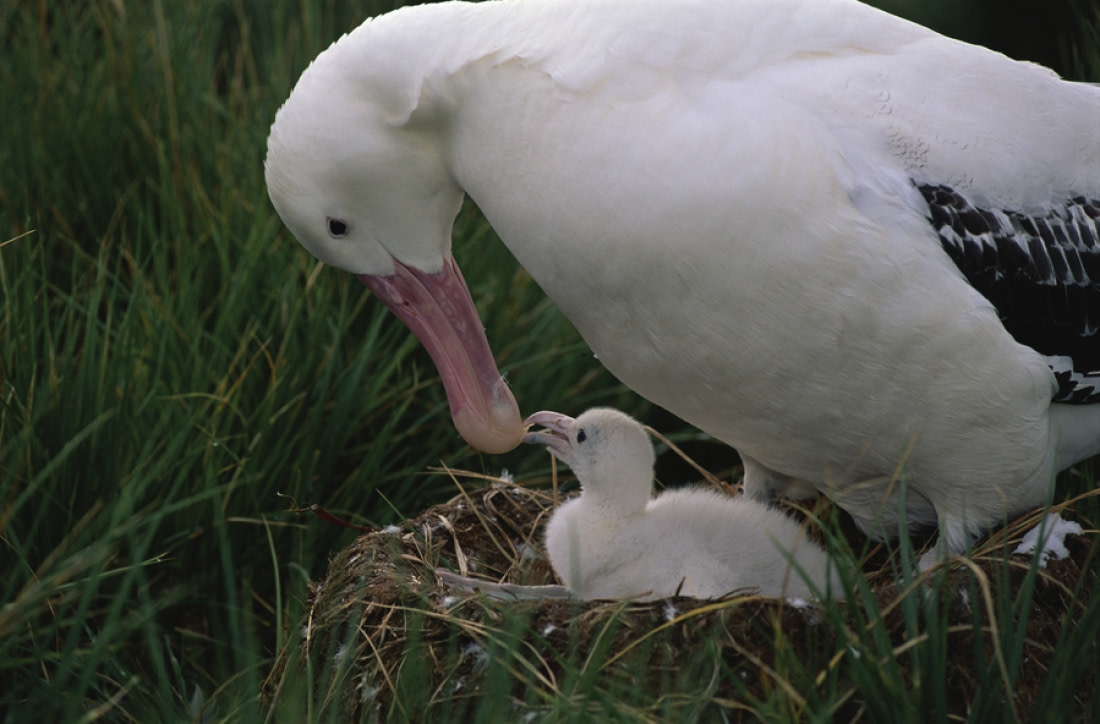
How long do Wandering Albatrosses live?
Wandering Albatrosses can live for over 50 years.
How many Wandering Albatrosses are there today?
There are about 25.200 adult Wandering Albatrosses in the world today.
Do Wandering Albatrosses have any natural predators?
Because they’re so big and spend almost all of their lives in flight, Wandering Albatrosses have almost no natural predators.
7 Wonderful Wandering Albatross Facts
- The Wandering Albatross is the largest member of its genus ( Diomedea ) and is one of the largest birds in the world.
- Wandering Albatrosses are also one of the best known and most studied species of birds.
- Diomedea refers to Diomedes, a hero in Greek mythology; of all the Acheaens he and Ajax were 2 nd only to Achilles in prowess. In mythology all of his companions turned into birds. Exulans is Latin for “exile” or “wanderer.”
- Wandering Albatrosses have the largest wingspan of any bird in the world today, stretching up to 3.5 metres across.
- Wandering Albatrosses are great gliders – they can soar through the sky without flapping their wings for several hours at a time. They’re so efficient at flying that they can actually use up less energy in the air than they would while sitting in a nest.
- Wandering Albatrosses have a special gland above their nasal passage that excretes a high saline solution. This helps keep salt level in their body, combating all the salt water they take in.
- Wandering Albatrosses get whiter the older they get.
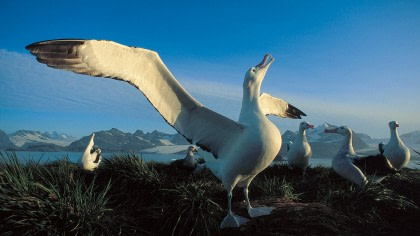
Related cruises
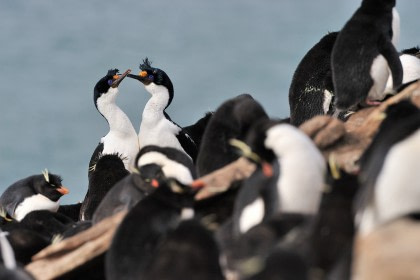
Falkland Islands - South Georgia - Antarctica
Meet at least six penguin species!
PLA20-24 A cruise to the Falkland Islands, South Georgia & the Antarctic Peninsula. Visit some of the most beautiful arrays of wildlife on Earth. This journey will introduce you to at least 6 species of penguin and a whole lot of Antarctic fur seals!
m/v Plancius
Cruise date:
18 Oct - 7 Nov, 2024
Berths start from:
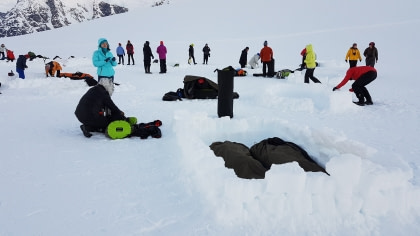
Antarctica - Basecamp - free camping, kayaking, snowshoe/hiking, photo workshop, mountaineering
The best activity voyage in Antarctica
HDS21a24 The Antarctic Peninsula Basecamp cruise offers you a myriad of ways to explore and enjoy the Antarctic Region. This expedition allows you to hike, snowshoe, kayak, go mountaineering, and even camp out under the Southern Polar skies.
m/v Hondius
1 Nov - 13 Nov, 2024
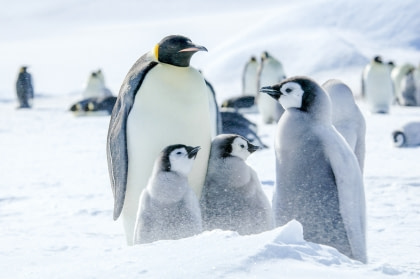
Weddell Sea – In search of the Emperor Penguin, incl. helicopters
Searching for the Elusive Emperor Penguins
OTL22-24 A true expedition, our Weddell Sea cruise sets out to explore the range of the Emperor Penguins near Snow Hill Island. We will visit the area via helicopter and see a variety of other birds and penguins including Adélies and Gentoos.
m/v Ortelius
10 Nov - 20 Nov, 2024
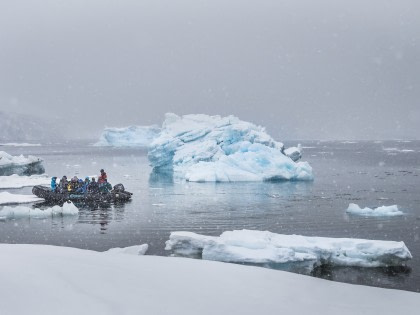
OTL23-24 A true expedition, our Weddell Sea cruise sets out to explore the range of the Emperor Penguins near Snow Hill Island. We will visit the area via helicopter and see a variety of other birds and penguins including Adélies and Gentoos.
20 Nov - 30 Nov, 2024
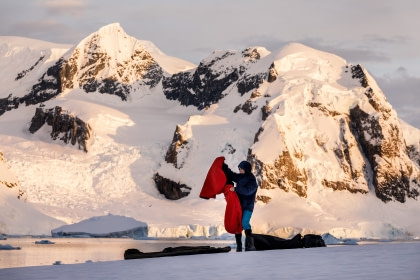
Antarctica - Basecamp - free camping, kayaking, snowshoe/hiking, mountaineering, photo workshop
HDS23-24 The Antarctic Peninsula Basecamp cruise offers you a myriad of ways to explore and enjoy the Antarctic Region. This expedition allows you to hike, snowshoe, kayak, go mountaineering, and even camp out under the Southern Polar skies.
23 Nov - 5 Dec, 2024
We have a total of 62 cruises
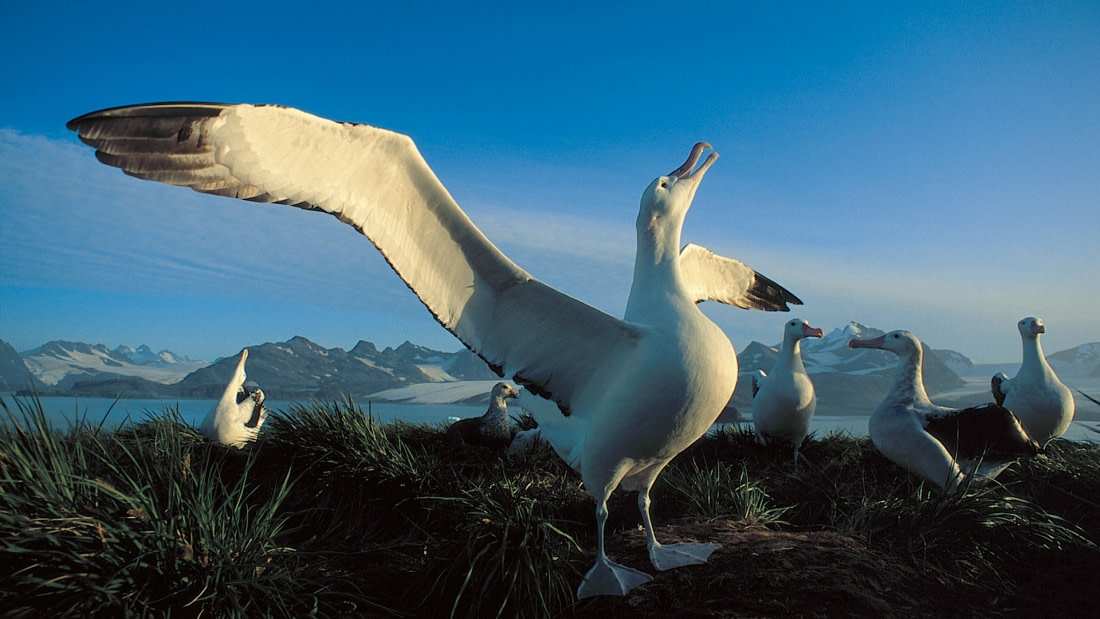
- Frogs in USA
- Bats in USA
- Lizards in USA
- Turtles in USA
- Hawks, Eagles, and Falcons in USA
- Birds in USA
- Woodpeckers in USA
- Hummingbirds in USA
- Owls in USA
- Hummingbird
- Desert Birds
- Colorful Birds
- Fastest Birds
- Birds of Prey
- Dangerous Birds
- Birds That Lay Blue Eggs
- Birds Around the World
- Birds That Sing at Night
- Birds by Color
- Crested Birds
- Alpine Birds
- Smartest Birds
- Herbivorous Birds
- Antarctic Birds
- Arctic Birds
- Poisonous Birds
- Longest Living Birds
- Birds That Mate For Life
- Long-Legged Birds
- Long-tailed Birds
- Diving Birds
- Birds That Eat Mosquitoes
- Fish-eating Birds
- Mountain Birds
- Small Birds
- Whistling Birds
- Nocturnal Birds
- Grasshopper
- South American
- North American
- Sonoran Desert
- Live in Lakes
- Deciduous Forest
- Temperate Forest
- Small Animals
- Hybrid Animals
- Rare Animals
- Monogamous Animals
- Animals that are Carnivorous
- Amazon Rainforest
- Death Valley
- Galápagos Islands
- Animals with Horns
- Animals with Antlers
- Camouflage Animals
- Ice Age Animals
- Animals that Migrate
- Animals with Big Eyes
- Endangered Animals
- Animals that are Omnivorous
- Animals You Can See On a Safari
- Animals Living in the Mariana Trench
- Animals with Long Necks
- Ugly Animals
- Smartest Animals
- Flying Animals
- Dumbest Animals
- Biggest Animals in the World
- Animals that Hibernate
- Fastest Animals in the World
- Hoofed Animals
- Animals that are Herbivorous
- Fluffy Animals
- Extinct Animals
- Melanistic Animals
- Longest Living Animals
- Animals That Mate For Life
- Ruminant Animals
- Scary Animals
- Poisonous Animals
- Colorful Animals
- Asexual Animals
- Animals that Burrow
- Fat Animals
- Dangerous Animals
- Slow Animals
- Nocturnal Animals
- Strong Animals
- Gay Animals
- Weird Animals
- Black Birds in Florida
- Beautiful Animals
- Animals That Lay Eggs (Oviparous Animals)
- Animals Living in Death Valley
- Yellowstone National Park
- Domestic Animals
- Land Animals
- Animals That Kill the Most Humans
Wandering Albatross
Table of Contents
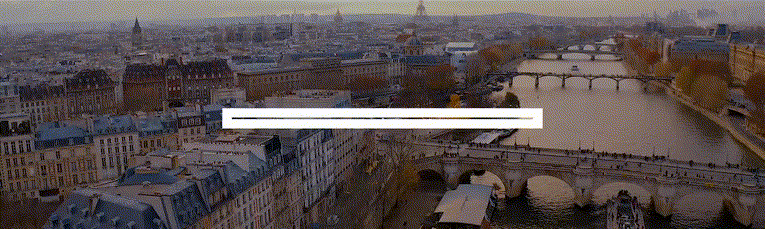
Scientific Classification
Table of content.
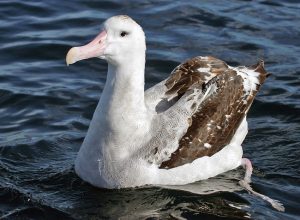
Physical Description
Size : They measure at around 3 ft 6 in to 4 ft 5 in (1.07-1.35 m).
Weight : Adult wandering albatrosses typically weigh between 13 and 28 lbs (5.9-12.7 kg).
Color : The plumage for juveniles is chocolate brown which becomes whiter with age. The wings in adults are white with black around the tips while the female’s wings have more black on them. The bill and feet are pink.
Sexual Dimorphism : Males are a little bit larger than females.
Wingspan : They have the largest wingspan among birds , measuring at around 8 ft 3 in to 11 ft 6 in (2.51-3.5 m).
The two recognized subspecies of the wandering albatross are D. e. exulans (nominate subspecies) and the D. e. gibsoni (also known as Gibson’s albatross).
Distribution
The breeding range for the wandering albatross includes South Georgia Island, Crozet Islands, Prince Edward Islands, Kerguelen Islands, and Macquarie Islands. It also feeds around the Kaikoura Peninsula on New Zealand’s South Island east coast.
They inhabit subantarctic islands with tussock grass, sedges, shrubs, mosses and peat soils. They nest on ridges, plateaus, valleys, and plains.
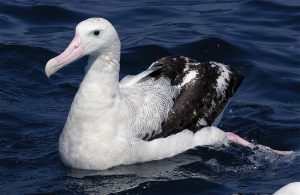
Wandering Albatross Pictures
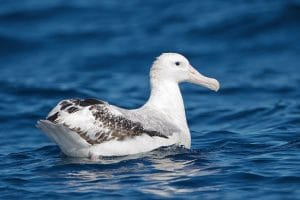
Wandering Albatross Images
- These birds spend most of their lives in the air, traveling long distances.
- They live in small groups during their forages in the sea.
- They become rather social during the breeding season.
- They are territorial towards members of the same sex during the breeding season and defend their nesting area with vocalizations.
Wandering albatrosses eat fish, squids, and crustaceans.
Mating & Reproduction
These birds mate for life and mate every other year. Males reach the breeding grounds before females and locate the same nesting sites they had used the previous season, although they may also choose to build new ones. Females arrive after males. The breeding season usually occurs between December and March. The female lays one egg per breeding season which is then incubated for 74-85 days. Both parents take part in incubation.
The hatchling stays in its parents’ care for up to 9 months of age, after which they achieve independence. They reach sexual maturity by the time they are 9 years old.
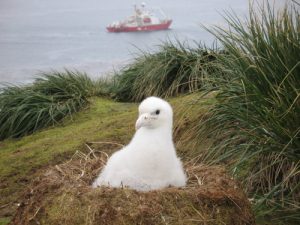
Wandering Albatross Chick
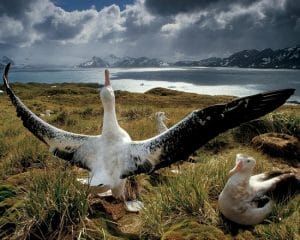
Wandering Albatross Size
Wandering albatrosses can live for up to 50 years.
Sounds & Communication
These birds communicate by croaking, bill-clapping, bill-touching, trumpeting, and pointing towards the sky with their bills.
Adaptations
- The large wings of the wandering albatross help them fly for vast distances over several hours without flapping. For every meter of drop in altitude, they can travel 22 meters in distance.
- The salt gland at the nasal passage helps them desalinate their bodies of the excess salt they come in contact with because of their oceanic lifestyle.
- They can dive up to a meter into the ocean to catch their prey. They, however, prefer to catch the fish from the surface of the ocean.
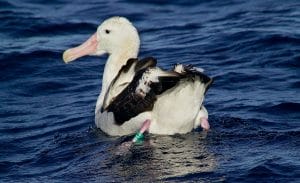
The Wandering Albatross
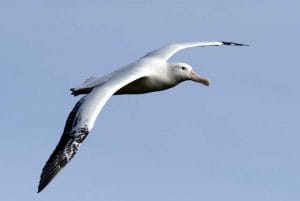
Wandering Albatross Flying
Adult wandering albatrosses have no predators. Eggs, hatchlings, and juveniles, on the other hand, are preyed upon by sheathbills and skuas. In addition to these two, several introduced animals like goats, pigs, rats, mice, and cats also eat the chicks and eggs.
IUCN Conservation Status
The International Union for Conservation of Nature lists the wandering albatross under their ‘Vulnerable’ category.
Interesting Facts
- The wandering albatross is the biggest bird in its genera and one the largest in the world.
- One individual lived to be 60 years old in New Zealand. She was named ‘Grandma.’
- Another banded individual was recorded to have traveled 3,730 miles in just 12 days.
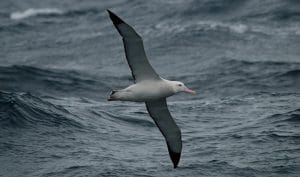
Wandering Albatross Wingspan
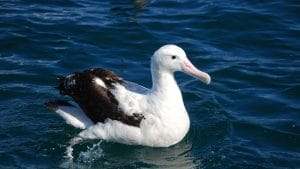
Wandering Albatross Bird
- http://www.coolantarctica.com/Antarctica%20fact%20file/wildlife/wandering-albatross.php https://oceanwide-expeditions.com/to-do/wildlife/wandering-albatross https://beautyofbirds.com/wandering-albatrosses/ http://animaldiversity.org/accounts/Diomedea_exulans/#ff4ee5a1ac2a7a07a049350b7c9b6fbc https://www.britannica.com/animal/albatross#ref243427 http://www.iucnredlist.org/details/22698305/0
Related Articles
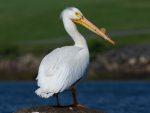
Leave a Reply Cancel reply
Your email address will not be published. Required fields are marked *
Recent Wallpapers
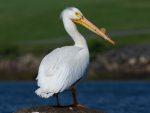
- Invertebrates
Subscribe our newsletter
Follow us on:.
- Privacy Policy
- Animal Habitats
- Animal Memes
© 2024 ( Animal Spot ). All rights reserved. Reproduction in whole or in part without permission is prohibited.
Large — Wandering and Royal —Albatrosses: Characteristics, Subspecies and Courtship
Home | Category: Sea Birds
LARGE ALBATROSSES
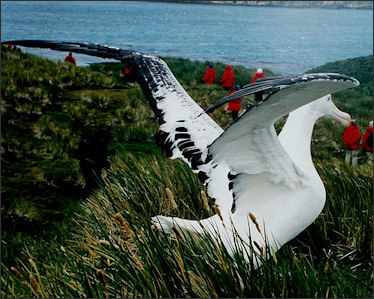
Considered to be the largest seabirds and among the largest of all birds capable of flight, Royal albatross and wandering albatrosses are predators at the top of the food chain. They engage in elaborate courtship displays and establish permanent pair bonds, Pairs devote a full year to the breeding cycle, in which a single chick is reared, then spend most of the next year on the high seas. fledglings catch gusts of wind, glide a few meters, then start again. These training sessions prepare them for flying over the open ocean. [Source: Canon advertisement]
Although humans formerly hunted albatrosses as food, adults currently have no predators. Their large size, sharp bill, and occasionally aggressive behavior make them undesirable opponents. However, some are inadvertently caught during large-scale fishing operations. Chicks and eggs, on the other hand, are susceptible to predation from skuas and sheathbills and introduced predators such as mice, pigs, cats, rats, and goats. Eggs that fall out of nests or are unattended are quickly preyed upon. Nests are frequently sheltered with plant material to make them less conspicuous. Small chicks that are still in the brooding stage are easy targets for large carnivorous seabirds.
Royal albatrosses and all subspecies of wandering albatrosses are highly vulnerable to becoming bycatch of commercial fisheries, and population declines are mostly attributed to this. Introduced predators such as feral cats, pigs, goats, and rats on various islands leads to high mortality rates of chicks and eggs. Conservation efforts have included the removal of introduced predators from islands and fishery relocation. /=\
See Separate Articles: SEA BIRDS: TYPES, MIGRATIONS AND THREATS ioa.factsanddetails.com ; ALBATROSSES: CHARACTERISTICS, NESTING AND THREATS ioa.factsanddetails.com
Websites and Resources: Animal Diversity Web (ADW) animaldiversity.org ; National Oceanic and Atmospheric Administration (NOAA) noaa.gov ; Encyclopedia of Life eol.org ; Smithsonian Oceans Portal ocean.si.edu/ocean-life-ecosystems ; MarineBio marinebio.org/oceans/creatures
Wandering Albatrosses
wandering albatross
Wandering albatrosses (Scientific name:Diomedea exulans) have the largest wings of any bird: three to 3.5 meters (10 to 11 feet) across. Because such enormous wings are difficult to flap rapidly they have difficulty taking off. Some launch themselves off cliffs. Others leap into head winds which provide them with lift. Crowded colonies often have a landing strip where the birds can land and takeoff, with birds cuing up like waiting planes at an airport. Taking off is particularly hard for young birds.
Wandering albatrosses are native to oceanic islands, the Indian Ocean, Atlantic Ocean and Pacific Ocean and are found almost exclusively in the Southern Hemisphere, although occasional sightings just north of the Equator have been reported. They are widely dispersed over the Southern Ocean and have been seen on coastal areas and islands of southern South America, southern Africa and southern Australia. In 2002, there were an estimated 8,500 pairs. [Source: Canon advertisement]
Wandering albatrosses breed on several remote oceanic and subantarctic islands, which are characterized by peat soils, tussock grass on hillocks, sedges, mosses, and shrubs. Wandering albatrosses nest in sheltered areas on plateaus, near ridges and on grassy plains or in valleys. Outside of the breeding season, wandering albatrosses are found only in the open ocean, where food is abundant. [Source: Lauren Scopel, Animal Diversity Web (ADW) /=]
Wandering and royal albatrosses are long-lived. A wandering albatross nicknamed "Grandma" was recorded to live over 60 years in New Zealand. Due to the late onset of maturity, with the average age at first breeding about 10 years, such longevity is not unexpected. However, there is fairly high chick mortality, ranging from 30 to 75 percent. Their slow breeding cycle and late onset of maturity make wandering albatrosses highly susceptible to population declines when adults are caught as bycatch in fishing nets. /=\
Wandering Albatross Characteristics
Wandering albatrosses range in length from 1.1 to 1.37 meters (3.6 to 4.5 feet). Their wingspan ranges from 2.5 to 3.5 meters (8.2 to 11.5 feet), with their average wingspan being 3.1 meters (10.2 feet). They weigh six to 11 kilograms (13 to 24 pounds), with their average weight being 8.1 kilograms (18 pounds). Their average basal metabolic rate is 20.3649 watts. Sexual dimorphism (differences between males and females is minimal: Both sexes are roughly equal in size and look similar but males are larger sexes are colored and patterned differently. [Source: Lauren Scopel, Animal Diversity Web (ADW) /=]
Wandering albatrosses are endothermic (use their metabolism to generate heat and regulate body temperature independent of the temperatures around them), homoiothermic (warm-blooded, having a constant body temperature, usually higher than the temperature of their surroundings) and have bilateral symmetry (both sides of the animal are the same). /=\
All subspecies of wandering albatrosses have extremely long wingspans, white underwing coverts, and pink bills. Adult body plumage ranges from pure white to dark brown, and the wings range from being entirely blackish to a combination of black with white coverts and scapulars. They are distinguished from the closely related royal albatross by their white eyelids, pink bill color, lack of black on the maxilla, and head and body shape. On average, males have longer bills, tarsi, tails, and wings than females. /=\
Juveniles of all subspecies are very much alike; they have chocolate-brown plumage with a white face and black wings. As individuals age, most become progressively whiter with each molt, starting with the back.
Wandering Albatross Subspecies
There is some disagreement over how many subspecies of wandering albatross there are, and whether they should be considered separate species. Most subspecies of wandering albatrosses are difficult to tell apart, especially as juveniles, but DNA analyses have shown that significant differences exist. [Source: Lauren Scopel, Animal Diversity Web (ADW) /=]
The main subspecies are: 1) Diomedea exulansexulans, which breeds on South Georgia, Prince Edward, Marion, Crozet, Kerguelen, and Macquarie islands; 2) Diomedea exulans dabbenena, which occurs on Gough and Inaccessible islands, ranging over the Atlantic Ocean to western coastal Africa; 3) Diomedea exulans antipodensis, which is found primarily on the Antipodes of New Zealand, and ranges at sea from Chile to eastern Australia; 4) Diomedea exulans. amsterdamensis, which is found only on Amsterdam Island and the surrounding seas. Other subspecies names that have become obsolete include A) Diomedea exulansgibsoni, now commonly considered part of D. e. antipodensis, and B) Diomedea exulansgibsoni chionoptera, considered part of D. e. exulans. /=\
1) D. e. exulans averages larger than other recognized subspecies, and is the only taxon that achieves fully white body plumage, and this only in males. Although females do not become pure white, they can still be distinguished from other subspecies by color alone. Adults also have mostly white coverts, with black only on the primaries and secondaries. 2) Adults of D. e. amsterdamensis have dark brown plumage with white faces and black crowns, and are distinguished from juveniles by their white bellies and throats. In addition to their black tails, they also have a black stripe along the cutting edge of the maxilla, a character otherwise found in D. epomophora but not other forms of Wandering albatrosses. Males and females are similar in plumage. /=\
3) Adults of D. e. antipodensis display Sexual Dimorphism (differences between males and females): in plumage, with older males appearing white with some brown splotching, while adult females have mostly brown underparts and a white face. Both sexes also have a brown breast band. 4) With age, D. e. dabbenena gradually attains white plumage, although it never becomes as white as male D. e. exulans. The wing coverts also appear mostly black, although there may be white patches. Females have more brown splotches than males, and have less white in their wing coverts. /=\
Wandering albatrosses exulans and Wandering albatrosses antipodensis are listed by the International Union for Conservation of Nature (IUCN) Red list and Birdlife International as being vulnerable; Wandering albatrosses dabbenena is listed as Endangered. Wandering albatrosses amsterdamensis is listed as critically Endangered due to introduced predators, risk of becoming bycatch, small population size, threat of chick mortality by disease, and loss of habitat to cattle farming
Wandering Albatross Behavior and Feeding
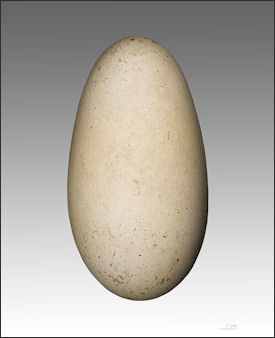
Wandering albatrosses defend small nesting territories. The average size of these territories is one square meters. Otherwise the range within which they travel is many thousands of square kilometers. While foraging at sea, wandering albatrosses travel in small groups. Large feeding frenzies may occur around fishing boats. Individuals may travel thousands of kilometers away from their breeding grounds, even occasionally crossing the equator. /=\
Wandering albatrosses sense using vision, touch, sound and chemicals usually detected with smell. They communicate with vision, touch and sound. During the breeding season, they are gregarious and displays are common Displays and vocalizations are common when defending territory or mating. They include croaks, bill-clapping, bill-touching, skypointing, trumpeting, head-shaking, the "ecstatic" gesture, and "the gawky-look". Individuals may also vocalize when fighting over food.
Wandering albatrosses primarily eat fish, such as toothfish, squids, other cephalopods, and occasional crustaceans. The primary method of feeding is by surface-seizing. They have the ability to plunge and dive up to one meter. They will sometimes follow fishing boats and feed on catches with other seabirds, which they usually outcompete because of their size.
Wandering Albatross Mating and Reproduction
Wandering albatrosses engage in seasonal breeding. Breeding occurs biennially, possibly annually if the previous season's attempt fails. The breeding season is from December through March. Pairs with chicks from the previous season co-exist in colonies with mating and incubating pairs. Pairs unsuccessful in one year may try to mate again in the same year or the next one, but their chances of successfully rearing young are low.[Source: Lauren Scopel, Animal Diversity Web (ADW) /=]
Wandering albatrosses are monogamous (have one mate at a time). They forming pair bonds for life. Females may bond temporarily with other males if their partner and nest are not readily visible. After foraging at sea, males arrive first at the same breeding site every year within days of each other. They locate and reuse old nests or sometimes create new ones. Females arrive later, over the course of a few weeks.
Copulation occurs in the austral summer, usually around December (February for D. e. amsterdamensis). Rape and extra-pair copulations are frequent, despite their monogamous mating strategy. Some individuals may reach sexual maturity by age six. Immature, non-breeding individuals will return to the breeding site. Group displays are common among non-breeding adults, but most breeding adults do not participate. /=\
Wandering Albatross Nesting and Young
courting wandering albatross male and female
For wandering albatrosses the average number of eggs per season is one. The time to hatching ranges from 74 to 85 days, with the fledging age ranging from seven to 10 months and the age in which they become independent ranging from seven to 10 months. Females and males reach sexual maturity at six to 22 years, with the average being 10 years. During the pre-fertilization stage provisioning and protecting is done by females. During the pre-birth stage provisioning is done by females and protecting is done by males and females. During the pre-weaning stage provisioning and protecting are done by females and males. During Pre-independence provisioning is by females and males. /=\
Pairs nest on slopes or valleys, usually in the cover of grasses or shrubs. Nests are depressions lined with grass, twigs, and soil. Males choose the nesting territory, and stay at the nest site more than females before incubation. Both parents incubate eggs and alternate during incubation. Although females take the first shift, males are eager to take over incubation and may forcefully push females off the egg.
Although there is generally equal parental investment, males tend to invest more as the chick nears fledging. Occasionally, a single parent may successfully rear its chick. Untended eggs are in danger of predation by skuas and sheathbills, After the chick hatches, they are brooded for about four to six weeks until they can be left alone at the nest. Males and females alternate foraging at sea. Following the brooding period, both parents leave the chick by itself while they forage. The chicks are entirely dependent on their parents for food for nine to 10 months, and may wait weeks for them to return. Chicks are entirely independent once they fledge. /=\
Royal Albatrosses
Royal albatrosses (Scientific name: Diomedea epomophora) live in temperate, tropical and polar saltwater-marine environments. They are found in coastal areas and in the open ocean far from land. Nearly 80 percent of a royal albatross' life is spent directly exposed to the cold, treacherous, open oceans of the Southern Hemisphere. Remote tropical islands are sought out for nesting. They typically nest on slopes with tussock grass providing some shelter, though exposed sites are also common as they ease the often difficult tasks of take-off and landing. [Source: Jason LaGosh, Animal Diversity Web (ADW) /=]

Royal albatross are remarkably long-lived when considering that the vast majority of their lives are spent over the perilous southern oceans. The adult mortality rate is three percent per year. In the wild, a royal albatross was known to have lived to over 58 years. It is possible that some birds may reach an age of 80 years. Due in part to their large size and solitary lifestyle, both in the air and on secluded islands, royal adult albatross have no known predators. Introduced predators such as cats and rats may be a threat to eggs and young.
Humans have been a threat in the past. Populations declined rapidly due to commercial fishing practices but recent, stricter penalties for killing royal albatross have helped populations remain stable and healthy. In New Zealand waters trawlers are required to replace outdated equipment and implement new, safer methods. Today, populations are estimated to be 10,000 to 20,000 pairs. Royal albatross are listed as 'Vulnerable' by the International Union for Conservation of Nature (IUCN). They have no special status according to the Convention on the International Trade in Endangered Species (CITES).
Feeding and Northern and Southern Royal Albatross
There are two subspecies of royal albatrosses. 1) Northern royal albatrosses (D. e. sanfordi) commonly nest on Campbell Island and the Auckland Islands. Southern royal albatrosses (D.e. epomophora) nest almost exclusively on the Chatham Islands, located hundreds of miles east of New Zealand. After breeding, the species may circumnavigate the Southern Ocean, though it is most commonly sighted in New Zealand and South American waters. It has never been recorded north of the Equator. /=\
Differences in the appearances of the two subspecies are minimal. Both are predominantly white, with faint pinkish bills. Northern royal albatrosses are considerably smaller and have entirely black upper wings. Southern royal albatrosses have predominantly white wings with black markings near the wing tips. Northern royal albatross can fly 1,800 kilometers in 24 hours.
The range of Royal albatrosses extends throughout the oceans of the Southern Hemisphere. They mainly eat squid such as the greater hooked squid and giant warty squid as well as fish such as blue grenadier and some crustaceans. Due to their lack of maneuverability, an albatross rarely picks up prey in flight. Instead, they sit on the water and use a method known as surface-seizing. Occasionally, they make shallow plunges. Most of their hunting, particularly for squid, is done at night.
Royal Albatross Characteristics, Flying and Behavior
Royal albatrosses have very long, slender, knife-like wings. They have an average weight of nine kilograms (20 pounds) and range in length from 1.1 to 1.2 meters (3.5 to 4 feet). Their wingspan ranges from three to 3.5 meters (10 to 11.5 feet), with their average wingspan being 3.25 meters (10.7 feet). Males are slightly larger than females.[Source: Jason LaGosh, Animal Diversity Web (ADW) /=]

Royal albatrosses are good gliders and are diurnal (active during the daytime), nocturnal (active at night), motile (move around as opposed to being stationary), nomadic (move from place to place, generally within a well-defined range) and solitary. It it estimated that they travel more than 163,000 kilometers (100,000 miles) each year, reaching speeds over 110 kilometers per hour (70 miles per hour). Royal albatross are generally solitary at sea. Sometimes they form large congregations when abundant food is present, particularly around fishing boats. Hundreds of older juveniles and birds without mates may gather on land in resting areas.
Because they are unable to sustain flapping flight, royal albatross cannot fly in calm weather. According to Animal Diversity Web: When winds drop, royal albatross may be forced to float in the oceans. Instead of flapping their wings, royal albatross glide, using the updrafts of air which are deflected upwards by the waves of the ocean. As majestic as royal albatross are in the sky, their methods for take-off and landing are anything but graceful. Royal albatross must take a long running start with their wings spread to take off. An element of danger exists in landing, particularly on solid ground. Without continued flapping, braking can be difficult. Often times, this results in crash landings, where injuries are not uncommon. /=\
Royal albatrosses communicate with vision, touch and sound and sense using vision, touch, sound and chemicals usually detected with smell. Elaborate displays are done by males and females to form pair-bonds. Actions like 'Bill-circling', 'Sky-pointing', 'Flank-touching' with the bill and the spreading of the wings are involved in courtship. These displays are typically accompanied by a variety of calls. This form of communal dancing usually takes place on land but on occasion it can occur at sea. Royal albatross are usually silent at sea but can become rather vocal when competing for food, especially around fish boats. Croaking, shrieking, and gargling sounds are the most common sounds made during competition for food. As a threat to intruders, a highly characteristic rattling sound can be produced by clappering the bill quickly and repeatedly. /=\
Royal Albatross Mating, Reproduction and Offspring
Royal albatrosses engage in seasonal breeding and breed every other year. The breeding season begins in October Without fail, only one egg is laid. Eggs weigh between 205 to 487 grams, about five to 11 percent of the body weight of the mother. The average time to hatching is 79 days. Chicks have white down and their coloration is similar to that of adults. The average fledging age is 240 days. At this time chicks simply flies off on their own. Females and males reach sexual maturity at 9 to 11 years. [Source: Jason LaGosh, Animal Diversity Web (ADW) /=]
Royal albatross are monogamous (have one mate at a time) and pair for life. 'Divorce' is unusual and under normal conditions only occurs after several failed breeding attempts, typically only death splits a pair. Young are altricial. This means that young are born relatively underdeveloped and are unable to feed or care for themselves or move independently for a period of time after birth. Pre-birth protection and pre-weaning provisioning and protecting are done by females and males.
According to Animal Diversity Web: Royal albatross have extensive and varied courtship displays that include actions like 'Bill-circling', 'Sky-pointing', 'Flank-touching' with the bill, and full spreading of the wings. In many cases, these rituals are done and a pair is formed in the season prior to breeding. An elaborate courtship is unnecessary for birds that have bred together in the previous year. Previously mated pairs usually use the same nest-site as the year before. Typically, the male arrives a few days before the female. A few greeting ceremonies are performed upon the arrival of the female, and shortly thereafter, they breed. Breeding is biennial (occurs every two years), due in part to the long incubation period. As a result, there is no replacement egg laying, forcing a pair to wait until the following season to re-nest if their egg is lost. /=\
After arriving to the nest-site first, the male defends the territory against other males and rebuilds or starts building a new nest while he waits for his partner. When the female arrives a few days later, the birds briefly display then copulate. Immediately afterwards, both return to sea where they feed and begin to build up a reserve of food. Both birds return to the nest shortly before the egg is laid. The female lays the egg then immediately retreats to the sea.
The male is left to incubate the egg until the female returns, sometimes leaving the male without food or water for two to three weeks. When the female returns to the nest, the male leaves to find food and regain his strength. This pattern continues until the egg hatches and the chick no longer needs to be brooded, this usually takes six weeks. At this point, both parents leave to find food but return daily to feed their chick a meal of partly digested fish, squid, and stomach oil that adults produce during the ordinary digestion of their food. The oil is rich in fats and helps provide the nutrients necessary for the chick to grow despite long spans without food. The growing chick wanders around the nest-site between visits, but must return to the nest to be fed. After a few brief failures, the chick simply flies away to start life on its own./=\
Image Sources: Wikimedia Commons, NOAA
Text Sources: Animal Diversity Web (ADW) animaldiversity.org ; National Oceanic and Atmospheric Administration (NOAA) noaa.gov ; Wikipedia, National Geographic, Live Science, BBC, Smithsonian, New York Times, Washington Post, Los Angeles Times, The New Yorker, Reuters, Associated Press, Lonely Planet Guides and various books and other publications.
Last Updated May 2023
- Google+
Page Top
This site contains copyrighted material the use of which has not always been authorized by the copyright owner. Such material is made available in an effort to advance understanding of country or topic discussed in the article. This constitutes 'fair use' of any such copyrighted material as provided for in section 107 of the US Copyright Law. In accordance with Title 17 U.S.C. Section 107, the material on this site is distributed without profit. If you wish to use copyrighted material from this site for purposes of your own that go beyond 'fair use', you must obtain permission from the copyright owner. If you are the copyright owner and would like this content removed from factsanddetails.com, please contact me.

An official website of the United States government
The .gov means it’s official. Federal government websites often end in .gov or .mil. Before sharing sensitive information, make sure you’re on a federal government site.
The site is secure. The https:// ensures that you are connecting to the official website and that any information you provide is encrypted and transmitted securely.
- Publications
- Account settings
Preview improvements coming to the PMC website in October 2024. Learn More or Try it out now .
- Advanced Search
- Journal List
- v.7(17); 2017 Sep
Early‐life foraging: Behavioral responses of newly fledged albatrosses to environmental conditions
Sophie de grissac.
1 Centre d'Etudes Biologiques de Chizé, CNRS & Université de La Rochelle, Villiers en Bois, France
Frederic Bartumeus
2 Centre for Advanced Studies of Blanes (CEAB‐CSIC), Girona, Spain
3 CREAF, Barcelona, Spain
4 Institució Catalana de Recerca i Estudis Avançats (ICREA), Barcelona, Spain
Henri Weimerskirch
Associated data.
All tracking data are available in the Dryad public archive at http://datadryad.org/ .
In order to survive and later recruit into a population, juvenile animals need to acquire resources through the use of innate and/or learnt behaviors in an environment new to them. For far‐ranging marine species, such as the wandering albatross Diomedea exulans , this is particularly challenging as individuals need to be able to rapidly adapt and optimize their movement strategies in response to the highly dynamic and heterogeneous nature of their open‐ocean pelagic habitats. Critical to this is the development and flexibility of dispersal and exploratory behaviors. Here, we examine the movements of eight juvenile wandering albatrosses, tracked using GPS /Argos satellite transmitters for eight months following fledging, and compare these to the trajectories of 17 adults to assess differences and similarities in behavioral strategies through time. Behavioral clustering algorithms (Expectation Maximization binary Clustering) were combined with multinomial regression analyses to investigate changes in behavioral mode probabilities over time, and how these may be influenced by variations in day duration and in biophysical oceanographic conditions. We found that juveniles appeared to quickly acquire the same large‐scale behavioral strategies as those employed by adults, although generally more time was spent resting at night. Moreover, individuals were able to detect and exploit specific oceanographic features in a manner similar to that observed in adults. Together, the results of this study suggest that while shortly after fledging juvenile wandering albatrosses are able to employ similar foraging strategies to those observed in adults, additional skills need to be acquired during the immature period before the efficiency of these behaviors matches that of adults.
1. INTRODUCTION
To survive, animals must either be familiar with their surrounding environment or, when facing unfamiliar conditions, able to learn and remember when and where resources can be found, alongside where they can hide from predators. In addition, a good knowledge of their environment may also influence the outcome of competitive interactions (Krebs, 1982 ; Sandell & Smith, 1991 ; Stamps, 1987 ). As such, the efficiency of exploratory behaviors has important consequences for individual survival (Baker, 1993 ; Verbeek, Drent, & Wiepkema, 1994 ), and thus individual and population fitness. This is particularly true for immature animals, which are foraging independently for the first time with little to no parental guidance. These individuals typically forage in an unknown environment, and thus rely on an innate ability to find and handle resources (Akesson & Weimerskirch, 2005 ; Alerstam, Hedenström, & Åkesson, 2003 ) alongside/or associations with congeners that they can copy (Fagan, Cantrell, Cosner, Mueller, & Noble, 2012 ; Mueller, O'Hara, Converse, Urbanek, & Fagan, 2013 ). Moreover, when compared to older individuals, inexperienced juveniles are generally less able to effectively apply crucial skills such as navigation, foraging, and predator avoidance (Marchetti & Price, 1989 ; Sergio et al., 2014 ; Thorup, Alerstam, Hake, & Kjellén, 2003 ). As such, their survival probabilities are usually much lower than those of adults (Clobert, Perrins, McCleery, & Gosler, 1988 ; Magrath, 1991 ; Naef‐Daenzer, Widmer, & Nuber, 2001 ; Perrin, 1979 ). In addition, they may be more vulnerable to sudden changes in habitat availability (e.g., anthropogenic disturbance and prey depletion/redistribution) alongside extreme climatic events (Nevoux, Weimerskirch, & Barbraud, 2007 ). As such, innate abilities for orientation and foraging cannot be the only mechanisms juveniles rely on to survive, and a certain amount of learning and adjustment is probably necessary in order to endure the critical period of early life and later recruit into a population.
In many seabird species, chicks are left alone at the nest by their parents before fledging. As such, they have to leave the colony and forage at sea independently without the opportunity to learn from their parents. Vital foraging skills are therefore likely learned quickly (within the first few months at sea). A large capacity for behavioral adaptation to environmental variability may also aid survival. Although immaturity can last several years (e.g., up to ten years for albatrosses), these first months at sea appear particularly critical to the survival of juvenile seabirds (Daunt, Afanasyev, Adam, Croxall, & Wanless, 2007 ; Horswill et al., 2014 ; Riotte‐Lambert & Weimerskirch, 2013 ). However, despite this, little is currently known about the detailed foraging tactics of animals during this time, alongside how individuals respond to environmental cues that aid in the acquisition of resources (Hazen et al., 2012 ; Lewison et al., 2012 ; Scales et al., 2014 ). In particular, there is a lack of quantitative analyses examining how juveniles survive, which is likely because observing young seabirds at sea is challenging.
Long‐ranging pelagic seabirds, such as albatrosses and petrels, forage on heterogeneously distributed prey in an environment with little physical constraints and a paucity of landmarks (Weimerskirch, 2007 ; Weimerskirch, Gault, & Cherel, 2005 ). Moreover, they may rely on particular environmental conditions to be efficient. For example, albatross and petrel flight is strongly influenced by wind, which is used to minimize corresponding energetic costs (Felicísimo, Muñoz, & González‐Solis, 2008 ; Pennycuick, 1982 ; Weimerskirch, Guionnet, Martin, Shaffer, & Costa, 2000 ). In addition, individuals from these species may forage at specific habitats, such as regions of elevated productivity, shelf slopes, ocean fronts, or oceanic waters with species‐specific temperature preferences where prey availability is enhanced (Hunt, 1991 ; Kappes, 2009 ; Louzao et al., 2011 ; Pinaud & Weimerskirch, 2007 ; Scales et al., 2016 ). However, the use of specific wind conditions and oceanographic habitat features by inexperienced juvenile albatrosses and petrels to optimize energy acquisition is unclear. Specifically, it is unknown whether individuals forage using strategies similar to those employed by older, more experienced adults as soon as they fledge, or whether they progressively acquire these with time.
The aim of this study was to examine the early foraging behaviors of juvenile wandering albatrosses Diomedea exulans during their first months at sea following fledging. We use a dataset of eight juveniles, tracked using GPS/Argos transmitters during their first eight months after leaving their natal island in the southern Indian Ocean, and compare this to the tracks of 17 adults from the same colony. Through the use of a behavioral clustering algorithm, we identify the main behavioral modes adopted by these animals when at sea and examine (1) whether the at‐sea behaviors of juveniles change through time after fledging, (2) to what extent behavioral mode is influenced by external conditions (e.g., light, oceanographic features, and climatic factors), and (3) whether juvenile behavioral modes differ from those of adults.
2.1. Fieldwork and telemetry
Fieldwork was conducted on the Crozet Islands, Southern Indian Ocean (46.2°S, 52.4°E), during the breeding seasons of 2013 (juveniles and adults), and 2014 and 2015 (for adults only). In 2013, 10 wandering albatross chicks (six males and four females), that were about to fledge, were fitted with GPS/Argos satellite transmitters (Platform Terminal Transmitter, PTT 100, Microwave Telemetry, Columbia, USA). These were attached to the central back feathers using adhesive tape (TESA ® ) and glue (Loctite ® ). GPS tags were programmed to record one location every two hours (accuracy ~20–75 m). These locations were then transmitted every three days by the Argos transmitters (which were powered by solar battery). The device weighed 65 g which is <1% of the average mass of the juvenile birds (10.3 ± 3.0 kg) and well below the limit recommended for flying birds (Phillips, Xavier, Croxall, & Burger, 2003 ). Individuals were sexed using a molecular sexing method (Weimerskirch, Lallemand, & Martin, 2005 ).
To compare juvenile behavior with that of adults, 17 breeding adults from the same colony were tracked with GPS transmitters during incubation from January to March (2013–2015 inclusive). This time period corresponds to the first three months of juvenile independence following fledging in late December. Adults were equipped with GPS tags using the methods described above. GPS tags recorded one location every 15 min. To be comparable to the data retrieved from juveniles, locations were later resampled at 2 hourly intervals.
2.2. Clustering of foraging behaviors
Expectation Maximization binary Clustering (EMbC; Garriga, Palmer, Oltra, & Bartumeus, 2016 ) was used to classify the behavioral modes adopted by an individual while at sea. The EMbC algorithm is a variant of Gaussian Mixture Model maximum‐likelihood estimation (or Expectation Maximum Clustering). It is a robust, nonsupervised multivariate clustering algorithm that considers the correlation and uncertainty of variables to give a meaningful local label that can be easily biologically interpreted. This includes a percentage uncertainty for each classification. Behaviors were categorized using two input variables: the velocity and turning angle of a bird between each location. Both parameters were calculated with loxodromic distances and bearings using the “geosphere” R package (Hijmans, 2015 ). Tracks were clustered into four behavioral categories: high velocity/low turning angle (HL), high velocity/high turning angle (HH), low velocity/low turning angle (LL) and low velocity/high turning angle (LH; Table 1 and detailed statistics in Table S1 ). HL and HH behaviors correspond to rapid movements which we have, respectively, termed “ballistic” (commuting phases, i.e., rapid speed and high directionality) and “diffusive” (sinuous exploratory phases at large scale using looping movements). LL and LH behaviors correspond to slow movements when the bird is mainly sitting on the water, and are referred to, respectively, as “resting” (bird drifting passively) and “active sitting.” The later of these, “active sitting,” reflects a mixture of different behavioral types, such as (1) the use of a sit‐and‐wait foraging strategy (Weimerskirch, Cherel, Cuenot‐Chaillet, & Ridoux, 1997 ), (2) intensive foraging on a prey patch using short flights interspersed with sitting bouts, and (3) the transition between the “resting” mode and one of the two rapid modes (“ballistic” and “diffusive”; i.e., when a bird takes off with a change of direction at the end of the two hours segment). These interpretations have been validated in earlier papers through the use of visual observations, activity logger analyses, and energetic budget analyses (Louzao, Weigand, Bartumeus, & Weimerskirch, 2014 ; Weimerskirch, Delord, Guitteaud, Phillips, & Pinet, 2015 ; Weimerskirch, Pinaud, Pawlowski, & Bost, 2007 ; Weimerskirch et al., 1997 , 2002 ). In the analyses performed here, we used only track portions labeled with 100% certainty by the algorithm (i.e., when the time interval used to compute velocity and turns was not superior to two hr).
Binary clusters as determined by the EMbC algorithm (Garriga et al., 2016 ) and their corresponding behavioral mode. Adapted from Louzao et al. ( 2014 )

2.3. Environmental variables
To study the relationship between behavioral mode and biophysical environmental conditions, we considered several environmental variables that are known to influence the foraging behaviors of marine predators elsewhere (Friedland et al., 2012 ; Hunt, 1991 ; Hunt et al., 1999 ; Hyrenbach, Veit, Weimerskirch, & Hunt, 2006 ; Louzao et al., 2011 ). These were as follows: six hourly wind velocity and direction (0.25° resolution), bathymetry (0.016° resolution), monthly chlorophyll‐α concentration (CHLa, 0.04° resolution), daily sea‐level height anomaly (SLHA, 0.25° resolution), and moon brightness. Bathymetry, wind, and CHLa data were downloaded from the NOAA coast watch website ( http://coastwatch.pfeg.noaa.gov ). The SLHA data were taken from the Aviso data portal and were produced by Ssalto/Duacs with support from CNES ( http://www.aviso.altimetry.fr/duacs/ ). Moon data were obtained via the “lunar” R package (Lazaridis, 2014 ) and used to compute a continuous moon brightness index by associating the height of the moon above the horizon with lunar phase. To aid interpretation, this index was also split into three categories corresponding to (1) “dark nights” when the moon was under the horizon and/or in its first quarter, (2) “half‐moon nights” when the moon was above the horizon and between its first and third quarter, and (3) “full‐moon nights” when the moon was above the horizon and in its third quarter.
2.4. Statistical analyses
All juvenile analyses excluded the first 15 days of a track following departure from the colony; as during this period, individuals are known to drift on the water while they wait for favorable wind conditions, upon which adopt a specific directional flight in order to rapidly move away from their natal ground and reach lower latitudes (De Grissac, Börger, Guitteaud, & Weimerskirch, 2016 ; Weimerskirch, Akesson, & Pinaud, 2006 ). Moreover, only the first eight months of data received from an individual was used, even if transmission were received beyond this. First, we used linear mixed models, Cochran–Mantel–Haenszel tests, and chi‐squared tests to ascertain how behavioral mode proportions of adults and juveniles varied with sex, through time and in comparison with each other. Then, to investigate how environmental conditions influence the probability for juveniles and adults to use the different behavioral modes, we performed a set of multinomial logistic regressions using the R package “mlogit” (Croissant, 2013 ). These models predict the probability that an individual engages in each of the four behaviors (see descriptions above) according to the corresponding environmental conditions of each location (Awkerman, Fukuda, Higuchi, & Anderson, 2005 ; Freeman et al., 2013 ). We also included day/night duration in our analyses, which was calculated using the “geosphere” R package (Hijmans, 2015 ). However, it is noted that this parameter is correlated with time, as birds were tracked from summer (December/January) to winter (July). Predictions are made in turn for each environmental variable at each time sample, while all other variables are considered fixed at average values. The model then tests how these predicted probabilities vary with respect to each other when environmental variables change (e.g., how the probability of one behavior changes compared to the probability of another, according to the value of environmental variable). Four models were fitted using four subsets of data: (1) diurnal locations of juveniles, (2) diurnal locations of adults, (3) nocturnal locations of juveniles, and (4) nocturnal locations of adults. This was because the behaviors of individuals differed substantially between the day and night (see Results section). Model selection was performed using Akaike's Information Criterion (AIC), by giving preference to the model exhibiting the lowest AIC. To avoid over‐parametrization, when the change in AIC was <2, we examined the number of parameters comprising a model and favored models with a smaller number of variables. Environmental variables were interpreted as having a significant influence on behavioral mode probabilities at p < .05. Autocorrelation in model residuals was tested for and found minimal at lag of 1–3 (see Fig. S2 ). To our knowledge, there is currently no way to account for autocorrelation in multinomial regression. For juvenile models, we got rid of most of the autocorrelation (at least to a lag 1) by resampling the input data to remove one of two consecutive locations. We did not resample at a courser resolution as this would have resulted in sample sizes too small for robust statistics, which is also the reason adult data were not resampled. Nevertheless, selected juvenile models with and without this correction produced very similar results. This suggests that while autocorrelation may be a source of some bias in our analyses, models are likely robust enough to give reliable results.
All values are given as average ±1 SD , unless stated. All analyses were computed using the R Software Environment (R Core Team 2015 ).
From the juvenile dataset, one track was discarded because just after leaving the colony the individual landed on the sea and drifted slowly for one month before the device stopped transmitting. This suggests the bird died before starting any foraging movements. A second track was discarded because device malfunction resulted in multiple oversized gaps between locations (up to three weeks in length). As such, the following results correspond to analyses conducted on the eight remaining tracks (five males and three females). Transmissions were received for between 112 and 372 days (mean = 246.5 ± 88.6 days), yielding an average of 10.8 ± 0.8 GPS locations per day and a dataset comprising 18,457 locations in total. Two individuals were tracked for <4 month (one male and one female) while the other six birds were tracked for a total of eight months or more (up until late December 2014). Across the three years (2013 through to 2015), adults were tracked between January and March for time periods lasting 8–25 days, resulting in a dataset comprising 4,385 locations in total.
3.1. Overall movements
During their first year at sea, juveniles dispersed widely across the subtropical Indian Ocean (40–25°S), ranging from the South African to Australian coasts and the Tasman Sea. Two individuals entered the Pacific Ocean and one the Atlantic Ocean (Figure 1 ). Until up to their eighth month at sea, all juveniles remained in relatively warm waters north of the sub‐Antarctic convergence (Figure 1 ). When in the western part of the Indian Ocean, they remained north of the subtropical front. Individuals flew mainly over oceanic waters but also visited shelf slopes without ever crossing the continental limit (200 m deep; see Fig. S2 ). There was individual variability in terms of the types of habitats visited, the times at which they were exploited and generalized movement patterns. While some individuals moved from oceanic waters to concentrate around shelf slopes, others remained continuously in oceanic habitats, and tended to use oceanographic features such as ridges and sea mounts and make larger scale movements (see Fig. S3 ). At a maximum, juveniles reached areas as far as 3,560 ± 1,277 km from the colony during their first month at sea, and after eight months, their maximum range averaged 7,674 ± 2,860 km (max 11,000 km). The six individuals that were tracked over eight months each covered an average total distance of 83,449 ± 7,837 km since their departure (min 74,328 km to max 96,120 km). The daily distances covered by all individuals increased significantly (Kruskal–Wallis: χ 7 2 = 23.414, p = .0014, Tukey post hoc: p = .011) between the first and second months from 237 ± 66 to 353 ± 41 km/day, but showed no significant trend afterward (Kruskal–Wallis: χ 6 2 = 9.794, p = .13). This compares to an adult average daily travel distance of 503 ± 134 km. As illustrated in Figure 2 , across all tracks, movements were characterized by continuous changes in the four behavioral modes determined by EMbC.

Trajectories of the eight juvenile (a) and 17 breeding adult (b) wandering albatrosses. Males are marked in blue and females in red. Crozet Island is symbolized by the yellow star. Dashed lines are, from South to North, the sub‐Antarctic front and the southern subtropical front

Fifty‐day portions of a juvenile's trajectory with behavioral modes as segmented by EM bC. The bar at the top of the figure shows an ethogram of behavioral segmentations (yellow: resting; red: active sitting; blue: ballistic movement; light blue: diffusive movement), while the central map shows an example juvenile track colored (as described above) by behavioral mode)
3.2. Sex differences
Juvenile diurnal activity was influenced by sex during the first 3 month at sea following fledging (see Fig. S4 ), with males spending more time resting than females (linear mixed model: t 6 = 2.87, estimate = 9.61 ± 3.34, p = .028). However, after 3 months, these differences were no longer significant ( t 6 = −1.14, estimate = −4.31 ± 3.76, p = .29). Due to the small sample size, the effect of sex was not evaluated for behavioral and environmental analyses on both juvenile and adult datasets.
3.3. Influence of diurnal cycle on behavior
The proportion of time spent in each of the four behavioral modes differed between day and night for both juveniles (Cochran–Mantel–Haenszel test controlling for individual: M 3 2 = 2,758.9, p < .001, Fig. S1 ) and adults (Cochran–Mantel–Haenszel test controlling for individual: M 3 2 = 190.9, p < .001). During the day, juveniles and adults predominantly performed “rapid” flying behaviors (ballistic: 42.1 ± 5.0% (juveniles), 43.2 ± 11.8% (adults), and diffusive: 33.2% ± 3.7 (juveniles), 31.4 ± 8.3% (adults); see the top of Fig. S1 ). In contrast, during the night juveniles mainly rested (43.7 ± 7.5%), while adults performed both ballistic (35.1 ± 10.1%) and resting (28.2 ± 7.6%) behaviors (see the bottom of Fig. S1 ).
3.4. Changes with time
Juvenile behavioral mode proportions varied with time since departure from the colony, following the same trend for both day and night analyses (Figure 3 ). Resting proportions decreased, while the use of diffusive movements steadily increased and ballistic behavioral proportions increased and then stabilized. The proportional use of active‐sitting behaviors remained relatively stable throughout the tracking period and was generally lower than that of the other behavioral modes. During the first month, compared to adults, juveniles spent more time resting and less time in flight (chi‐squared test: χ 3 2 = 84.352, p < .001). After this first month, during daylight, juvenile behavioral proportions fell within the range of those observed for adults (chi‐squared test: χ 3 2 = 1.3607, p = .714, Figure 3 a). For nighttime behaviors, differences between adults and juveniles were generally larger than those observed during the day, and were maintained up until the eighth month (chi‐squared test over the whole period: χ 3 2 = 196.12, p < .001), although a decrease through time was noted. Overall, across an entire trip (inclusive of both day and night movements), juveniles used resting and active‐sitting modes more often than adults, and ballistic behaviors less often (Figure 3 b).

Change over time of the proportion of behavioral mode use by juveniles during day (a) and night (b) alongside comparison with those of adults. Proportions of behavioral mode use (yellow: resting; red: active sitting; blue: ballistic movement; light blue: diffusive movement) are averaged by than across individuals and over periods of 1–2 months for juveniles (dots), and over the whole trip for adults (triangles). Bars show standard deviations. The gray line is the mean day duration in hours along juvenile tracks (from January to August), and the gray triangle is the mean duration of the day during the period across which adult tracking took place (summer)
3.5. Multinomial logistic regression
All variables retained following model selection had a significant influence on variation in the use probability of at least one behavior with respect to another. These are listed in Table 2 , alongside an estimation of their overall contribution (absolute value of the t ‐statistic). Full model outputs with parameter estimates and significance are displayed in Table 2 of Fig. S4 shows variation in behavioral use probabilities according to each individual variable tested, while keeping all other variables fixed at the mean value observed across the dataset.
Variables retained after model selection for the four multinomial logistic regression models alongside their overall contribution (absolute value of the t ‐statistic)
The most important variables are highlighted in bold.
3.5.1. Correlations between behavioral modes and night durations/time
Night duration significantly influenced the behavioral mode probabilities of juveniles during the night (Figure 4 b, top row). With increasing night length, the probabilities of resting and active‐sitting behaviors decreased while there was an increase in the probabilities of using ballistic (coefficient = .2, p < .001) and diffusive (coefficient = .27, p < .001; Figure 4 b, top row) behaviors. However, because night duration and time are highly correlated, it was not possible to determine whether these observations were mainly due to a seasonal decrease in night duration or also because juveniles gained experience through time. Night length varied little during the tracking period of adults. It had no effect on adult behavioral mode probabilities and was not retained following model selection procedures.

Modeled relationship between environmental variables and the probability of each behavioral mode use from multinomial logistic regression. Columns show day (a) and night (b) for juveniles and adults. Plain lines show predicted probabilities for juveniles and adults to perform each of the four behaviors (yellow: resting; red: active sitting; blue: ballistic movement; light blue: diffusive movement) in response to changes in night length, wind speed, bathymetry, chlorophyll‐a, and sea‐level height anomalies. Dashed lines represent 95% CI s
3.5.2. Environmental correlates of diurnal behavior
The influences of key environmental variables on the behavioral mode probabilities of juveniles and adults during the day are shown in Figure 4 (see detailed model outputs in Table S2 ). Wind speed was the most influential of these for adults and juveniles (Table 2 ). For both groups, with increasing wind speed, individuals were significantly more likely to perform ballistic and diffusive movements in place of active‐sitting or resting behaviors (all coefficients > .25, p < .05 for adults and juveniles, see Tables S2 and S3 ). Individual responses to changes in bathymetry were also similar across both the juvenile and adult groups. Here, with decreasing depth, the probability of performing ballistic movements progressively decreased in favor of more diffusive movements (coefficient = −.42, p < .05 [adults]; coefficient = −.29, p < .05 [juveniles], see Tables S2 and S3 ), which became more likely at depths shallower than 3,000 m (juveniles) and 2,000 m (adults). For juveniles, in locations with higher CHLa concentrations, flying (i.e., ballistic and diffusive) behavioral mode probabilities increased compared to resting modes (coefficient = .34 [ballistic] and 0.3 [diffusive], both p < .05, see (Tables S2 and S3 ). However, changes in CHLa concentrations did not influence adult behavioral mode probabilities. SLHAs only influenced adult behavioral mode probabilities. Here, individuals were more likely to engage in flying behaviors compared to resting behaviors when SLHAs were negative (coefficient = .24 [ballistic] and .23 [diffusive], both p < .05, see Tables S2 and S3 ).
3.5.3. Environmental correlates of nocturnal behavior
At night, juveniles performed mainly resting or active‐sitting behaviors. However, they became more likely to fly (i.e., through the performance of ballistic or diffusive movements) as wind speed increased (all p < .05, see Tables S2 and S3 ). Specifically, the probability of flying became higher than that of sitting at the surface when wind speeds exceeded 13 m/s (Figure 4 ). The same pattern was observed for adults, but with a much lower wind speed threshold of 5 m/s. Bathymetry had a significant effect on juveniles, driving an increase in the probability of using active‐sitting or diffusive behavior instead of ballistic movements at shallower depths (coefficient = .27 [active sitting] and .35 [diffusive], both p < .05, Tables S2 and S3 ). CHLa concentration and SLHA did not influence the nocturnal behaviors of juveniles (Figure 4 , Table 2 ), while SLHA did influence the behaviors of adults during the night. Here, although individuals were more likely to perform ballistic and diffusive movements when over negative SLHA, individual variability was high (Figure 4 ), and this was only significant when comparing ballistic movement probabilities to resting probabilities (coefficient = −.34, p = .05).
Moon brightness strongly influenced the nocturnal behavioral mode probabilities of both juveniles and adults (Table 2 ). When moonlight intensity increased, individuals were more likely to perform both ballistic and diffusive movements over resting (Table S2 and Fig. S5 ). We also observed a slight decrease in the probability of active‐sitting behaviors when moonlight was very bright (i.e., during full‐moon nights). For the juveniles tracked across both summer and winter, the effect of moonlight on behavioral mode probabilities is combined with the effect of night duration. During winter, when the nights are the longest (>13.5 hr), the proportion of time spent resting at night during a full moon was similar to that during the day (Figure 5 ). Conversely, when nights were short (<10.5 hr), juveniles spent most of the night resting regardless of moon brightness.

Proportion of resting behavior used by juveniles during full moon, half‐moon and dark nights according to night length. Long nights correspond to durations >13.5 hr, medium nights to durations of between 10.5 and 13.5 hr and short nights to durations <10.5 hr (classes obtained from observations of night length distribution)
4. DISCUSSION
Following fledging, juvenile wandering albatrosses spend 3–4 years at sea before returning to their natal colony. In this study, we show that within the first year, individuals develop and employ movement strategies similar to those observed in adults. At 2 months, juveniles were already found throughout the subtropical Indian Ocean and had daily travel distances exceeding 300 km, which is close to the average traveling rate of adults (Riotte‐Lambert & Weimerskirch, 2013 ; Salamolard & Weimerskirch, 1993 ). At the beginning of their at sea period, these performances may be due to innate capacities that would help respond to changes in self‐internal state and programmed necessities (Akesson & Weimerskirch, 2005 ; Alerstam et al., 2003 ). However, the marine environment in which these individuals forage is highly dynamic, and changes in biophysical oceanographic conditions drive the patchy distribution of prey alongside heterogeneity in movement costs for predators (Constable, Nicol, & Strutton, 2003 ; Hunt et al., 1999 ; Pennycuick, 1982 ; Weimerskirch, Guionnet, et al., 2000 ). As such, to optimize energy acquisition, juveniles need to be able to detect and interpret such structuring, and adapt their search strategies accordingly (Bartumeus & Catalan, 2009 ; Charnov, 1976 ). Such abilities are generally thought to be acquired and/or improved upon through time with experience (Newton, 2008 ). We show that, during the first months of their life at sea, inexperienced juveniles are able to respond to changes in their biophysical environment as adults do.
4.1. Individual variability
Juvenile wandering albatrosses displayed high individual variability in habitat use and large‐scale movement patterns. This is similar to that observed in migratory adults, which has been linked to age, colony, and sex (Weimerskirch et al., 2015 ). Partially inherited factors, such as personality (Patrick & Weimerskirch, 2015 ; Verbeek et al., 1994 ) and/or individual quality (i.e., consistent between‐individual differences related to phenotypic characteristics (Wilson & Nussey, 2010 ), may also influence individual variability in juvenile exploratory movements (Dingemanse, Both, Drent, van Oers, & van Noordwijk, 2002 ; Dingemanse, Both, Noordwijk, Rutten, & Drent, 2003 ). Indeed, individuals may differ in how they collect information about their surroundings and react to new environments (Verbeek et al., 1994 ). This may also be contingent upon early‐life conditions which can further influence individual quality and behavioral choice (Fay, Barbraud, Delord, & Weimerskirch, 2016 ; Fay, Weimerskirch, Delord, & Barbraud, 2015 ; Lindström, 1999 ; Stamps, 1987 ). However, while individual variability is common across a number of albatross species (Louzao et al., 2014 ; Shaffer, Costa, & Weimerskirch, 2001 ), observed levels in this study are likely emphasized due to the relatively small sample size (eight individuals), and so our results should be treated with some degree of caution.
4.2. Behavioral changes over time
Despite high individual variability, several behavioral patterns were identified that were consistent across individuals. During their first month at sea, juveniles flew < adults, spending more time on the surface, particularly at night. However, by the second month, daytime juvenile behavioral mode proportions were similar to those of adults. This may be because the flight capacities of juveniles, as indicated by daily travel distances here alongside patterns in speed and wind use elsewhere (Riotte‐Lambert & Weimerskirch, 2013 ; Weimerskirch et al., 2006 ), are already almost equal to those of adults. As such, the first month appears to be a period of rapid change for young birds when basic but vital capacities are likely developed through muscle reinforcement and optimal flight practice. This would enable individuals to quickly learn how to effectively use winds to reduce energetic movement costs. Indeed, rapid improvements in flying performance have already been observed for other young seabirds (e.g., brown booby sula leucogaster (Yoda, Kohno, & Naito, 2004 ). As such, long periods spent resting on the water during the first month of the at sea period may reflect especially high levels of flight energy expenditure in birds which at this time are untrained in flying skills. Subsequent decreases in the total time spent foraging alongside the probable use of suboptimal search strategies (Daunt et al., 2007 ; Wunderle, 1991 ; Wunderle & Martinez, 1987 ), mean that during this first month juveniles may rely on energetic reserves accumulated on the nest (Weimerskirch, Barbraud, & Lys, 2000 ). After the possible depletion of these reserves within the first few weeks at sea, finding food may become a priority (Soutullo, Urios, Ferrer, & Peñarrubia, 2006 ) which may explain why this adjustment period is so short, and basic foraging skills are rapidly learnt.
With time, juveniles tended to behave more and more similarly to breeding adults, yet after six months at sea still spent comparatively more time resting at night. We suggest this reflects the comparatively higher energetic demands of breeding adults alongside central place constraints, which force consistently higher levels of foraging effort regardless of underlying environmental conditions (Mackley, Phillips, Silk, Wakefield, Afanasyev, Fox, et al., 2010 ; Mackley, Phillips, Silk, Wakefield, Afanasyev, & Furness 2010 ; Pinet, Jaeger, Cordier, Potin, & Le Corre, 2011 ; Salamolard & Weimerskirch, 1993 ). Indeed, nonbreeding adult wandering albatrosses spend up to 50% more time on the water at night during winter, than the breeding adults of this study do in summer (>60% vs 30%, respectively; Weimerskirch et al. ( 2015 )). Progressive changes in juvenile behavior from the first month until at least the sixth may not solely be a consequence of ontogenetic modification, and could also relate to external constraints such as increases in night duration during winter which may induce observed decreases in the proportion of time spent resting.
Adaptation to light availability by diurnal animals is well known across a range of taxa (including seabirds) which use vision to navigate and locate prey (Clarke, Chopko, & Mackessy, 1996 ; Dias, Granadeiro, & Catry, 2012 ; Fernandez‐Duque, 2003 ; Mackley, Phillips, Silk, Wakefield, Afanasyev, & Furness 2010 ; Regular, Hedd, & Montevecchi, 2011 ; Weimerskirch, Gault, et al., 2005 ). As such, even if many of the prey of albatrosses (e.g., fish and squid) approach the surface at night, the ability to see and capture them from the air in the dark is reduced (Weimerskirch, Gault, et al., 2005 ). Indeed, direct measures of prey capture events have shown that wandering albatrosses obtain most of their prey during the day. However, they can also feed successfully at night (Weimerskirch, Gault, et al., 2005 ; Weimerskirch et al., 2007 ), through the use of a “sit‐and‐wait” foraging strategy (which is likely included in our active‐sitting behavior), has been suggested as an efficient alternative to the forage‐in‐flight strategy, particularly when individuals are highly time constrained (e.g., breeding adults; Louzao et al. ( 2014 )). During such periods, it may be difficult for birds to satisfy energetic demands during daylight hours, and so compensatory night foraging may be required. Such behavior may also be required during winter, when day lengths are reduced. For juveniles, this effect may be exacerbated by suboptimal foraging and/or competitive skills, which can prevent individuals from finding enough food during daytime and so encourage additional night foraging. Indeed, immature (>1 year old) albatrosses have been shown to forage more than adults during the night (Weimerskirch, Gault, et al., 2005 ), which would also explain why juveniles in our study spent less time resting at night than wintering adults (40% vs the 60% reported by Weimerskirch et al. ( 2015 )). Increases in foraging time to compensate suboptimal foraging performance have been suggested for other juvenile seabirds such as shags phalacrocorax aristotelis (Daunt et al., 2007 ). However unlike albatrosses, shags are unable to forage at night and so juveniles experience lower survival rates in winter when day duration is decreased. Notably, juvenile albatrosses in our study appear to take advantage of periods of increased moon light to forage more at night and compensate for a reduction in day length, thus particularly during winter. Lunar phase is known to influence the behavior of many birds, including pelagic seabirds such as petrels and shearwaters (Pinet et al., 2011 ; Yamamoto et al., 2008 ). A slight decrease in active‐sitting behavior probabilities for nights with a full moon may reflect behavioral changes, possibly because diurnally vertically migrating prey may position themselves at greater depths when moon light is increased rendering this foraging strategy less effective (Conners, Hazen, Costa, & Shaffer, 2015 ; Weimerskirch et al., 1997 ).
4.3. Adjustment to oceanographic conditions
The impact of wind on the flight costs and velocities of adult wandering albatrosses is well documented (Pennycuick, 1982 ; Weimerskirch, Guionnet, et al., 2000 ). In this study, juveniles appear to also react strongly to wind conditions, adjusting their behaviors similarly to adults. This suggests juveniles possess innate abilities to exploit wind, although further experience may be required to increase efficiency. For example, Riotte‐Lambert and Weimerskirch ( 2013 ) have shown individuals progressively fly mostly with tail and side‐to‐tail winds during the first months. The correct use of wind conditions is key to the efficient management of energetic budgets.
Besides wind and ambient light, young albatrosses also responded to changes in oceanic environmental conditions. When over waters deeper than 3,000 m (i.e., the majority of south western Indian Ocean), individuals were more likely to perform larger scale movements by performing ballistic movements. In contrast, diffusive movements were favored when flying over waters shallower than 3,000 m. Such sinuous movements are typically related to area restricted search behaviors (Kareiva & Odell, 1987 ; Louzao et al., 2014 ), which generally occur in areas of higher and more predictable prey density (Weimerskirch et al., 2007 ). The increased use of these types of strategies by juveniles in shallower waters may reflect the increased presence of favorable foraging habitats such as shelf slopes, seamounts, and ridges, which can aggregate prey (Fauchald & Tveraa, 2003 ; Louzao et al., 2011 ; Paiva, Geraldes, Ramírez, Garthe, & Ramos, 2010 ), which would trigger more intense exploration and/or use of prey search behaviors. In addition, juveniles increased search activity in areas with higher CHLa, which directly links to primary productivity and possibly reflects the idea that this parameter can be used as a proxy of higher‐trophic level prey availability (Hyrenbach et al., 2006 ). Coupled with detailed observations of bird trajectories, these results reiterate the importance of oceanographic features such as ridges, seamounts, and shelf slopes, alongside cyclonic oceanic eddies (which are known to be associated with increases in primary productivity) to foraging seabirds (Hunt et al., 1999 p. 199; McGillicuddy et al., 2007 ; Kai et al., 2009 ; Tosh et al., 2015 ). Although we did not detect links between adult behavioral patterns and areas of higher CHLa concentration, they have been previously shown to forage extensively over productive shelf slopes and oceanic plateaus (Waugh & Weimerskirch, 2003 ; Weimerskirch, 2007 ). Moreover, individuals were more likely to perform flying behaviors where sea‐level anomalies are negative, conditions that are typical at the centre of cyclonic eddies. Overall, juveniles, during their first months at sea, seemed to respond in a similar way to adults to the environment they encountered. In addition, these observed behavioral modifications suggest that juveniles may be able to respond, innately or after a short period of learning, to environmental proximal signals of prey availability such as water color and dimethyl‐sulfide odor alongside the presence of bird aggregations as it has been shown for adults (Fauchald, 2009 ; Nevitt, 2000 ). Finally, it is worth noting that juveniles and breeding adults face different constraints (notably, central place constraint for breeding birds), and a lack of nonbreeding adult data limits the interpretations made here.
Although recently fledged juveniles are able to detect and use potentially favorable habitats, they concentrate mainly in oceanic waters, where resources are known to be less abundant (Hunt et al., 1999 ). It is only after around six to seven months that some individuals start to concentrate around well‐known productive areas such as the shelf‐edges off the southwestern coast of Australia (Great Australian Bight). This may be partially explained by intraspecific and/or interspecific competitive pressures, as juveniles, with lower foraging performances, are thought to be less competitive than more experienced and so may be excluded from the most favorable areas birds (van den Hout et al., 2014 ; Sol, Santos, Garcia, & Cuadrado, 1998 ; Wheelwright & Templeton, 2003 ). However, while juveniles were able to accurately follow shelf‐slope contours (occurring at depths between 2,000 and 200 m), they seem to strictly avoid flying over the actual continental shelf (above 200 m deep) just like adults (Nicholls et al., 2002 ; Weimerskirch, 2007 and see Fig. S2 ).
5. CONCLUSION
In conclusion, juvenile albatrosses were able to respond to the environmental conditions they encountered during their dispersive movements in a manner similar to that observed in adults. However, it appears they only attain the body conditions and foraging skills of adults after several years of immaturity (Weimerskirch, 1992 ). Subsequently, the extensive duration of the immaturity period cannot be explained by movement performances and behavioral decisions alone, and may be additionally related to the accumulation of foraging skills, such as optimal prey choice and capture, alongside competitive ability. Such a long time period may also be necessary to acquire the experience needed to be able to forage from a central place (such as the breeding colony) and memorize information about the environmental conditions surrounding breeding grounds (when they begin visiting colonies from around four to five years of age). Such knowledge would enable immatures to bear both the costs of reproduction and maintenance. While current data do not yet enable investigations toward all these aspects of ontogeny of foraging behavior, new developments in tracking technologies will allow future studies to address these unknowns.
CONFLICT OF INTEREST
None declared.
DATA ACCESSIBILITY
Supporting information, acknowledgments.
The study at Crozet was supported by IPEV (program No 109). The Ethics Committee of IPEV and Comité de l'Environnement Polaire approved the field procedures. We thank the field assistants, especially Timothée Poupard and Valentin Nivet‐Mazerolle; Remi Fay for his relevant comments; Joan Garriga for sharing the EMbC package and his precious help to handle it; and the Wildlife Computer team for technical support. The study is a contribution to the Program EARLYLIFE funded by a European Research Council Advanced Grant under the European Community's Seven Framework Program FP7/2007–2013 (Grant Agreement ERC‐2012‐ADG_20120314 to Henri Weimerskirch). Sophie de Grissac was funded by the ERC Grant.
de Grissac S, Bartumeus F, Cox SL, Weimerskirch H. Early‐life foraging: Behavioral responses of newly fledged albatrosses to environmental conditions . Ecol Evol . 2017; 7 :6766–6778. https://doi.org/10.1002/ece3.3210 [ PMC free article ] [ PubMed ] [ Google Scholar ]
- Akesson, S. , & Weimerskirch, H. (2005). Albatross long‐distance navigation: Comparing adults and juveniles . Journal of Navigation , 58 , 365–373. [ Google Scholar ]
- Alerstam, T. , Hedenström, A. , & Åkesson, S. (2003). Long‐distance migration: Evolution and determinants . Oikos , 103 , 247–260. [ Google Scholar ]
- Awkerman, J. , Fukuda, A. , Higuchi, H. , & Anderson, D. (2005). Foraging activity and submesoscale habitat use of waved albatrosses Phoebastria irrorata during chick‐brooding period . Marine Ecology Progress Series , 291 , 289–300. [ Google Scholar ]
- Baker, R. R. (1993). The function of post‐fledging exploration: A pilot study of three species of passerines ringed in Britain . Ornis Scandinavica , 24 , 71–79. [ Google Scholar ]
- Bartumeus, F. , & Catalan, J. (2009). Optimal search behavior and classic foraging theory . Journal of Physics A: Mathematical and Theoretical , 42 , 434002. [ Google Scholar ]
- Charnov, E. L. (1976). Optimal foraging, the marginal value theorem . Theoretical Population Biology , 9 , 129–136. [ PubMed ] [ Google Scholar ]
- Clarke, J. A. , Chopko, J. T. , & Mackessy, S. P. (1996). The effect of moonlight on activity patterns of adult and juvenile prairie rattlesnakes ( Crotalus viridis viridis ) . Journal of Herpetology , 30 , 192–197. [ Google Scholar ]
- Clobert, J. , Perrins, C. M. , McCleery, R. H. , & Gosler, A. G. (1988). Survival rate in the great tit Parus major in relation to sex, age, and immigration status . The Journal of Animal Ecology , 57 , 287–306. [ Google Scholar ]
- Conners, M. G. , Hazen, E. L. , Costa, D. P. , & Shaffer, S. A. (2015). Shadowed by scale: Subtle behavioral niche partitioning in two sympatric, tropical breeding albatross species . Movement Ecology , 3 , 28. [ PMC free article ] [ PubMed ] [ Google Scholar ]
- Constable, A. J. , Nicol, S. , & Strutton, P. G. (2003). Southern ocean productivity in relation to spatial and temporal variation in the physical environment . Journal of Geophysical Research: Oceans , 108 , 8079. [ Google Scholar ]
- Croissant, Y. (2013). Mlogit: Multinomial logit model. R package version 0.2‐4. Retrieved , 13. [ Google Scholar ]
- Daunt, F. , Afanasyev, V. , Adam, A. , Croxall, J. P. , & Wanless, S. (2007). From cradle to early grave: Juvenile mortality in European shags Phalacrocorax aristotelis results from inadequate development of foraging proficiency . Biology Letters , 3 , 371–374. [ PMC free article ] [ PubMed ] [ Google Scholar ]
- De Grissac, S. , Börger, L. , Guitteaud, A. , & Weimerskirch, H. (2016). Contrasting movement strategies among juvenile albatrosses and petrels . Scientific Reports , 6 , 26103. [ PMC free article ] [ PubMed ] [ Google Scholar ]
- Dias, M. P. , Granadeiro, J. P. , & Catry, P. (2012). Do seabirds differ from other migrants in their travel arrangements? On route strategies of cory's shearwater during its trans–equatorial journey . PLoS One , 7 , e49376. [ PMC free article ] [ PubMed ] [ Google Scholar ]
- Dingemanse, N. J. , Both, C. , Drent, P. J. , van Oers, K. , & van Noordwijk, A. J. (2002). Repeatability and heritability of exploratory behaviour in great tits from the wild . Animal Behaviour , 64 , 929–938. [ Google Scholar ]
- Dingemanse, N. J. , Both, C. , van Noordwijk, A. J. , Rutten, A. L. , & Drent, P. J. (2003). Natal dispersal and personalities in great tits ( Parus major ) . Proceedings of the Royal Society of London. Series B: Biological Sciences , 270 , 741–747. [ PMC free article ] [ PubMed ] [ Google Scholar ]
- Fagan, W. F. , Cantrell, R. S. , Cosner, C. , Mueller, T. , & Noble, A. E. (2012). Leadership, social learning, and the maintenance (or collapse) of migratory populations . Theoretical Ecology , 5 , 253–264. [ Google Scholar ]
- Fauchald, P. (2009). Spatial interaction between seabirds and prey: Review and synthesis . Marine Ecology Progress Series , 391 , 139–151. [ Google Scholar ]
- Fauchald, P. , & Tveraa, T. (2003). Using first‐passage time in the analysis of area‐restricted search and habitat selection . Ecology , 84 , 282–288. [ Google Scholar ]
- Fay, R. , Barbraud, C. , Delord, K. , & Weimerskirch, H. (2016). Paternal but not maternal age influences early‐life performance of offspring in a long‐lived seabird . Proceedings of the Royal Society B: Biological Sciences , 283 , 20152318. [ PMC free article ] [ PubMed ] [ Google Scholar ]
- Fay, R. , Weimerskirch, H. , Delord, K. , & Barbraud, C. (2015). Population density and climate shape early‐life survival and recruitment in a long‐lived pelagic seabird . Journal of Animal Ecology , 84 , 1423–1433. [ PubMed ] [ Google Scholar ]
- Felicísimo, Á. M. , Muñoz, J. , & González‐Solis, J. (2008). Ocean surface winds drive dynamics of transoceanic aerial movements . PLoS One , 3 , e2928. [ PMC free article ] [ PubMed ] [ Google Scholar ]
- Fernandez‐Duque, E. (2003). Influences of moonlight, ambient temperature, and food availability on the diurnal and nocturnal activity of owl monkeys (Aotus azarai ) . Behavioral Ecology and Sociobiology , 54 , 431–440. [ Google Scholar ]
- Freeman, R. , Dean, B. , Kirk, H. , Leonard, K. , Phillips, R. A. , Perrins, C. M. , & Guilford, T. (2013). Predictive ethoinformatics reveals the complex migratory behaviour of a pelagic seabird, the Manx Shearwater . Journal of The Royal Society Interface , 10 , 20130279. [ PMC free article ] [ PubMed ] [ Google Scholar ]
- Friedland, K. D. , Stock, C. , Drinkwater, K. F. , Link, J. S. , Leaf, R. T. , Shank, B. V. , … Fogarty, M. J. (2012). Pathways between primary production and fisheries yields of large marine ecosystems . PLoS One , 7 , e28945. [ PMC free article ] [ PubMed ] [ Google Scholar ]
- Garriga, J. , Palmer, J. R. B. , Oltra, A. , & Bartumeus, F. (2016). Expectation–maximization binary clustering for behavioural annotation . PLoS One , 11 , e0151984. [ PMC free article ] [ PubMed ] [ Google Scholar ]
- Hazen, E. , Maxwell, S. , Bailey, H. , Bograd, S. , Hamann, M. , Gaspar, P. , … Shillinger, G. (2012). Ontogeny in marine tagging and tracking science: Technologies and data gaps . Marine Ecology Progress Series , 457 , 221–240. [ Google Scholar ]
- Hijmans, R. J. (2015). Geosphere: Spherical trigonometry. R package version 1.5‐1 . [ Google Scholar ]
- Horswill, C. , Matthiopoulos, J. , Green, J. A. , Meredith, M. P. , Forcada, J. , Peat, H. , … Ratcliffe, N. (2014). Survival in macaroni penguins and the relative importance of different drivers: Individual traits, predation pressure and environmental variability . Journal of Animal Ecology , 83 , 1057–1067. [ PMC free article ] [ PubMed ] [ Google Scholar ]
- van den Hout, P. J. , van Gils, J. A. , Robin, F. , van der Geest, M. , Dekinga, A. , & Piersma, T. (2014). Interference from adults forces young red knots to forage for longer and in dangerous places . Animal Behaviour , 88 , 137–146. [ Google Scholar ]
- Hunt, G. L. (1991). Occurrence of polar seabirds at sea in relation to prey concentrations and oceanographic factors . Polar Research , 10 , 553–560. [ Google Scholar ]
- Hunt, G. L. , Mehlum, F. , Russell, R. W. , Irons, D. , Decker, M. B. , & Becker, P. H. (1999). Physical processes, prey abundance, and the foraging ecology of seabirds Proceedings of the International Ornithological Congress (pp. 2040–2056).
- Hyrenbach, K. , Veit, R. , Weimerskirch, H. , & Hunt, L. Jr (2006). Seabird associations with mesoscale eddies: The subtropical Indian Ocean . Marine Ecology Progress Series , 324 , 271–279. [ Google Scholar ]
- Kai, E. T. , Rossi, V. , Sudre, J. , Weimerskirch, H. , Lopez, C. , Hernandez‐Garcia, E. , … Garçon, V. (2009). Top marine predators track Lagrangian coherent structures . Proceedings of the National Academy of Sciences of the United States of America , 106 , 8245–8250. [ PMC free article ] [ PubMed ] [ Google Scholar ]
- Kappes, M. (2009). Comparative foraging ecology and energetics of albatrosses . Santa Cruz: University of California. [ Google Scholar ]
- Kareiva, P. , & Odell, G. (1987). Swarms of predators exhibit “preytaxis” if individual predators use area–restricted search . American Naturalist , 130 , 233–270. [ Google Scholar ]
- Krebs, J. R. (1982). Territorial defence in the great tit ( Parus major ): Do residents always win? Behavioral Ecology and Sociobiology , 11 , 185–194. [ Google Scholar ]
- Lazaridis, E. (2014). Lunar: Lunar phase & distance, seasons and other environmental factors. R package version 0.1‐04 . [ Google Scholar ]
- Lewison, R. , Oro, D. , Godley, B. J. , Underhill, L. , Bearhop, S. , Wilson, R. P. , … Yorio, P. (2012). Research priorities for seabirds: Improving conservation and management in the 21st century . Endangered Species Research , 17 , 93–121. [ Google Scholar ]
- Lindström, J. (1999). Early development and fitness in birds and mammals . Trends in Ecology & Evolution , 14 , 343–348. [ PubMed ] [ Google Scholar ]
- Louzao, M. , Pinaud, D. , Peron, C. , Delord, K. , Wiegand, T. , & Weimerskirch, H. (2011). Conserving pelagic habitats: Seascape modelling of an oceanic top predator . Journal of Applied Ecology , 48 , 121–132. [ Google Scholar ]
- Louzao, M. , Weigand, T. , Bartumeus, F. , & Weimerskirch, H. (2014). Coupling instantaneous energy–budget models and behavioural mode analysis to estimate optimal foraging strategy: An example with wandering albatrosses . Movement Ecology , 2 , 8. [ PMC free article ] [ PubMed ] [ Google Scholar ]
- Mackley, E. K. , Phillips, R. A. , Silk, J. R. , Wakefield, E. D. , Afanasyev, V. , Fox, J. W. , & Furness, R. W. (2010). Free as a bird? Activity patterns of albatrosses during the nonbreeding period . Marine Ecology Progress Series , 406 , 291–303. [ Google Scholar ]
- Mackley, E. K. , Phillips, R. A. , Silk, J. R. D. , Wakefield, E. D. , Afanasyev, V. , & Furness, R. W. (2010). At‐sea activity patterns of breeding and nonbreeding white‐chinned petrels Procellaria aequinoctialis from South Georgia . Marine Biology , 158 , 429–438. [ Google Scholar ]
- Magrath, R. D. (1991). Nestling weight and juvenile survival in the blackbird, Turdus merula . The Journal of Animal Ecology , 60 , 335–351. [ Google Scholar ]
- Marchetti, K. , & Price, T. (1989). Differences in the foraging of juvenile and adult birds: The importance of developmental constraints . Biological Reviews , 64 , 51–70. [ Google Scholar ]
- McGillicuddy, D. J. , Anderson, L. A. , Bates, N. R. , Bibby, T. , Buesseler, K. O. , Carlson, C. A. , … Steinberg, D. K. (2007). Eddy/wind interactions stimulate extraordinary mid‐ocean plankton blooms . Science , 316 , 1021–1026. [ PubMed ] [ Google Scholar ]
- Mueller, T. , O'Hara, R. B. , Converse, S. J. , Urbanek, R. P. , & Fagan, W. F. (2013). Social learning of migratory performance . Science , 341 , 999–1002. [ PubMed ] [ Google Scholar ]
- Naef‐Daenzer, B. , Widmer, F. , & Nuber, M. (2001). Differential post‐fledging survival of great and coal tits in relation to their condition and fledging date . Journal of Animal Ecology , 70 , 730–738. [ Google Scholar ]
- Nevitt, G. A. (2000). Olfactory foraging by Antarctic procellariiform seabirds: Life at high Reynolds numbers . The Biological Bulletin , 198 , 245–253. [ PubMed ] [ Google Scholar ]
- Nevoux, M. , Weimerskirch, H. , & Barbraud, C. (2007). Environmental variation and experience‐related differences in the demography of the long‐lived black‐browed albatross . Journal of Animal Ecology , 76 , 159–167. [ PubMed ] [ Google Scholar ]
- Newton, I. (2008). The migration ecology of birds . Academic Press, Oxford, UK. [ Google Scholar ]
- Nicholls, D. , Robertson, C. , Prince, P. , Murray, M. , Walker, K. , & Elliott, G. (2002). Foraging niches of three Diomedea albatrosses . Marine Ecology Progress Series , 231 , 269–277. [ Google Scholar ]
- Paiva, V. H. , Geraldes, P. , Ramírez, I. , Garthe, S. , & Ramos, J. A. (2010). How area restricted search of a pelagic seabird changes while performing a dual foraging strategy . Oikos , 119 , 1423–1434. [ Google Scholar ]
- Patrick, S. C. , & Weimerskirch, H. (2015). Senescence rates and late adulthood reproductive success are strongly influenced by personality in a long‐lived seabird . Proceedings of the Royal Society B: Biological Sciences , 282 , 20141649. [ PMC free article ] [ PubMed ] [ Google Scholar ]
- Pennycuick, C. J. (1982). The flight of petrels and albatrosses (Procellariiformes), observed in South Georgia and its vicinity . Philosophical Transactions of the Royal Society of London B, Biological Sciences , 300 , 75–106. [ Google Scholar ]
- Perrin, M. R. (1979). The roles of reproduction, survival, and territoriality in the seasonal dynamics of Clethrionomys gapperi populations . Acta Theriologica , 24 , 475–500. [ Google Scholar ]
- Phillips, R. A. , Xavier, J. C. , Croxall, J. P. , & Burger, A. E. (2003). Effects of satellite transmitters on albatrosses and petrels . The Auk , 120 , 1082–1090. [ Google Scholar ]
- Pinaud, D. , & Weimerskirch, H. (2007). At‐sea distribution and scale‐dependent foraging behaviour of petrels and albatrosses: A comparative study . Journal of Animal Ecology , 76 , 9–19. [ PubMed ] [ Google Scholar ]
- Pinet, P. , Jaeger, A. , Cordier, E. , Potin, G. , & Le Corre, M. (2011). Celestial moderation of tropical seabird behavior . PLoS One , 6 , e27663. [ PMC free article ] [ PubMed ] [ Google Scholar ]
- R Core Team . (2015). R: A language and environment for statistical computing . Vienna, Austria: R Foundation for Statistical Computing. [ Google Scholar ]
- Regular, P. M. , Hedd, A. , & Montevecchi, W. A. (2011). Fishing in the dark: A pursuit‐diving seabird modifies foraging behaviour in response to nocturnal light levels . PLoS One , 6 , e26763. [ PMC free article ] [ PubMed ] [ Google Scholar ]
- Riotte‐Lambert, L. , & Weimerskirch, H. (2013). Do naive juvenile seabirds forage differently from adults? Proceedings of the Royal Society B: Biological Sciences , 280 , 20131434. [ PMC free article ] [ PubMed ] [ Google Scholar ]
- Salamolard, M. , & Weimerskirch, H. (1993). Relationship between foraging effort and energy requirement throughout the breeding season in the wandering albatross . Functional Ecology , 7 , 643. [ Google Scholar ]
- Sandell, M. , & Smith, H. G. (1991). Dominance, prior occupancy, and winter residency in the great tit (parus major) . Behavioral Ecology and Sociobiology , 29 , 147–152. [ Google Scholar ]
- Scales, K. L. , Miller, P. I. , Hawkes, L. A. , Ingram, S. N. , Sims, D. W. , & Votier, S. C. (2014). Review: On the Front Line: Frontal zones as priority at‐sea conservation areas for mobile marine vertebrates (ed A Punt) . Journal of Applied Ecology , 51 , 1575–1583. [ Google Scholar ]
- Scales, K. L. , Miller, P. I. , Ingram, S. N. , Hazen, E. L. , Bograd, S. J. , & Phillips, R. A. (2016). Identifying predictable foraging habitats for a wide‐ranging marine predator using ensemble ecological niche models . Diversity and Distributions , 22 , 212–224. [ Google Scholar ]
- Sergio, F. , Schmitz, O. J. , Krebs, C. J. , Holt, R. D. , Heithaus, M. R. , Wirsing, A. J. , … Korpimäki, E. (2014). Towards a cohesive, holistic view of top predation: A definition, synthesis and perspective . Oikos , 123 , 1234–1243. [ Google Scholar ]
- Shaffer, S. A. , Costa, D. P. , & Weimerskirch, H. (2001). Behavioural factors affecting foraging effort of breeding wandering albatrosses . Journal of Animal Ecology , 70 , 864–874. [ Google Scholar ]
- Sol, D. , Santos, D. M. , Garcia, J. , & Cuadrado, M. (1998). Competition for food in urban pigeons: The cost of being juvenile . The Condor , 100 , 298–304. [ Google Scholar ]
- Soutullo, A. , Urios, V. , Ferrer, M. , & Peñarrubia, S. G. (2006). Post‐fledging behaviour in Golden Eagles Aquila chrysaetos : Onset of juvenile dispersal and progressive distancing from the nest . Ibis , 148 , 307–312. [ Google Scholar ]
- Stamps, J. A. (1987). The effect of familiarity with a neighborhood on territory acquisition . Behavioral Ecology and Sociobiology , 21 , 273–277. [ Google Scholar ]
- Thorup, K. , Alerstam, T. , Hake, M. , & Kjellén, N. (2003). Bird orientation: Compensation for wind drift in migrating raptors is age dependent . Proceedings of the Royal Society of London. Series B: Biological Sciences , 270 , S8–S11. [ PMC free article ] [ PubMed ] [ Google Scholar ]
- Tosh, C. A. , de Bruyn, P. J. N. , Steyn, J. , Bornemann, H. , van den Hoff, J. , Stewart, B. S. , … Bester, M. N. (2015). The importance of seasonal sea surface height anomalies for foraging juvenile southern elephant seals . Marine Biology , 162 , 2131–2140. [ Google Scholar ]
- Verbeek, M. E. M. , Drent, P. J. , & Wiepkema, P. R. (1994). Consistent individual differences in early exploratory behaviour of male great tits . Animal Behaviour , 48 , 1113–1121. [ Google Scholar ]
- Waugh, S. M. , & Weimerskirch, H. (2003). Environmental heterogeneity and the evolution of foraging behaviour in long ranging greater albatrosses . Oikos , 103 , 374–384. [ Google Scholar ]
- Weimerskirch, H. (1992). Reproductive effort in long‐lived birds: Age‐specific patterns of condition, reproduction and survival in the wandering albatross . Oikos , 64 , 464. [ Google Scholar ]
- Weimerskirch, H. (2007). Are seabirds foraging for unpredictable resources? Deep Sea Research Part II: Topical Studies in Oceanography , 54 , 211–223. [ Google Scholar ]
- Weimerskirch, H. , Akesson, S. , & Pinaud, D. (2006). Postnatal dispersal of wandering albatrosses Diomedea exulans : Implications for the conservation of the species . Journal of Avian Biology , 37 , 23–28. [ Google Scholar ]
- Weimerskirch, H. , Barbraud, C. , & Lys, P. (2000). Sex differences in parental investment and chick growth in wandering albatrosses: Fitness consequences . Ecology , 81 , 309–318. [ Google Scholar ]
- Weimerskirch, H. , Bonadonna, F. , Bailleul, F. , Mabille, G. , Dell'Omo, G. , & Lipp, H.‐P. (2002). GPS tracking of foraging albatrosses . Science , 295 , 1259. [ PubMed ] [ Google Scholar ]
- Weimerskirch, H. , Cherel, Y. , Cuenot‐Chaillet, F. , & Ridoux, V. (1997). Alternative foraging strategies and resource allocation by male and female wandering albatrosses . Ecology , 78 , 2051–2063. [ Google Scholar ]
- Weimerskirch, H. , Delord, K. , Guitteaud, A. , Phillips, R. A. , & Pinet, P. (2015). Extreme variation in migration strategies between and within wandering albatross populations during their sabbatical year, and their fitness consequences . Scientific Reports , 5 , 8853. [ PMC free article ] [ PubMed ] [ Google Scholar ]
- Weimerskirch, H. , Gault, A. , & Cherel, Y. (2005). Prey distribution and patchiness: Factors in foraging success and efficiency of wandering albatrosses . Ecology , 86 , 2611–2622. [ Google Scholar ]
- Weimerskirch, H. , Guionnet, T. , Martin, J. , Shaffer, S. A. , & Costa, D. P. (2000). Fast and fuel efficient? Optimal use of wind by flying albatrosses . Proceedings of the Royal Society of London. Series B: Biological Sciences , 267 , 1869–1874. [ PMC free article ] [ PubMed ] [ Google Scholar ]
- Weimerskirch, H. , Lallemand, J. , & Martin, J. (2005). Population sex ratio variation in a monogamous long‐lived bird, the wandering albatross . Journal of Animal Ecology , 74 , 285–291. [ Google Scholar ]
- Weimerskirch, H. , Pinaud, D. , Pawlowski, F. , & Bost, C.‐A. (2007). Does prey capture induce area‐restricted search? A fine‐scale study using gps in a marine predator, the wandering albatross . The American Naturalist , 170 , 734–743. [ PubMed ] [ Google Scholar ]
- Wheelwright, N. T. , & Templeton, J. J. (2003). Development of foraging skills and the transition to independence in juvenile savannah sparrows . The Condor , 105 , 279–287. [ Google Scholar ]
- Wilson, A. J. , & Nussey, D. H. (2010). What is individual quality? An evolutionary perspective . Trends in Ecology & Evolution , 25 , 207–214. [ PubMed ] [ Google Scholar ]
- Wunderle, J. M. (1991). Age‐specific foraging proficiency in birds . Current Ornithology , 8 , 273–324. [ Google Scholar ]
- Wunderle, J. M. Jr , & Martinez, J. S. (1987). Spatial learning in the nectarivorous bananaquit: Juveniles versus adults . Animal Behaviour , 35 , 652–658. [ Google Scholar ]
- Yamamoto, T. , Takahashi, A. , Yoda, K. , Katsumata, N. , Watanabe, S. , Sato, K. , & Trathan, P. N. (2008). The lunar cycle affects at‐sea behaviour in a pelagic seabird, the streaked shearwater, Calonectris leucomelas . Animal Behaviour , 76 , 1647–1652. [ Google Scholar ]
- Yoda, K. , Kohno, H. , & Naito, Y. (2004). Development of flight performance in the brown booby . Proceedings of the Royal Society of London B: Biological Sciences , 271 , S240–S242. [ PMC free article ] [ PubMed ] [ Google Scholar ]
The Wandering Albatross and Global Warming
The giant oceanic birds are producing more and plumper chicks, at least for now
/https://tf-cmsv2-smithsonianmag-media.s3.amazonaws.com/accounts/headshot/greg-laden-240.jpg)
Weather changes not just from season to season, but also from year to year. Where I live in Minnesota, we had only a few days of frost before the year’s end, and January, normally the coldest month of the year, was relatively balmy. But in another year we might have days on end of sub-zero weather during the winter. It is hard for a person to detect climate change at this scale, even though global temperature measurements clearly show that the planet has warmed.
But every now and then something comes along that demonstrates a longer term trend that we can see and measure more directly. For instance, the USDA recently released a new version of its “ Plant Hardiness Zone Map .” If you are a gardener in the United States, you probably already know about this map; its zones are used to determine what kinds of plants can be grown outdoors in your area, the estimated dates of the last killing frost in the spring and the first killing frost in the fall. This is at least the second time in my memory that this map has been redrawn with all the zones moved to the north, reflecting a warming planet in a way that every gardener can observe and understand.
Not all global climate changes are simple warming, however. Global warming causes changes in ocean and atmospheric circulation as well. Westerly winds in the southern Pacific Ocean have shifted south towards the pole and have become more intense. A recent study in Science shows that the foraging patterns of breeding Wandering Albatross ( Diomedea exulans ) on the Crozet Islands has been changed by global warming in a way that seems to benefit them now, but that will likely harm them in the future.
Albatross are members of the bird order Procellariiformes, also known as the “tubenoses” because of the tube-like “nostrils” on their beaks. There are about 170 species of this kind of bird, including the petrels, shearwaters, storm petrels, diving petrels, and albatrosses. It is commonly said that the ocean is the last great frontier on earth, and this is probably true. It should not come as a surprise, then, that the Procellariiformes are among the “last great frontiers” of birding and bird research. Since the tubenoses spend almost all of their time at sea, they are hard to study. They come to land only to breed, and even then, usually on remote islands. They are so committed to being in the air over the ocean or floating on the surface of the sea that most members of this order are unable to walk at all. One group of tubenoses has the capacity to shoot a stream of noxious liquid (from its gut) at potential predators, which is an interesting adaptation to being unable to stand up and peck at intruders attempting to eat one’s egg or chick. (See this post for more information on tubenoses and a review of an excellent recent book on the tubenoses of North America.)
For all these reasons, foraging during nesting is a stress point in the life history of albatross. The birds forage by soaring around over the ocean, using wind as their main form of propulsion, literally sniffing out food sources (they have excellent smelling abilities). Therefore, the pattern of oceanic winds should matter a lot to their survival, especially during breeding season.
Which brings us back to changes in wind patterns due to global warming. The study by Henri Weimerskirch, Maite Louzao, Sophie de Grissac and Karine Delord is destined to become a classic because it touches on a sequence of logically connected observations to tell a compelling story. For my part, I’m going to use this in a classroom to demonstrate interesting science at my next opportunity. Let’s go over it step by step.
Albatross breeding is clearly difficult, and failure is likely common. One indicator of this is the fact that wandering albatross lay only one egg per season. Most coastal and terrestrial birds lay more than one, and in many species the number they lay varies from year to year depending on conditions. If wandering albatross lay only one egg, ever, there is a sort of underlying biological expectation of a low success rate.
For most birds, size matters. Within the normal range for a species, individual birds grow larger when conditions are good, and those birds do better in periods of difficulty because a large body stores more reserves and provides for more effective competition with other birds. A bird can grow large and bring lots of food back to the nest only if foraging is good, and the amount of food a bird obtains in a day is a combination of time (how long one forages) and the amount of food available in the environment.
The amount of food an albatross can obtain depends in part on the total area of the ocean that is searched each day, which in turn depends on how fast the bird flies. Since the albatross soars on the wind most of the time, this means that everything depends on factors such as the speed and direction of the wind. The study we are looking at today combines all of these things in an elegant exposé of the link between climate and the difficult job of producing baby albatrosses.
The wandering albatross travel enormous distances from their breeding grounds, often going more than 1,000 miles before returning to the nest to relieve their mate from guard duty. Males forage more widely and more to the south than females, who prefer northern waters. During this time, the birds use the wind as their primary form of locomotion. The researchers have shown that the winds in this region have increased in strength by a measurable amount, owing to shifts related to global warming. The average wind speed has gone up by about 10 percent from the 1990s to the present day. This allows the birds to move from foraging area to foraging area more swiftly than otherwise possible.
The total amount of time it takes both male and female albatross to complete a full journey of a given distance has decreased by between 20 percent and 40 percent from the 1990s to the present, and the speed at which the birds are observed to fly has gone up about the same for females, though the observed speed increase for males is not statistically significant. This is direct evidence that the amount of time spent foraging is less under present conditions than it was in the recent past, and it can be inferred that this is caused by the correlated increases in wind speed.
During the same period of time, the birds have gotten bigger. In 1990 the average female was about 7,500 grams and by 2010 females were about 8,500 grams. Males increased by about the same percentage, going from the mid-9,000 range to about 10,500 grams. These differences in mass are not reflected in the overall dimensions of the bird, just their weight. This indicates that during periods when the birds are on average smaller, many are underfed.
Breeding success for albatross varies considerably. The chance of successfully launching a baby albatross from the nest for the 350 pairs studied ranges from about 50 percent to just over 80 percent depending on the year (I’m leaving out one really bad year when the success rate was only 25 percent). During the past 40 years, over which it is thought the wind patterns have changed as described above, the “moving average” of breeding success (taking a few years together into account to dampen natural variation) has changed from about 65 percent to about 75 percent. These birds indeed seem to be benefiting from changes in wind pattern caused by global warming.
Most changes in weather, patterns of wind and rain and other effects of global warming are negative, as any review of the literature on this topic over the past decade will show. The benefits being experienced by these birds is unusual. But it may also be temporary. The researchers who produced this result say that the shift of winds towards the poles that brought higher energy patterns to these islands is likely to continue. As wind speeds increase, the benefit the birds will receive will at first level off then start to decrease, as overly windy conditions are bad for the albatross. The shift of westerly winds to the south of the islands will probably decrease the viability of foraging over the next few decades because it will make it easier for the birds to get to places with lower quality forage and thus decrease the rate of obtaining food. So, if the current changes in wind patterns are a gravy train for the Crozet Island wandering albatross, the train may eventually leave the station without them.
Weimerskirch, H., Louzao, M., de Grissac, S., & Delord, K. (2012). Changes in Wind Pattern Alter Albatross Distribution and Life-History Traits Science, 335 (6065), 211-214 DOI: 10.1126/science.1210270
Get the latest Science stories in your inbox.
/https://tf-cmsv2-smithsonianmag-media.s3.amazonaws.com/accounts/headshot/greg-laden-240.jpg)
Greg Laden | | READ MORE
Greg Laden is a freelance science writer.
- Bird Health
- Bird Behavior
- Birds Comparison
- Bird Watching
The Biography of Albatrosses and Their Oceanic Realm
- February 21, 2024 February 20, 2024
With their majestic wingspans and graceful flight, Albatrosses are among the most iconic seabirds inhabiting the world’s oceans.
Belonging to the family Diomedeidae, these remarkable birds are renowned for their ability to soar vast distances with minimal effort, utilizing dynamic soaring techniques to traverse the expansive ocean expanses where they spend most of their lives.
Albatrosses are predominantly found in the Southern Hemisphere, particularly in the open waters of the Antarctic, sub-Antarctic, and temperate regions. They exhibit a remarkable lifespan, with some species living for decades and even up to 60 years.
These avian wonders play a crucial role in marine ecosystems, serving as indicators of ocean health and contributing to nutrient cycling through their feeding habits.
However, albatross populations face numerous threats, including habitat degradation, pollution, and incidental bycatch, making their conservation important.
Physical Characteristics of Albatrosses
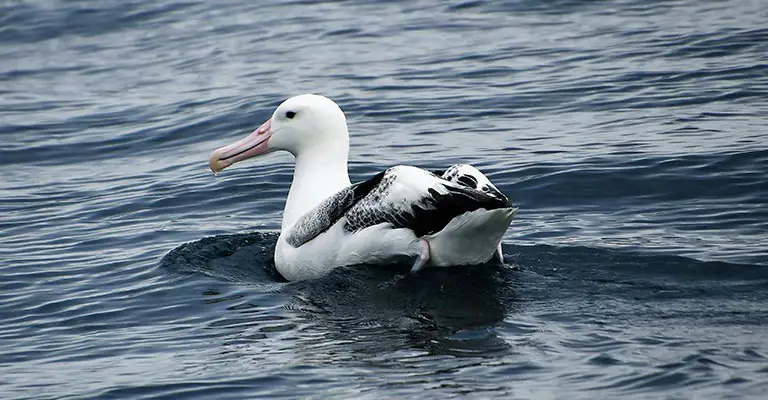
Identifying albatrosses can be captivating, as these majestic seabirds possess distinctive physical characteristics that set them apart from other avian species.
Here are eight key points to consider when identifying albatrosses:
Large Size and Wingspan
Albatrosses are notably large birds, with wingspans that can exceed 11 feet (3.4 meters) in some species, making them one of the biggest flying birds on Earth.
This impressive wingspan allows them to soar effortlessly over vast oceanic expanses for extended periods.
Long, Narrow Wings
Albatrosses have long, narrow wings perfectly adapted for gliding and dynamic soaring. These wings are significantly longer in proportion to their bodies compared to other seabirds, giving them a distinctive silhouette in flight.
White Plumage
Most albatross species have predominantly white plumage, contrasting sharply with the dark ocean waters below.
Some species may have varying degrees of black or gray markings on their wings or backs, but the overall impression is luminous whiteness.
Yellow Bill and Tubular Nostrils
One of the most noticeable features of albatrosses is their large, hooked bills, which are usually yellow.
Additionally, they have tubular nostrils located on either side of the bill, which are thought to aid in excreting excess salt ingested from seawater.
Webbed Feet
Albatrosses have webbed feet, adapted for swimming and aid in takeoff from the water’s surface. These feet are relatively small in proportion to their body size and are primarily used for steering while swimming.
Distinctive Flight Patterns
In flight, albatrosses exhibit a characteristic gliding motion, rarely flapping their wings except during takeoff and landing. They often soar low over the waves, effortlessly riding air currents with minimal effort.
Long, Streamlined Bodies
Albatrosses have sleek, streamlined bodies that minimize air resistance during flight. Their bodies are built for efficiency in the air, allowing them to cover vast distances with minimal energy expenditure.
Graceful Movements on Land
Despite their large size, albatrosses are surprisingly graceful on land, moving with a distinctive waddling gait. On solid ground, they may tuck their long wings close to their bodies, giving them a more compact appearance.
Identifying albatrosses involves observing a combination of physical characteristics, including their large size, yellow bill, webbed feet, distinctive flight patterns, streamlined bodies, and graceful movements both in the air and on land.
These unique features make albatrosses unmistakable and fascinating subjects for birdwatchers and naturalists alike.
Taxonomy of Albatrosses
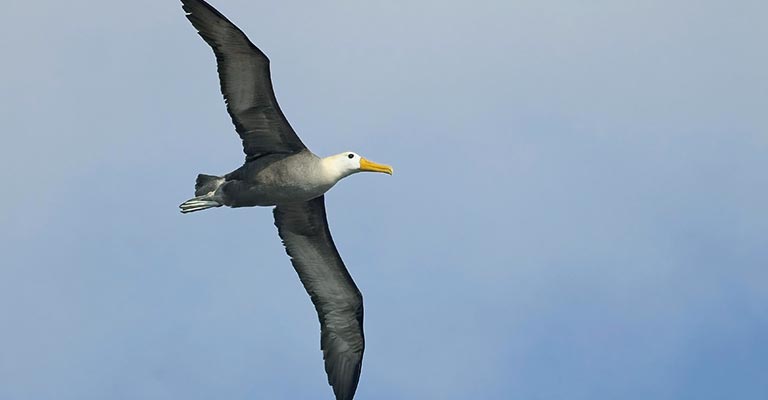
Here is a table that will showcase all the taxonomical details of Albatrosses:
The albatross family comprises several species with unique characteristics and distribution across the world’s oceans. Here’s a brief overview of the species mentioned:
Short-tailed Albatross (Phoebastria albatrus)
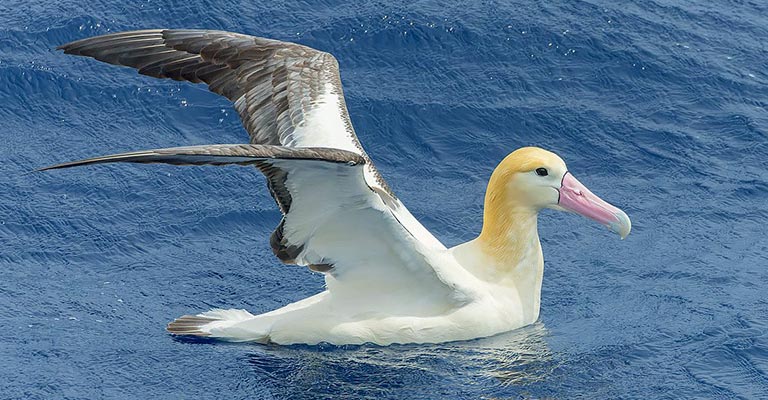
Recognizable by its large size, white body, and pale yellow bill, the short-tailed albatross is one of the rarest and most endangered species. It inhabits the North Pacific Ocean and breeds on Torishima and the Senkaku Islands.
Black-footed Albatross (Phoebastria nigripes)
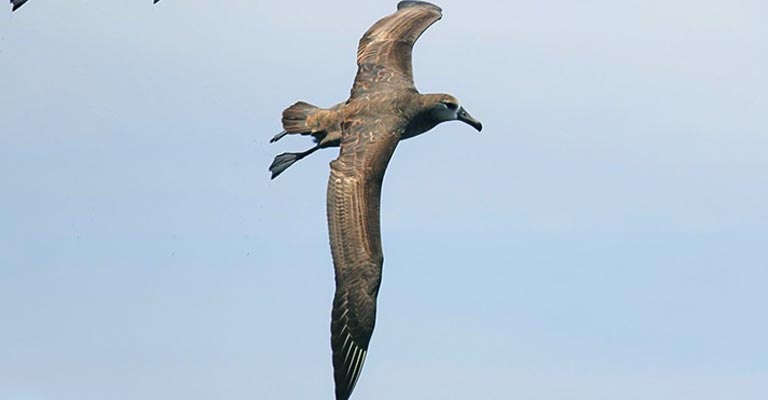
Named for its dark feet, this species has a black back and wings with a white face and underparts. It nests primarily in the Northwestern Hawaiian Islands and the Japanese archipelago.
Laysan Albatross (Phoebastria immutabilis)
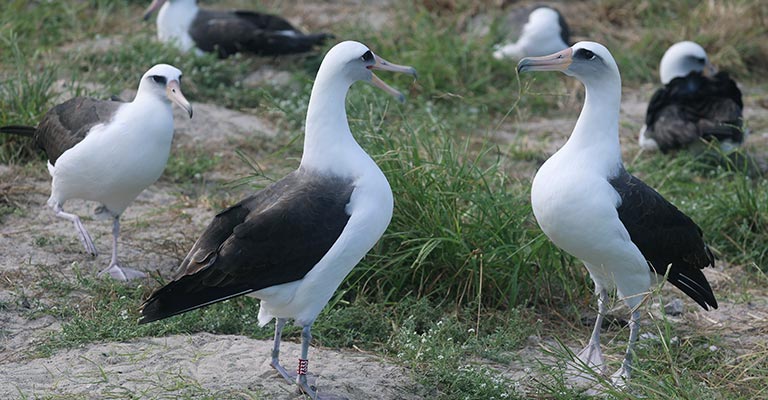
The Laysan albatross is a familiar sight in the North Pacific with a striking appearance characterized by a white body, black wings, and a yellow-orange bill. It breeds primarily on islands such as Laysan and Midway Atoll.
Snowy Albatross (Diomedea exulans)
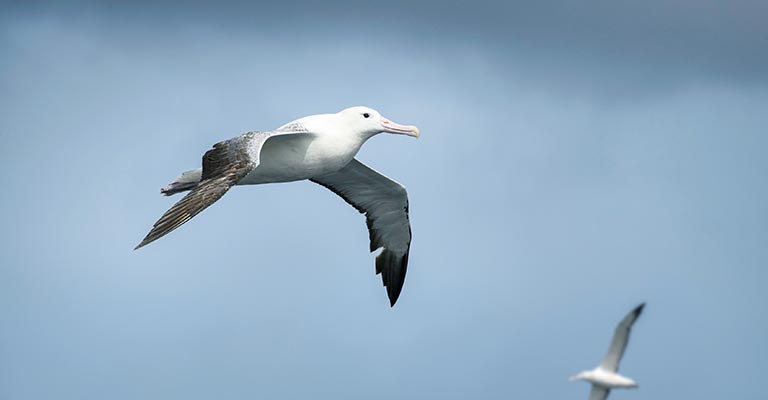
Also known as the wandering albatross, the snowy albatross is the largest of all albatross species. It has a white body with black wingtips and a pink bill with a distinctive hook. It ranges widely across the Southern Ocean.
Light-mantled Albatross (Phoebetria palpebrata)
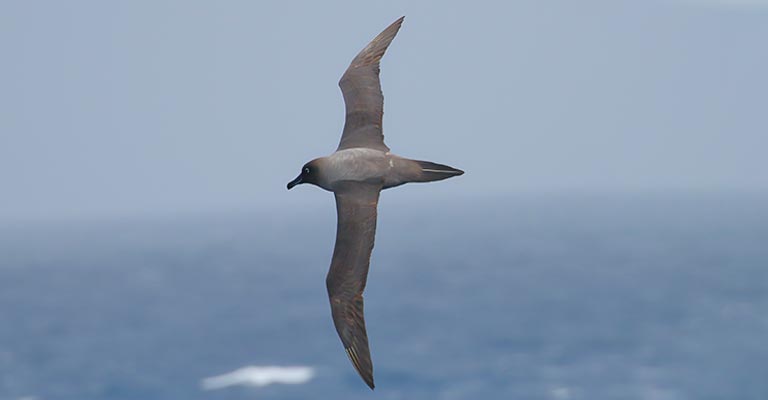
This species is named for the pale gray mantle on its upperparts. It has a white head, neck, underparts, black flight feathers, and a dark bill. It breeds on islands such as South Georgia and the Falkland Islands.
Black-browed Albatross (Thalassarche melanophris)
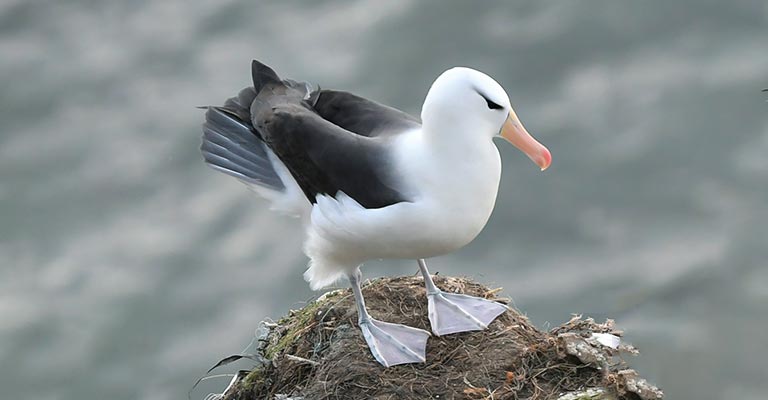
Recognizable by the dark eyebrow stripe above its eye, the black-browed albatross has a white body with dark upper wings and a yellow bill. It nests on islands in the Southern Ocean, including the Falkland Islands and South Georgia.
Salvin’s Albatross (Thalassarche salvini)
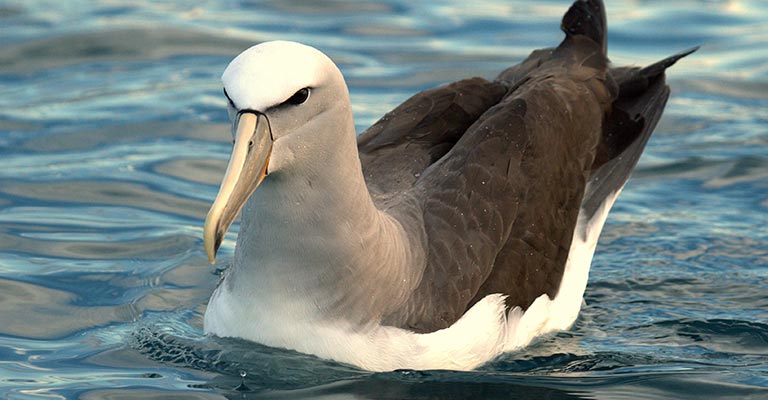
Named after the English ornithologist Osbert Salvin, this species has a dark mantle and upper wings, a white face, throat, underparts, and a yellow-orange bill. It breeds on islands such as the Antipodes Islands and the Bounty Islands.
Chatham Albatross (Thalassarche eremita)
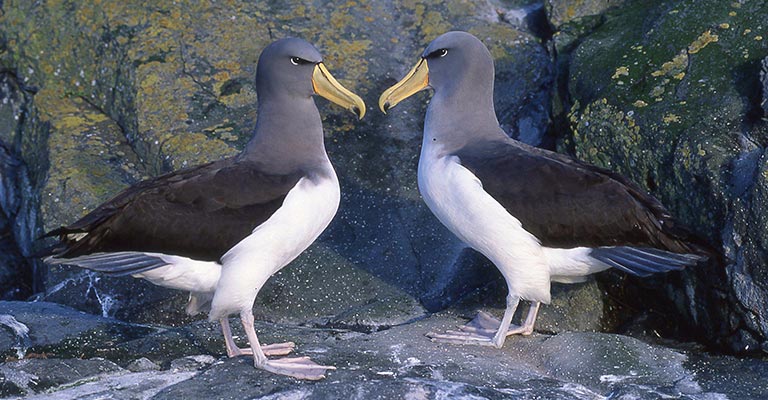
Endemic to the Chatham Islands of New Zealand, this species has a dark mantle and upper wings, a white face, throat, and underparts, and a yellow bill with a dark tip.
White-capped Albatross (Thalassarche steadi)
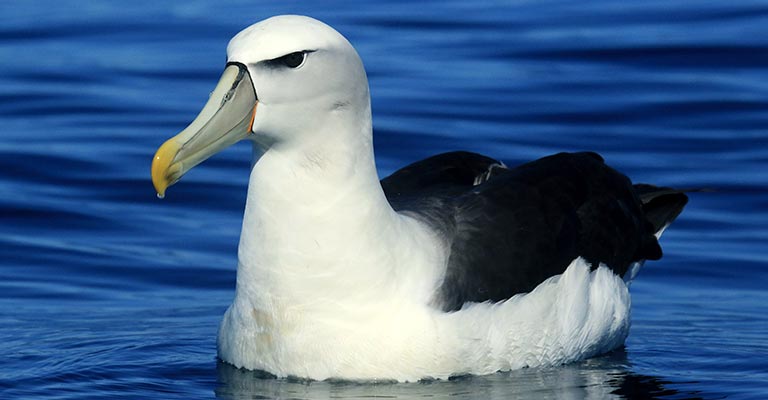
With a white cap on its head and a dark mantle, this species has a white face, throat, underparts, and a yellow-orange bill. It breeds on islands such as Campbell Island and the Auckland Islands.
Atlantic Yellow-nosed Albatross (Thalassarche chlororhynchos)
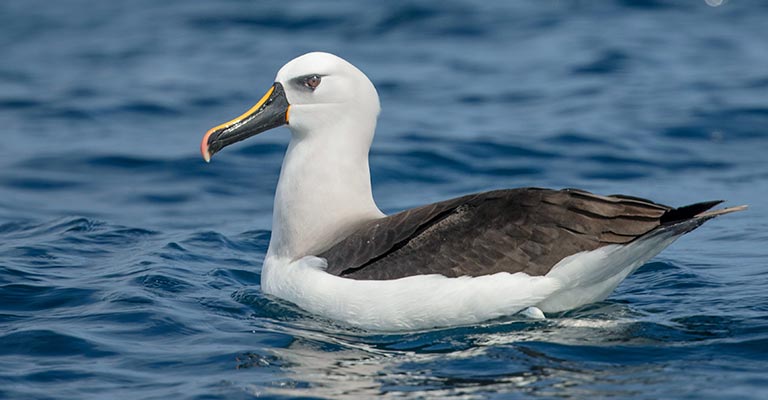
Found primarily in the South Atlantic Ocean, this species has a yellow bill with a black tip, a white face, throat, underparts, and dark upperparts. It breeds on islands such as Gough Island and Tristan da Cunha.
These albatross species play a unique role in marine ecosystems and face conservation challenges, including habitat loss, fisheries bycatch, and marine pollution.
Conservation efforts are crucial to ensuring the survival of these magnificent birds for generations to come.
Common Food of Albatrosses
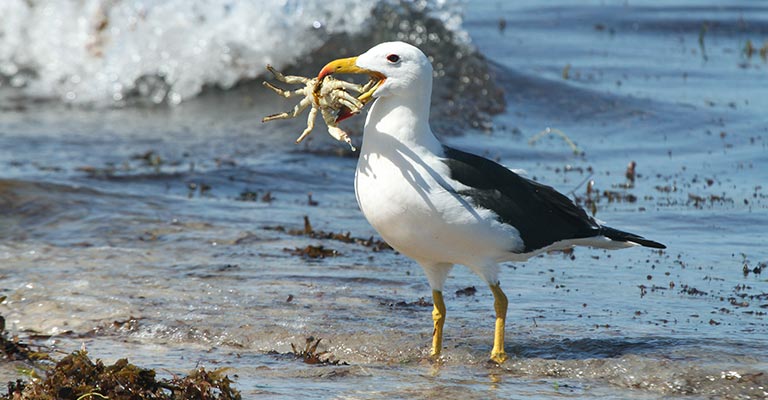
Albatrosses are primarily carnivorous seabirds with diverse diets that vary depending on their species and foraging habits. Familiar food sources for albatrosses include:
- Fish: Albatrosses feed on various fish species, including lanternfish, squid, and small pelagic fish like anchovies and sardines.
- Crustaceans: Some albatross species supplement their diet with crustaceans such as krill, shrimp, and crab larvae.
- Cephalopods: Squid and octopus are significant components of the albatross diet, providing valuable protein and nutrients.
- Offal and carrion: Albatrosses are opportunistic feeders and may scavenge offal from fishing vessels or carrion floating on the ocean surface.
- Flying fish: These agile birds are known to catch flying fish in mid-air as they leap from the water to escape predators.
Albatrosses are adept at foraging over vast oceanic expanses, using their keen eyesight and sense of smell to locate food sources efficiently.
Hunting Habit of Albatrosses
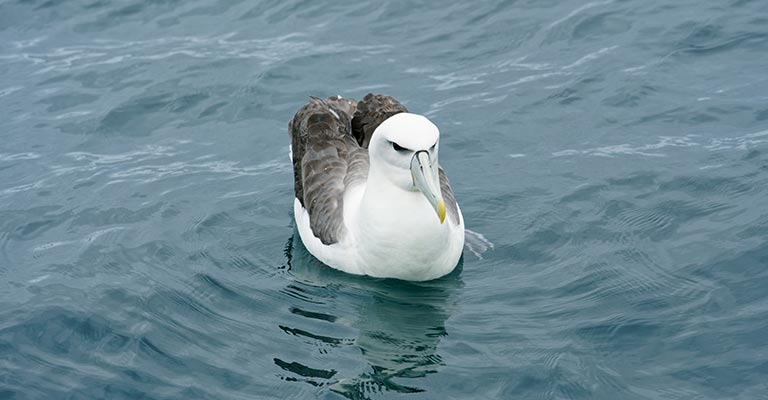
Albatrosses are highly skilled hunters, relying on their keen senses and efficient flight capabilities to locate and capture prey in the vast expanse of the open ocean.
With their exceptional eyesight, these birds can scan the water surface from great heights, spotting potential prey such as fish, squid, and crustaceans.
Once a target is identified, albatrosses use their impressive aerial agility to dive down and snatch prey from the water’s surface, often employing dramatic swoops and maneuvers to catch their elusive quarry.
Utilizing a combination of gliding and dynamic soaring techniques, albatrosses can cover large distances with minimal energy expenditure, allowing them to search for food over expansive oceanic regions for extended periods.
Their hunting behavior is opportunistic, taking advantage of both natural prey concentrations and the presence of fishing vessels to scavenge for offal and discarded fish.
Albatrosses Life History
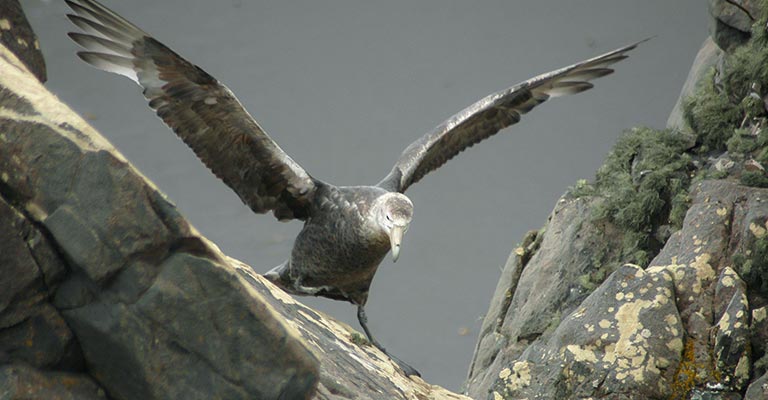
With their majestic wingspans and graceful flight, Albatrosses are iconic seabirds known for their remarkable life history and importance in marine ecosystems.
These fascinating birds exhibit a range of behaviors and adaptations that enable them to thrive in the harsh conditions of the open ocean.
From their foraging habits to nesting behaviors, albatrosses possess a unique life history that reflects their specialized role in the marine environment.
Albatrosses are primarily carnivorous, feeding on diverse prey, including fish, squid, crustaceans, and occasionally carrion. They use their keen eyesight to spot prey from the air and employ aerial agility to capture it from the water’s surface.
Albatrosses are pelagic birds, spending the majority of their lives at sea and only returning to land to breed and rear their young.
They inhabit many oceanic habitats, from the Southern Ocean around Antarctica to the North Pacific and North Atlantic Oceans.
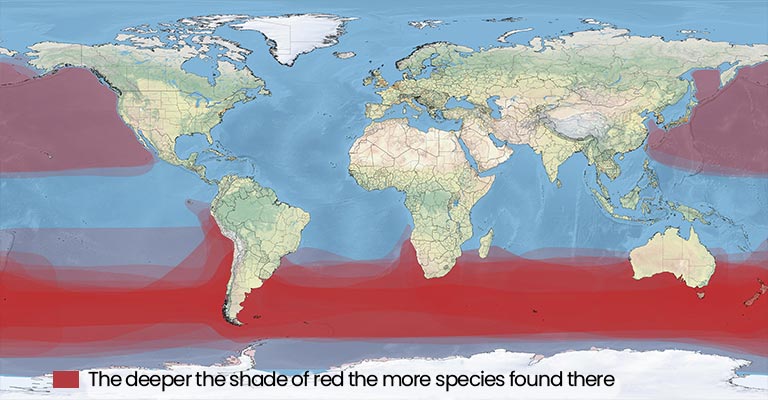
Albatross species have specific ranges dictated by their breeding and foraging behaviors. Range maps illustrate the distribution of various species across oceans and continents, highlighting key breeding colonies and foraging grounds.
Albatrosses typically nest in large colonies on remote islands, often on cliffs or rocky outcrops, to avoid terrestrial predators. They construct simple nests of vegetation or soil where they lay a single egg.
Breeding behavior in albatrosses involves elaborate courtship displays, with pairs forming long-term bonds. Incubation and chick-rearing duties are shared between parents, with chicks fledging after several months.
Albatross populations are susceptible to various diseases, including avian pox and aspergillosis. These diseases can have significant impacts on individual birds and breeding colonies.
Treatment of albatross diseases often involves veterinary care provided by conservation organizations and research institutions.
Interventions may include medication administration, wound treatment, and rehabilitation efforts for affected individuals.
Conservation
Albatrosses face numerous conservation challenges, including habitat loss, fisheries bycatch, pollution, and climate change.
Conservation efforts focus on mitigating these threats through habitat protection, bycatch reduction technologies, and international agreements to safeguard albatross populations and their marine habitats.
The life history of albatrosses encompasses a range of fascinating behaviors and adaptations that highlight their importance as apex predators of the open ocean.
Understanding and conserving these magnificent birds is essential for maintaining the health and integrity of marine ecosystems worldwide.
5 Fun Facts About Albatrosses
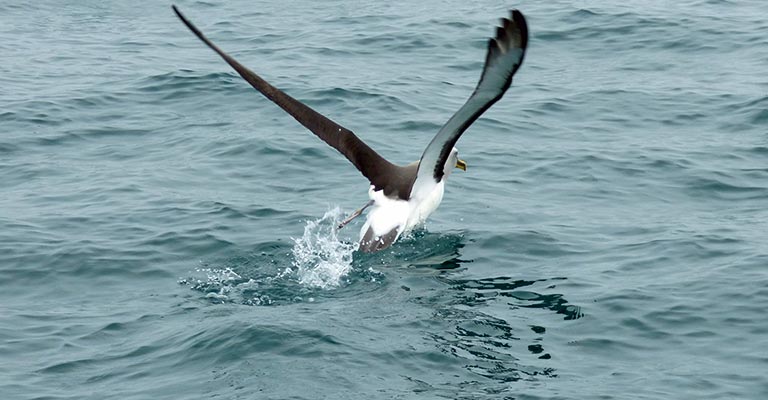
Albatrosses, with their impressive wingspans and remarkable abilities, are among the most fascinating seabirds in the world. Here are five fun facts about these magnificent birds:
- Dynamic Soaring Masters: Albatrosses are adept at dynamic soaring, a flying technique using wind gradients to gain energy and travel vast distances with minimal effort. They can cover thousands of miles without flapping their wings.
- Longest Wingspan in Birds: The wandering albatross holds the longest wingspan of any living bird, reaching up to 11 feet (3.4 meters). This remarkable wingspan allows them to glide effortlessly over the open ocean for extended periods.
- Monogamous Pairs: Albatrosses are known for their long-term monogamous relationships. Mated pairs often perform elaborate courtship rituals, including synchronized dances and bill-clacking displays, to reinforce their bonds.
- Incredible Lifespan: Some albatross species, such as the Laysan albatross, have astonishingly long lifespans, with individuals known to live for over 60 years. This longevity is crucial for successful breeding and maintaining populations over time.
- Marine Conservation Indicators: Albatross populations are essential indicators of marine ecosystem health. Their presence and breeding success reflect the abundance of prey species and the overall condition of oceanic habitats, making them invaluable for conservation efforts.
Wrapping Up
Albatrosses stand as magnificent ambassadors of the open seas, captivating us with their soaring flights, intricate courtship rituals, and vital role in marine ecosystems.
As apex predators of the ocean, they command our admiration and concern.
Yet, their future hangs precariously in the balance, threatened by habitat degradation, pollution, and climate change. We must rally together to protect these majestic birds and their oceanic habitats.
By implementing effective conservation measures, supporting research initiatives, and raising awareness about the plight of albatrosses, we can ensure that these remarkable seabirds continue to grace our skies and inspire generations to come.
Leave a Reply Cancel reply
Your email address will not be published. Required fields are marked *
Save my name, email, and website in this browser for the next time I comment.
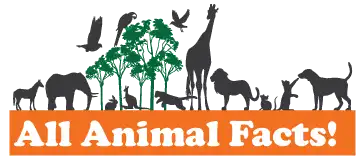
- May 16, 2022
Wandering Albatross
The wandering albatross is a large seabird that can be found throughout the world’s oceans. These magnificent creatures are known for their impressive wingspans, which can reach up to 11 feet wide. Wandering albatrosses are also recognized for their gentle nature and graceful movements. In this blog post, we will discuss some interesting facts about these amazing birds . We’ll also take a closer look at the habitat and behavior of the wandering albatross. So, if you’re interested in learning more about these fascinating creatures, keep reading!
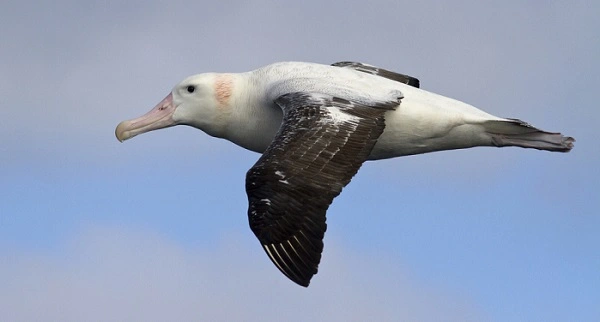
Wandering Albatross Description
Wandering Albatrosses are the largest of all the albatrosses . They are mostly white, with black Wingtips and a yellow bill . Wandering Albatrosses can be found in the Southern Ocean, where they spend most of their time flying in search of food. They are excellent flyers , and can stay in the air for hours at a time without flapping their wings. When they do land, it is usually on an iceberg or another floating object. Wandering Albatrosses mate for life , and pairs will often perform elaborate courtship rituals before nesting. These rituals can involve calling to each other, bowing, and preening each other’s feathers. Once a pair has bonded, they will return to the same nesting site year after year. Wandering Albatrosses typically lay two eggs at a time , but only one chick will survive to fledge. The chicks are born downy and white, and grow quickly on a diet of regurgitated food from their parents. Wandering Albatrosses are fascinating birds, and their long wingspans make them a sight to behold in flight.
Wandering Albatross Habitat
Wandering Albatross habitat Wandering albatrosses are the largest flying birds in the world. These magnificent birds are typically found in the Southern Ocean , where they breed on remote island groups such as the Falkland Islands , South Georgia , and the Crozet Archipelago . While Wandering albatrosses spend most of their time at sea, they do come ashore to nest. Their nest sites are typically located on slopes or ridges near the ocean, where they can take advantage of the wind to help them takeoff and land. Wandering albatrosses typically lay one egg per breeding season, which is incubated by both parents for around 80 days. Once the chick hatches, it will remain in the nest for up to nine months before taking its first flight. Wandering albatrosses can live for over 60 years, and they play an important role in the marine ecosystem by scavenging for carrion and helping to disperse nutrients around the ocean.
Wandering Albatross Diet
Wandering Albatrosses feed on squid , fish and krill . They have sharp beaks that they use to catch their prey. Wandering Albatrosses usually eat whatever is most abundant at the time. They sometimes follow ships to scavenge food scraps. Wandering Albatrosses mate for life and lay one egg per year. The chicks are born on remote islands and stay with their parents for up to two years before setting out to find a mate of their own.
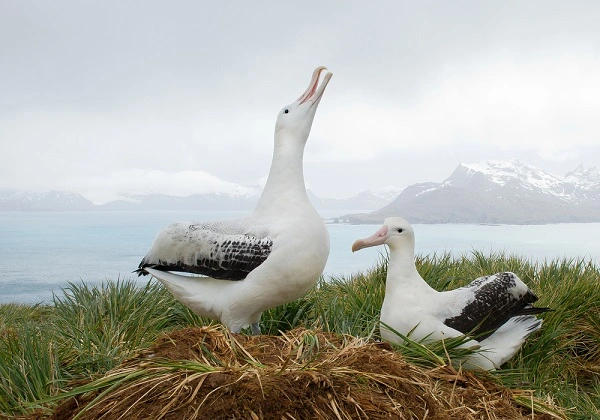
Wandering Albatross Size
Wandering Albatrosses are the largest flying birds in the world , with a wingspan that can reach up to 11 feet . Wandering Albatrosses are found in the southern oceans near Antarctica and on isolated islands in the Pacific and Indian Oceans. These birds spend most of their time at sea, only coming ashore to breed. Wandering Albatrosses are pelagic birds, meaning that they live and feed far out at sea. Their diet consists mainly of squid and fish, which they capture by swooping down on their prey from a great height. Wandering Albatrosses are truly remarkable birds, and their enormous size is just one of the many things that make them so special.
Wandering Albatross Lifespan
Wandering Albatrosses are one of the longest-lived bird species , with a lifespan of up to 50 years . However, their life expectancy is reduced in captivity , with birds in zoos and aviaries typically only living for 20-30 years . In the wild, Wandering Albatrosses face a number of threats, including habitat loss, pollution, and predation by introduced species. As a result of these threats, Wandering Albatross populations have declined in recent years. Despite these challenges, Wandering Albatrosses continue to captivate audiences with their impressive wingspan and their elegant flying style. These birds are truly amazing creatures, and it is hoped that their populations will recover in the future.
Wandering Albatross Behavior
Wandering albatrosses breed on remote islands in the Southern Ocean , and they spend most of their lives at sea, only coming ashore to breed. These birds are highly nomadic , and they often travel thousands of miles in search of food. Wandering albatrosses typically eat squid and fish, but they have also been known to scavenge carrion from ships. Their long wings allow them to glide for hours on wind currents, and they are adept at riding these currents to find food. Wandering albatrosses mate for life, and they typically lay two eggs per breeding season. The chicks are born blind and helpless, and it takes them several months to learn how to fly. Once they fledge, wandering albatrosses do not return to land until they are ready to breed themselves. These birds have an incredibly long lifespan, with some individuals living for over 50 years.
Wandering Albatross Speed
Wandering Albatrosses are one of the fastest, capable of flying at speeds of up to 95 kilometers per hour . Wandering Albatrosses use their speed to help them cover large distances in search of food. Their long wings also help them to glide for long periods of time without having to flap their wings, allowing them to conserve energy while they fly. The Wandering Albatross is the fastest member of the albatross family and one of the fastest birds in flight.
Wandering Albatross Hunting
One of the most fascinating – and concerning – things about Wandering Albatrosses is their hunting strategy . Essentially, they will follow boats and ships in order to scavenge food, which can often lead to them being accidentally caught in fishing lines or other equipment. In fact, Wandering Albatrosses are thought to be one of the most heavily affected bird species when it comes to fisheries bycatch. Hunting these magnificent birds is having a significant impact on global populations, and something needs to be done in order to protect them. For this reason, a number of organizations are working hard to raise awareness of the issue and find ways to reduce bycatch. Hopefully, by working together we can ensure that Wandering Albatrosses are around for future generations to enjoy.
Frequently Asked Question
What is a Wandering Albatross?
How big do wandering albatrosses get and what do they look like, what kind of habitat do wandering albatrosses live in and what do they eat, how long do wandering albatrosses live for and how many young do they have, do wandering albatrosses migrate.
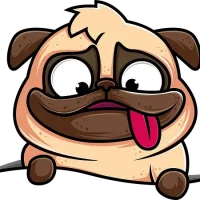
- Terms and Conditions
- Privacy Policy
- DMCA Notice
- Reference Manager
- Simple TEXT file
People also looked at
Original research article, foraging behavior and energetics of albatrosses in contrasting breeding environments.
- 1 Department of Ecology and Evolutionary Biology, University of California, Santa Cruz, Santa Cruz, CA, United States
- 2 Centre d'Études Biologiques de Chizé, Centre National de la Récherche Scientifique, Villiers en Bois, France
Animals can maximize fitness by optimizing energy acquisition through the selection of favorable foraging habitats, but trade-offs exist between time spent in preferred feeding habitats, energetic costs of travel, and reproductive constraints. For pelagic seabirds, geographic distribution of suitable breeding islands can restrict access to marine prey resources and influence foraging strategies. Laysan ( Phoebastria immutabilis ) and black-footed albatrosses ( P. nigripes ) breeding in the Northwest Hawaiian Islands, and Indian yellow-nosed albatrosses ( Thalassarche carteri ) breeding in the Southern Indian Ocean, utilize productive subtropical-subpolar transition zones during their breeding and non-breeding periods, but this marine feature is at a comparatively greater distance for Hawaiian albatrosses during the breeding period due to location of nesting islands. We investigated the foraging behavior and energetics of these three species to evaluate how proximity to preferred marine habitats has influenced their overall foraging strategies. During incubation, all three species traveled to subtropical-subpolar transition zones, however, Hawaiian albatrosses ranged farther to reach this habitat. All species reduced time at sea during brooding, and Hawaiian albatrosses reduced their foraging ranges to distances similar to yellow-nosed albatrosses. As a consequence, Hawaiian albatrosses foraged in the warm, oligotrophic environment of the subtropical gyre during brooding while yellow-nosed albatrosses continued to forage in a subtropical-subpolar transition zone. Landing rates, an indicator of foraging effort, did not differ between reproductive stages and were highly variable within and among species. Hawaiian albatrosses generally spent more time in flight compared to yellow-nosed albatrosses, a strategy that may relate to searching for dispersed and unpredictable prey. Mean absolute field-metabolic rate (FMR) was greatest for black-footed albatrosses, and similar between Laysan and yellow-nosed albatrosses, but mass-specific FMR did not differ between species. Hawaiian albatrosses had lower total body water than yellow-nosed albatrosses (indicating greater lipid reserves), and had FMRs that fell below the allometric relationship for studied albatross species, attributes that likely reflect physiological adaptations for foraging in a low-productivity environment.
Introduction
Animals can maximize fitness by optimizing energy acquisition through the selection of preferred habitats ( Emlen, 1966 ; MacArthur and Pianka, 1966 ; Levins, 1968 ; Pyke, 1984 ), however, trade-offs exist when preferred foraging habitats are distant to breeding habitats ( Charnov, 1976 ; Orians and Pearson, 1979 ; Alerstam and Högstedt, 1982 ; Weimerskirch and Cherel, 1998 ). In the marine environment, animals that breed on land but forage at sea should adopt a strategy that optimizes energy gain while minimizing the cost of transporting energy resources (e.g., food, oil, milk) back to the breeding site ( Ricklefs, 1983 ; Pennycuick et al., 1984 ; Costa, 1991 ; Houston, 1993 ; Costa and Shaffer, 2012 ). Because marine prey are patchily distributed within a fluid, dynamic environment ( MacKas and Boyd, 1979 ; Russell et al., 1992 ; Fauchald et al., 2000 ; Weimerskirch, 2007 ), marine predators can optimize energy acquisition by exploiting physical oceanographic features that aggregate prey resources ( Schneider, 1990 ; Hunt, 1997 ; Hunt et al., 1998 ; Croll et al., 2005 ; Keiper et al., 2005 ). According to central place foraging theory, whether or not an individual selects a prey patch depends on its distance to the central place as well as its quality ( Orians and Pearson, 1979 ; Olsson and Bolin, 2014 ). Therefore, proximity of the breeding site to productive marine habitat is likely to play a role in shaping foraging strategies of marine predators ( Costa, 1993 ; Harding et al., 2013 ).
For pelagic seabirds, access to preferred marine habitats during the breeding season depends on location of the breeding colony, reproductive stage, and energetic costs of travel ( Orians and Pearson, 1979 ; Weimerskirch et al., 1993 ; Guinet et al., 1997 ; Shaffer et al., 2003 ). Albatrosses are well-adapted to long-distance travel due to their economical mode of flight ( Pennycuick, 1982 ; Costa and Shaffer, 2008 ; Sibly et al., 2012 ) and anatomical specialization for soaring and gliding ( Pennycuick, 1982 ), which enable low flight costs ( Costa and Prince, 1987 ; Shaffer et al., 2004 ). Albatross foraging range is variably constrained during the breeding period, however, due to changing energetic requirements at the nest. During incubation, the fasting capabilities of adults allow breeding pairs to alternate long shifts at the nest (~2–3 weeks) with far-ranging trips to sea. Foraging range contracts toward the end of incubation, and becomes most restricted during the brooding period, when young chicks require frequent meals and adults alternate short trips to sea (~3 days) with time spent at the nest provisioning young chicks. The chick-rearing period begins when the fasting and thermoregulatory capabilities of chicks have developed sufficiently for them to remain at the nest independently, enabling both adults to take longer trips to sea (~2–3 weeks). Albatrosses are therefore able to search for prey resources in productive habitats on basin-wide scales during the incubation and chick-rearing periods ( Jouventin and Weimerskirch, 1990 ; BirdLife International, 2004 ; Kappes et al., 2015 ), but are limited to short-distance trips during brooding, when energy deficits can occur in order to maximize food delivery to the chick ( Ricklefs, 1983 ; Shaffer et al., 2003 ). When rearing larger chicks, albatrosses allocate resources between themselves and their offspring, and may employ a dual foraging strategy, whereby adults maximize prey delivery to chicks by making short-distance trips, and restore their body condition when making long-distance trips ( Weimerskirch et al., 1997 ).
Laysan ( Phoebastria immutabilis ) and black-footed albatrosses ( P. nigripes ) breeding in the Hawaiian Islands, and Indian yellow-nosed albatrosses ( Thalassarche carteri ) breeding on Amsterdam Island in the southern Indian Ocean, utilize similar marine habitats (in two different ocean basins) when making long-range movements, but differ in terms of accessibility of preferred foraging habitats during the breeding period. On long foraging trips during incubation and chick-rearing, all three species utilize subtropical-subpolar transition zones ( Hyrenbach et al., 2002 ; Pinaud and Weimerskirch, 2005 ; Pinaud et al., 2005 ; Kappes et al., 2015 ) where warm, subtropical waters come into contact with cooler, subpolar waters ( Backus, 1986 ; Olson, 2001 ). These are highly productive pelagic habitats ( Lutjeharms and Valentine, 1984 ; Barange et al., 1998 ; Read et al., 2000 ; Olson, 2001 ; Polovina et al., 2001 ) that provide enhanced foraging opportunities for surface-feeding predators like albatrosses because surface convergence along frontal boundaries can aggregate neustonic or buoyant prey ( Olson and Backus, 1985 ; Franks, 1992 ; Govoni and Grimes, 1992 ; Olson et al., 1994 ). During the brooding period, however, when albatrosses take shorter foraging trips, Laysan and black-footed albatrosses are restricted to foraging in warm, oligotrophic waters ( Fernández et al., 2001 ; Kappes et al., 2010 , 2015 ) where prey abundance may be lower ( Ashmole, 1971 ; Ballance et al., 1997 ). Conversely, yellow-nosed albatrosses have access to cooler, more productive waters similar to habitats utilized during incubation and chick-rearing (Figure 1 ; Pinaud and Weimerskirch, 2005 ; Pinaud et al., 2005 ). The latter case is more characteristic of albatrosses in general; most albatrosses breed on islands in productive pelagic or coastal upwelling environments ( Tickell, 2000 ).
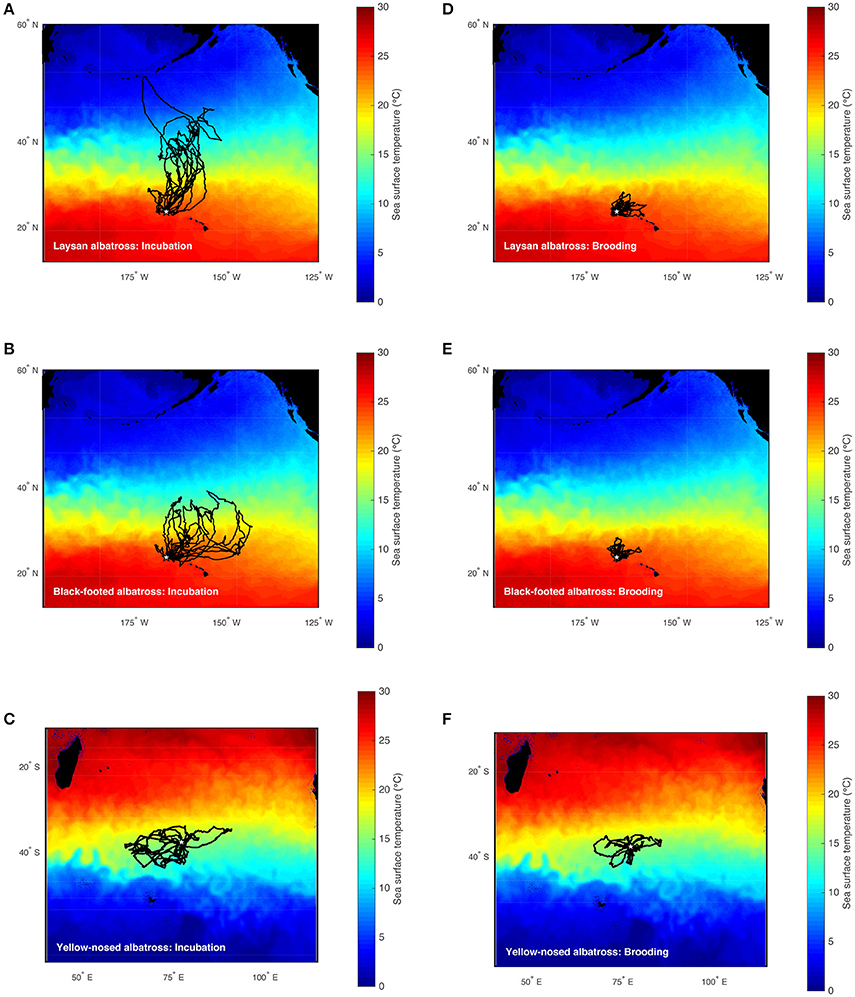
Figure 1 . Foraging trips of Laysan, black-footed, and Indian yellow-nosed albatrosses during late incubation (A–C) and brooding (D–F) . Laysan and black-footed albatrosses were tracked at Tern Island, Northwest Hawaiian Islands during 2002–2003, 2004–2005, and 2005–2006, and yellow-nosed albatrosses were tracked at Amsterdam Island, southern Indian Ocean, during 2006–2007 (study colonies indicated with a star). Tracks are superimposed on time-averaged Blended 5-day sea surface temperature (°C) for the respective study periods retrieved via the NOAA OceanWatch Live Access Server ( http://coastwatch.pfel.noaa.gov/erddap/ ).
We compared the foraging movements and activity patterns of Laysan, black-footed, and yellow-nosed albatrosses during the incubation and brooding periods, and measured energy expenditure during the brooding phase. By comparing closely related species with breeding locations that differ in terms of proximity to preferred marine habitats, we evaluated how these species respond behaviorally and physiologically to differing environmental conditions and reproductive demands. We hypothesized that during incubation, when all three species forage in productive subtropical-subpolar transition zones, activity patterns would be similar among species, but that differences would emerge during brooding when Hawaiian albatrosses are constrained to forage in an oligotrophic environment and competition for resources is likely high. Specifically, we hypothesized that Hawaiian albatrosses would spend more time in flight than yellow-nosed albatrosses during brooding, due to greater time spent in transit between more dispersed prey patches. We also hypothesized that Laysan and black-footed albatrosses would have lower landing rates and expend less energy at sea than yellow-nosed albatrosses during brooding, as a means of reducing energetic costs of foraging in a low-productivity environment.
Tracking Activities
We studied Laysan and black-footed albatrosses at Tern Island (23.87°N, 166.28°W), French Frigate Shoals, Northwest Hawaiian Islands during the 2002–2003, 2004–2005, and 2005–2006 breeding seasons, and Indian yellow-nosed albatrosses at Amsterdam Island (37.86°S, 77.52°E), Southern Indian Ocean, during the 2006-07 breeding season. We used satellite telemetry to determine at-sea locations of foraging albatrosses during late incubation and brooding to characterize differences in behavior between species and reproductive stages. During brooding, we also measured field metabolic rates of tracked albatrosses using the doubly labeled water technique ( Lifson and McClintock, 1966 ; Nagy, 1980 ; Speakman, 1997 ).
Seventy-five adult albatrosses were equipped with satellite platform terminal transmitters (30 g Pico-100, Microwave Telemetry, Columbia, MD; and 35 g SPOT4, Wildlife Computers, Redmond, WA) during late incubation (within 2 weeks of hatch date; 10 Laysan, 11 black-footed, 11 yellow-nosed albatrosses) and brooding (15 Laysan, 13 black-footed, 15 yellow-nosed albatrosses). Satellite tags were attached to dorsal feathers with adhesive tape (tesa ® , Hamburg, Germany), and satellite transmissions were downloaded via the Argos satellite system (Service Argos, Inc., Largo, MD). Individuals were also equipped with temperature recorders (10 g Lotek LTD 2400 and 1100, Lotek Wireless, St. John's, Newfoundland) attached to a plastic leg band so that temperature recordings (±0.05°C) could be used to characterize activity patterns while at sea ( Wilson et al., 1995 ). Foraging activity is only presented for the brooding period, when high-resolution (12 s) temperature records were available. In all cases, total mass of deployed devices was <2% of bird body mass, which is under the recommended limit for albatross tracking studies ( Phillips et al., 2003 ). Sex was determined from blood samples ( Fridolfsson and Ellegren, 1999 ) for all individuals tracked. All protocols employed in this study were approved by the Institutional Animal Care and Use Committees, University of California Santa Cruz.
Foraging Behavior
We delimited albatross foraging tracks based on visual observations of departure and arrival times from twice-daily nest checks during incubation, and hourly nest checks from dawn to dusk during brooding. To remove unlikely Argos locations, tracks were filtered using the Iknos Toolkit (Y. Tremblay, unpublished program) for Matlab (The MathWorks, Natick, MA), following Kappes et al. (2010) . First, a speed filter of 80 km h −1 was applied to transit rates between successive locations (following Hyrenbach et al., 2002 ; Suryan et al., 2006 ) to remove unrealistic flight speeds ( Spear and Ainley, 1997 ). Next, the maximum change in azimuth was set to 170° to remove track spikes between successive locations that are likely to be erroneous ( Keating, 1994 ; Freitas et al., 2008 ). Finally, to avoid errors in transit rate determination ( Hays et al., 2001 ), the minimum time between successive fixes was set to 10 min.
We calculated maximum distance traveled from the colony using great-circle distances to account for the earth's curvature. We divided great-circle distances between off-colony Argos locations by the time between successive locations to calculate average transit rate. To characterize albatross foraging activity patterns, we determined the proportion of time spent in flight and the frequency of landings on the sea surface. Landing rates are indicative of feeding effort ( Weimerskirch et al., 2000 ; Shaffer et al., 2001a ) because albatrosses must land on the sea surface in order to consume prey ( Conners et al., 2015 ). Previous research has demonstrated that take-offs and landings are the most energetically demanding activities albatrosses engage in at sea ( Weimerskirch et al., 2000 ), and landing rates of wandering albatrosses ( Diomedea exulans ) are correlated with field metabolic rates ( Shaffer et al., 2001a ). We used an algorithm (Iknos toolkit for Matlab; Y. Tremblay, unpublished program; Kappes et al., 2015 ) to identify landings based on rapid changes in temperature, and stable periods associated with sitting on the sea surface ( Wilson et al., 1995 ), for those individuals equipped with temperature records. We defined daylight hours based on civil twilight (sun no more than 6° below the horizon) using NOAA's solar calculator in the maptools package in R ( Lewin-Koh and Bivand, 2010 ) and temporally-matching to tracking locations.
Field Metabolic Rates
Doubly labeled water was used to determine field metabolic rates (FMR) of Laysan, black-footed, and yellow-nosed albatrosses at sea ( FMR at − sea ) and at the nest ( FMR on − nest ) during the brooding period ( Lifson and McClintock, 1966 ; Nagy, 1980 ; Speakman, 1997 ). Fifteen birds of each species were captured at the nest immediately following a mate switch, and an initial blood sample (0.5–3.5 ml) was collected from a vein on the tarsus. Albatrosses were given an intraperitoneal injection of 1.6–1.9 ml sterile water containing 0.9% NaCl, and either 34.5 atom percentage oxygen-18 and 35.9 atom percentage deuterium (Laysan and black-footed albatrosses), or 29.8 atom percentage oxygen-18 and 5.0 Mbq g −1 of tritiated water (yellow-nosed albatrosses). Mass of injectate (±0.01 g) was determined by weighing the syringe before and after injection using a portable field balance (Ohaus Corp., Pine Brook, NJ). Each bird was weighed to the nearest 50 g using either a spring-loaded Pesola (Pesola AG, Baar, Switzerland) or Salter scale (Salter Weightronix Ltd, West Bromwich, UK) and then placed in a box or holding pen; isotopes were allowed to equilibrate for ~90 min ( Shaffer et al., 2001b ) before a second blood sample was collected. Three Laysan, two black-footed, and three yellow-nosed albatrosses were held for 3 h to help ensure equilibration when there was evidence that injections may have been made into the gastrointestinal cavity or cutaneous fat. All individuals were equipped with satellite tags and/or temperature recorders and released at the nest. After completion of a foraging trip, each bird was recaptured and a third blood sample was collected, within 2–3 h of returning to the colony. Satellite tags and temperature recorders were then removed and final body mass was measured. To determine FMR on − nest , three Laysan, four black-footed, and three yellow-nosed albatrosses were subsequently captured after 2 days at the nest and a fourth blood sample was collected from these individuals. In one instance, a Laysan albatross did not depart to sea after release, but instead switched with its mate again and remained on the nest; for this individual only FMR on − nest was calculated. This double-switching behavior was also observed at Laysan and black-footed albatross nests checked daily to determine attendance patterns of control individuals.
Due to equipment limitations, one black-footed albatross was equipped with a temperature recorder only, two yellow-nosed albatrosses were equipped with satellite tags only, and one black-footed albatross was equipped with a GPS tag (TechnoSmart, Rome, Italy) rather than a satellite tag; in this case, the GPS record demonstrated that the individual stayed near Tern Island overnight and then returned to the nest (short departures were also observed in control pairs, with both members of the pair remaining at the nest for several days in some cases). As this was not representative of FMR at − sea or FMR on − nest , the metabolic rate measured for this individual is not included in the subsequent analyses.
All blood samples were collected with a syringe and 21–25 gauge needle, transferred to a vacutainer (B-D brand with spray-coated lithium heparin, Beckton-Dickinson, Franklin Lakes, NJ) and stored in a cooler with cold packs until centrifugation on the day of collection. Plasma was transferred to 2 ml cryogenic plastic screw cap vials (with silicon O-rings; Corning Inc., Corning, NY) and frozen until isotopic analyses were performed. Aliquots of water distilled from plasma samples (following Ortiz et al., 1978 ) were then used to determine specific activity of deuterium by laser-absorption spectroscopy (University of California Davis, Davis, CA) or tritium by scintillation spectrometry (LS 6500, Beckman Coulter Inc., Fullterton, CA) in triplicate; specific activity of oxygen-18 was determined by mass ratio spectrometry (Metabolic Solutions, Nashua, NH).
The initial dilution space of oxygen-18 was used to calculate the volume of initial total body water. To calculate final body water volume, body mass at recapture was multiplied by the initial fractional water content, which has been validated in Shaffer et al. (2006) . CO 2 production was calculated using equation 2 in Nagy (1980) ; this equation assumes that body mass of the animal changes linearly with time. For two yellow-nosed albatrosses and one Laysan albatross, FMR was determined using the single sample method described in Speakman (1997) , because initial total body water calculations suggested isotopes were not fully equilibrated when the post-equilibration blood sample was collected. FMR (mL g −1 h −1 ) was converted to kJ (and W) by applying a conversion factor of 24.7 kJ = 1 L CO 2 following ( Pettit et al., 1988 ), based on chemical composition of the diet of Laysan albatrosses ( Harrison et al., 1983 ); this conversion factor was assumed to approximate CO 2 yield from the diet of black-footed and yellow-nosed albatrosses. Mass-specific FMR (W kg −1 ) was determined by dividing FMR by mean body mass so that energy expenditure is more directly comparable among species. Because FMR calculations included time spent at the nest before departure and after arrival at the nest, measured FMR was corrected based on visual observations of departure and arrival times at the nest. Following methods of Costa and Prince (1987) , FMR at − sea was calculated as:
We compiled average FMR and body mass values for Hawaiian and yellow-nosed albatrosses with values from other studies (male and female wandering albatrosses ( Shaffer et al., 2001a ), shy albatrosses ( Thalassarche cauta ; Green and Brothers, 1995, Abstract from First International Albatross and Petrel Conference, Hobart, Australia), gray-headed albatrosses (T. chrysostoma ; Costa and Prince, 1987 ), black-browed albatrosses ( Thalassarche melanophrys ; Shaffer et al., 2004 ), and Laysan albatrosses during incubation ( Pettit et al., 1988 ) to provide a mechanism for comparing energy expenditure among species ( Shaffer, 2011 ). Given large differences in mass between wandering albatrosses and other studied albatross species, we tested for the effect of genus Diomedea in the relationship between log-transformed FMR and body mass values.
Statistical Analysis
Statistical analyses were implemented in the program R ( R Development Core Team, 2010 ). We used ANOVA and Tukey multiple comparison tests ( Hothorn et al., 2008 ) to investigate differences in foraging behavior between species and reproductive stages, and species differences in FMR, total body water, body mass, and foraging activity; Bonferroni-corrected P -values are presented for multiple comparisons. Trip characteristics, landing rates, time in flight, total body water, and FMR for each species did not differ significantly between the sexes, therefore males and females were grouped for all analyses. To meet normality assumptions, percent time in flight was arcsine transformed and landing rate was log transformed prior to analysis. Simple linear regression was used to investigate relationships between foraging behavior and FMR at − sea for each species, and to examine the allometric relationship between log-transformed FMR and body mass for albatrosses from this and other studies. P -values reported are two-tailed, and the significance level was set as P = 0.05. All averages are reported as Mean ± SD .
Hawaiian albatrosses traveled significantly farther during incubation compared to brooding (more than four times as far on average; Table 1 ; P < 0.001 for pair-wise tests), whereas maximum foraging range did not differ between incubation and brooding for yellow-nosed albatrosses ( P = 0.08). All three albatross species took foraging trips of longer duration during incubation compared to brooding ( P < 0.01 for all pair-wise tests): Hawaiian albatrosses took trips that were more than three times longer, whereas yellow-nosed albatrosses took trips that were just under two times longer on average (Table 1 ). During the incubation period, the majority of Hawaiian albatrosses traveled north of the Tern Island colony to cooler waters of the North Pacific Transition Zone; during brooding, their movements were restricted to warmer waters of the subtropical gyre (Figures 1 , 2 ). Yellow-nosed albatrosses foraged in similar thermal environments during the incubation and brooding periods (Figure 2 ). Black-footed albatrosses traveled more rapidly during incubation compared to brooding ( P = 0.02); transit rates did not differ between breeding stages for Laysan and yellow-nosed albatrosses (Table 1 ).
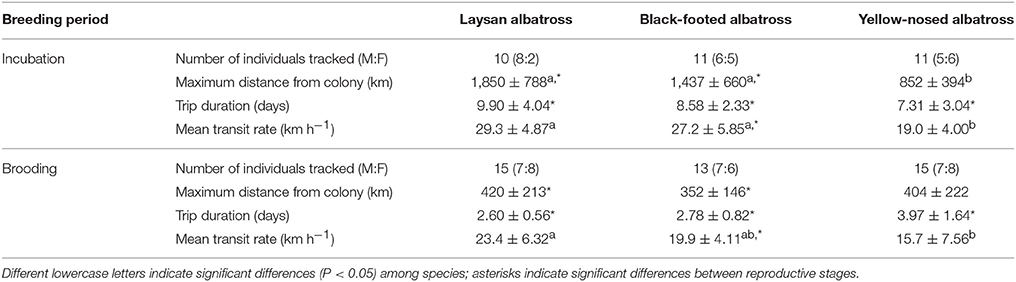
Table 1 . Summary characteristics (Mean ± SD ) of foraging trips of Laysan and black-footed albatrosses tracked at Tern Island, Northwest Hawaiian Islands, and Indian yellow-nosed albatrosses tracked at Amsterdam Island, southern Indian Ocean, during late incubation and brooding.
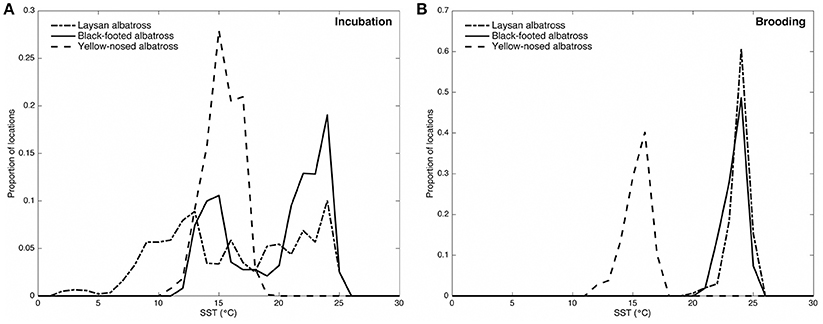
Figure 2 . Distribution of sea surface temperatures (°C) along Laysan, black-footed, and Indian yellow-nosed albatrosses foraging routes during incubation (A) and brooding (B) . Laysan and black-footed albatrosses were tracked at Tern Island, Northwest Hawaiian Islands during 2002–2003, 2004–2005, and 2005–2006, and yellow-nosed albatrosses were tracked at Amsterdam Island, southern Indian Ocean, during 2006–2007. Blended 5-day sea surface temperature retrieved via the NOAA OceanWatch Live Access Server ( http://coastwatch.pfel.noaa.gov/erddap/ ).
During the incubation period, Hawaiian albatrosses traveled farther (over 700 km on average) and more rapidly (9 km h −1 on average) than yellow-nosed albatrosses ( P < 0.02 for pair-wise tests), but spent a similar amount of time at sea (between 7 and 10 days on average). During brooding, all three species traveled similar distances (~400 km) and durations (3–4 days). Laysan albatrosses traveled more rapidly than yellow-nosed albatrosses during brooding (8 km h −1 on average; P = 0.004), but this was the only species difference in transit rate observed. Sea surface temperature along foraging tracks was most similar among species during incubation; Hawaiian albatrosses used warmer waters than yellow-nosed albatrosses during brooding (Figure 2 ).
Percent time in flight and landing rates did not differ between reproductive stages for any of the three species (Table 2 ). During incubation, Hawaiian albatrosses spent more time in flight at night (70–80%) compared to yellow-nosed albatrosses (~50%; Table 2 ; P < 0.01 for pair-wise tests). During brooding, Hawaiian albatrosses spent more time in flight both during the day (~90%) and at night (80–90%) compared to yellow-nosed albatrosses (~70% during day; ~40% at night; Table 2 ; P < 0.01 for pair-wise tests). Yellow-nosed albatrosses had higher overall and daytime landing rates than black-footed albatrosses during incubation ( P < 0.001), but there were no species differences in overall or daytime landing rates during brooding. All three species demonstrated diel patterns in foraging activity (Table 2 ).
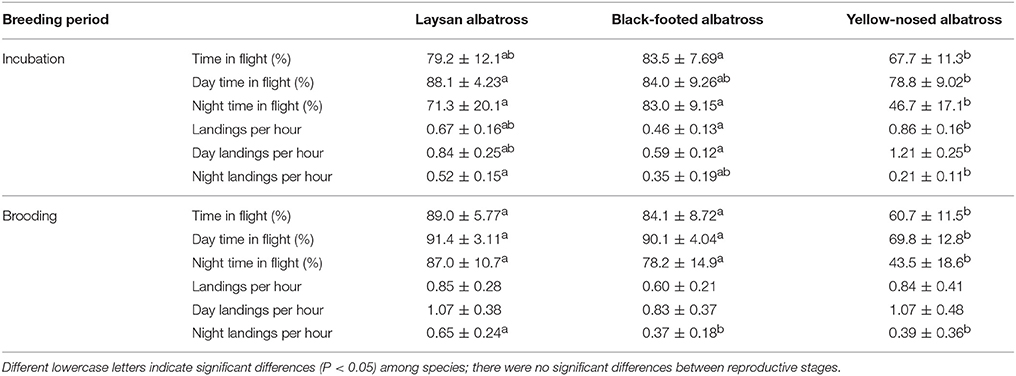
Table 2 . Summary characteristics (Mean ± SD ) of at-sea activity patterns of Laysan and black-footed albatrosses breeding at Tern Island, Northwest Hawaiian Islands, and Indian yellow-nosed albatrosses breeding at Amsterdam Island, southern Indian Ocean, during late incubation and brooding.
Body mass differed between the three species studied; black-footed albatrosses were significantly heavier than Laysan albatrosses ( P < 0.001), which were significantly heavier than yellow-nosed albatrosses ( P = 0.002; Table 3 ). Total body water (%) did not differ significantly between Laysan and black-footed albatrosses, but each species of Hawaiian albatross had a lower percentage of total body water compared to yellow-nosed albatrosses ( P < 0.001 for pair-wise tests). On average, albatrosses gained mass during the foraging trip but the change in mass did not differ between species and was highly variable among individuals (Table 3 ).
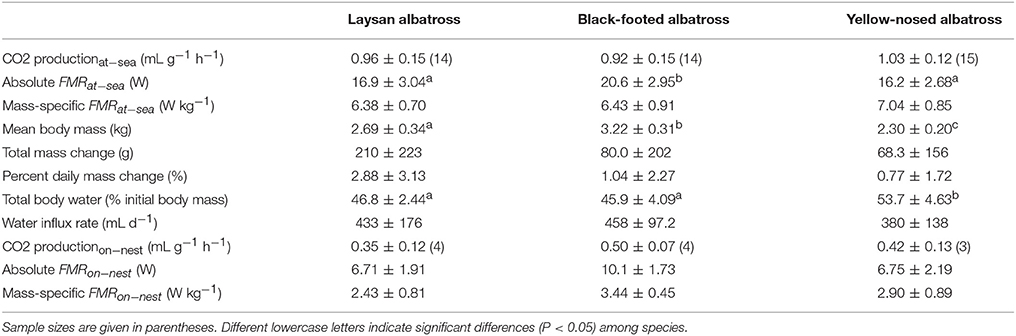
Table 3 . Energy expenditure (Mean ± SD ) of Laysan and black-footed albatrosses breeding at Tern Island, Northwest Hawaiian Islands, and Indian yellow-nosed albatrosses breeding at Amsterdam Island, southern Indian Ocean, during the brooding period.
Mean absolute FMR at − sea (W) was greatest for black-footed albatrosses ( P = 0.005, Laysan pair-wise test; P < 0.001, yellow-nosed pair-wise test), and similar for Laysan and yellow-nosed albatrosses (Table 3 ). Mean absolute FMR on − nest (W) did not differ between albatross species but sample sizes were low for this parameter (Table 3 ). Mass-specific FMR at − sea (W kg −1 ) and FMR on − nest (W kg −1 ) did not differ between species. The ratio of FMR at − sea to FMR on − nest was lowest for black-footed albatrosses (1.9), and similar for Laysan (2.6) and yellow-nosed albatrosses (2.4).
For each species, we investigated the relationship between at-sea behavior and mass-specific field metabolic rates, however, FMR at − sea (W kg −1 ) was not statistically related to foraging range, trip duration, transit rates, the percent time in flight, the number or frequency of landings, change in mass, or water influx rates.
We plotted the allometric relationship between FMR and body mass for albatrosses from this and other doubly labeled water studies to provide a mechanism for appropriately comparing energy expenditure among species (Figure 3 ; Shaffer, 2011 ). We found a significant effect of the genus Diomedea on the relationship between log-transformed FMR and body mass [β = 0.60, t (6) = 3.19, P = 0.02], therefore our discussion focuses on the allometric relationship of the smaller albatross species (solid line; Figure 3 ) rather than the line for all studied species (dashed line; Figure 3 ). We found that Hawaiian albatrosses during brooding fall below the regression line for smaller albatross species, whereas yellow-nosed albatrosses fall above this line (Figure 3 ).
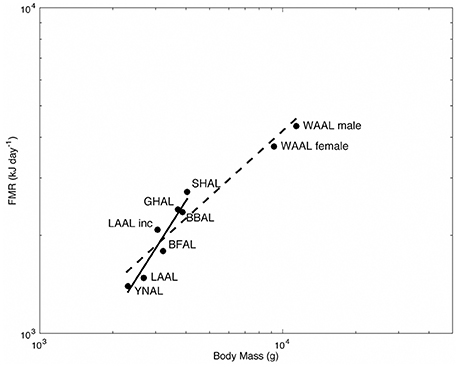
Figure 3 . Allometry of field metabolic rate (FMR; kJ d −1 ) for albatrosses measured using the doubly labeled water method. FMR and body mass (g) are plotted on a logarithmic scale for WAAL male and female (wandering albatross Diomedea exulans ; Shaffer et al., 2001a ), SHAL (shy albatross Thalassarche cauta ; Green and Brothers 1995, Abstract from First International Albatross and Petrel Conference, Hobart, Australia), GHAL (gray-headed albatross T. chrysostoma ; Costa and Prince, 1987 ), BBAL (black-browed albatross T. melanophrys ; Shaffer et al., 2004 ), LAAL Inc. (Laysan albatross Phoebastria immutabilis during incubation; Pettit et al., 1988 ), and BFAL (black-footed albatross P. nigripes ), LAAL (Laysan albatross), and YNAL (Indian yellow-nosed albatross T. carteri ) during brooding (this study).
Comparative Foraging Behavior
During the incubation period, all three albatross species foraged within convergence zones between warm subtropical waters and cool subpolar waters (Figure 1 ) where productivity is regionally enhanced ( Lutjeharms and Valentine, 1984 ; Barange et al., 1998 ; Read et al., 2000 ; Olson, 2001 ; Polovina et al., 2001 ) and albatross prey resources are aggregated ( Harrison et al., 1983 ; Gong et al., 1993 ; Yatsu et al., 1993 ; Pearcy et al., 1996 ; Pinaud et al., 2005 ; Conners, 2015 ). To reach these habitats, Laysan and black-footed albatrosses traveled significantly farther than yellow-nosed albatrosses and spent more time at sea. During brooding, all species reduced time at sea and Hawaiian albatrosses retracted their foraging ranges to the warm, oligotrophic environment close to the breeding colony (Figures 1 , 2 ), where prey abundance is likely lower ( Ashmole, 1971 ; Ballance et al., 1997 ). Conversely, yellow-nosed albatrosses were able to forage in a similar thermal environment during both reproductive stages, despite restricted movements during brooding (Figures 1 , 2 ).
Hawaiian albatrosses spent more time in flight than yellow-nosed albatrosses, especially at night and during the brooding period. This is in agreement with our hypothesis, as we expected that spending more time in flight would be suitable for foraging in a low-productivity environment with unpredictable prey resources ( Weimerskirch et al., 2005 ). Reliance on flight to travel between dispersed prey patches is seen in other tropical seabirds where greater flight proficiency is associated with lower productivity habitats ( Ballance et al., 1997 ). Compared to other albatross species that forage in more productive marine habitats such as subtropical and polar convergences, continental shelf-breaks and slopes, and coastal upwelling zones ( Tickell, 2000 ; BirdLife International, 2004 ), yellow-nosed albatrosses spent a similar proportion of time in flight (44–69%; Weimerskirch and Guionnet, 2002 ; Phalan et al., 2007 ). Contrary to our hypothesis, we did not detect a consistent difference in overall landing rates between Hawaiian albatrosses and yellow-nosed albatrosses during brooding, despite contrasting foraging environments. Landing rates during brooding were similar between species during the day, but higher for Laysan albatrosses at night. Higher landing rates at night for Laysan albatrosses may be related to nocturnal feeding in this species ( Harrison et al., 1983 ; Conners et al., 2015 ). The tendency of all species to forage during daylight hours is supported by previous research ( Fernández and Anderson, 2000 ; Weimerskirch and Guionnet, 2002 ; Kappes et al., 2015 ).
Foraging Energetics
Brooding Laysan and black-footed albatrosses did not differ in terms of body composition; however, both Hawaiian albatrosses demonstrated significantly lower total body water when compared to yellow-nosed albatrosses. Lower total body water suggests that Hawaiian albatrosses have comparatively greater lipid reserves ( Reilly and Fedak, 1990 ; Ellis and Jehl, 1991 ; Groscolas et al., 1991 ), which may be related to foraging in a low-productivity environment. If foraging conditions are poor during brooding, adults may rely on lipid body stores obtained during the incubation period for self-maintenance and then allocate food resources acquired at sea during the brooding period to rapidly-growing chicks ( Weimerskirch and Lys, 2000 ).
Mean absolute FMR at − sea was greatest for black-footed albatrosses, and similar for Laysan and yellow-nosed albatrosses. Greater absolute FMR at − sea in black-footed albatrosses can be explained by larger body size ( Nagy, 2005 ) and higher wing loading in this species ( Suryan et al., 2008 ). Contrary to our prediction, Laysan and black-footed albatrosses did not exhibit lower mass-specific FMR at − sea compared to yellow-nosed albatrosses. We expected that Hawaiian albatrosses would minimize foraging costs by employing a comparatively economical foraging strategy in response to sparse, unpredictable local prey resources ( Flint and Nagy, 1984 ; Weimerskirch et al., 2005 ). We therefore also combined our results with published research and examined residual variation in the allometric relationship between body mass and FMR at − sea to further evaluate the comparative energy expenditure of Hawaiian and yellow-nosed albatrosses (discussed below).
We did not find significant relationships between FMR at − sea and foraging range, trip duration, transit rates, the percent time in flight, the number or frequency of landings, change in mass, or water influx rates within each species. As predicted, Hawaiian albatrosses spent more time in flight than yellow-nosed albatrosses, however, we were not able to detect a relationship between time in flight and energetic costs within species. During brooding, overall landing rates did not differ between species and were highly variable. Previous research using the doubly labeled water method demonstrated a relationship between energetic costs and landing rates in wandering albatrosses ( Shaffer et al., 2001a ), therefore we expected that landing rates would be related to energy expenditure within species in this study. The lack of a relationship between landing rates and field metabolic rates may be explained by the relatively smaller size of Hawaiian and yellow-nosed albatrosses (2–4 kg) compared to the larger wandering albatross (8–10 kg; Tickell, 1968 ).
While field metabolic rates of black-footed and yellow-nosed albatrosses have not been studied during incubation, Pettit et al. (1988) measured FMR at − sea and FMR on − nest of Laysan albatrosses at Tern Island during the incubation period. Estimates of FMR on − nest during incubation were similar to our estimates of FMR on − nest during brooding, however, estimates of FMR at − sea during incubation were higher than our estimates of FMR at − sea during brooding for all three species ( Pettit et al., 1988 ; Figure 3 ). This contrasts with a study of wandering albatrosses, where FMR at − sea was higher during brooding, compared to the incubation period ( Shaffer et al., 2003 ). Although estimated FMR at − sea was greater during incubation for Laysan albatrosses, activity patterns during incubation and brooding were similar (this study; Kappes et al., 2015 ). This provides further evidence that activity patterns do not relate directly to energy expenditure in this species, contrary to findings for wandering albatrosses ( Weimerskirch et al., 2000 ; Shaffer et al., 2001a ). Higher FMR at − sea during the incubation period could reflect effort directed at assimilating lipid stores while foraging at distant, preferred habitats ( Welcker et al., 2009 ), so that adults are able to effectively provision young chicks while foraging in an oligotrophic marine habitat during brooding.
To further examine variation in energy expenditure among species, we evaluated the allometric relationship between FMR and body mass for smaller albatross species and examined where Hawaiian and yellow-nosed albatrosses fell in relation to the plotted regression line (Figure 3 ). We found that Hawaiian albatrosses during brooding fell below the regression line, whereas yellow-nosed albatrosses fell above this line (Figure 3 ). This indicates that Hawaiian albatrosses expend comparatively less energy at sea during brooding after accounting for species differences in mass, which may be related to foraging in an oligotrophic environment during this reproductive stage. It may also be related to the fact that Hawaiian albatrosses breed during boreal winter when winds are stronger, compared to conditions during the Indian Ocean austral summer when yellow-nosed albatrosses breed. Laysan albatrosses during incubation fall well above the regression line, indicating that this species expends comparatively more energy during this reproductive stage, which may be related to effort directed at assimilating lipid stores in distant, preferred habitats.
Conclusions
Among albatrosses, Hawaiian albatrosses are unique in that they are constrained to forage in a warm, oligotrophic marine environment during the energetically demanding brooding period. As hypothesized, Hawaiian albatrosses spent more time in flight than yellow-nosed albatrosses during brooding, a behavior suited for traveling between dispersed prey patches. Contrary to our predictions, we did not detect species differences in overall landing rates or mass-specific FMR at − sea during brooding, measures indicative of foraging energy expenditure. However, compared to yellow-nosed albatrosses, Hawaiian albatrosses had lower total body water (greater lipid reserves) and field metabolic rates that fell below the allometric relationship for studied albatross species, attributes which may reflect physiological adaptations of these species to foraging in a low-productivity environment. Given the relative lack of information on the physiological constraints of species movements ( Hays et al., 2016 ), our comparative approach provides a valuable case study as to how a group of related species responds physiologically and behaviorally to differing environmental conditions and reproductive demands.
Data Availability
Satellite tracking datasets generated from this research are available by request from the BirdLife Tracking Ocean Wanderers database ( http://seabirdtracking.org ).
Author Contributions
All authors participated in study design and interpretation of results. MA and YT: collected tracking data; MA, DC, and SS: implemented the doubly labeled water technique in the field; MA: performed laboratory work, conducted data analysis, and implemented statistical analyses; MA: wrote the first draft of the manuscript, and all authors contributed substantially to revisions.
This research was part of the Tagging of Pacific Pelagics (TOPP) program, funded in part by the National Ocean Partnership Program (N00014-02-1-1012), the Office of Naval Research (N00014-00-1-0880 & N00014-03-1-0651), the Gordon and Betty Moore, David and Lucille Packard, and Alfred P. Sloan Foundations. MA was supported by a Fulbright grant to France, a Chancellor's Dissertation-Year Fellowship and a UCSC Regents Fellowship, and grants from the ARCS Foundation, the Earl H. Myers and Ethel M. Myers Trust, Friends of Long Marine Lab, the Society for Integrative and Comparative Biology, the STEPS Institute, and the UCSC Ecology & Evolutionary Biology department. Cambridge Isotope Laboratories, Inc. provided deuterium and oxygen-18 isotopes for this study as a research award. S & A Machine and Tool Co. generously provided overseas shipping costs of research supplies for use on Amsterdam Island.
Conflict of Interest Statement
The authors declare that the research was conducted in the absence of any commercial or financial relationships that could be construed as a potential conflict of interest.
The reviewer GS and handling Editor declared their shared affiliation.
Acknowledgments
We thank Jill Awkerman, Sarah Chisholm, Peter Kappes, Nicolas Mignot, Scott Seganti, and Jean-Baptiste Thiebot for invaluable assistance in the field, and Lindsay Young for conducting genetic sexing. We are grateful to the Hawaiian Islands National Wildlife Refuge, U.S. Fish and Wildlife Service, Department of the Interior, for logistic support and permission to conduct research on Tern Island; to L'Institut Polaire Français and Terres Australes et Antarctiques Françaises for supporting field activities on Amsterdam Island; and to the Fulbright Commission for supporting research on Amsterdam Island, and while in residence at Centre d'Études Biologiques de Chizé (CNRS).
Alerstam, T., and Högstedt, G. (1982). Bird migration and reproduction in relation to habitats for survival and breeding. Ornis Scand. 13, 25–37. doi: 10.2307/3675970
CrossRef Full Text | Google Scholar
Ashmole, N. P. (1971). “Seabird ecology and the marine environment,” in Avian Biology , eds D. S. Farner and J. R. King (London: Academic Press), 223–286.
Google Scholar
Backus, R. H. (1986). “Biogeographic boundaries in the open ocean,” in Pelagic Biogeography , Vol. 49, eds A. C. Pierrot-Bults, S. van der Spoel, B. J. Zahuranec, and R. K. Johnson (UNESCO Technical Paper in Marine Science), 9–13.
Ballance, L. T., Pitman, R. L., and Reilly, S. B. (1997). Seabird community structure along a productivity gradient: importance of competition and energetic constraint. Ecology 78, 1502–1518. doi: 10.1890/0012-9658(1997)078[1502:SCSAAP]2.0.CO;2
Barange, M., Pakhomov, E. A., Perissinotto, R., Froneman, P. W., Verheye, H. M., Taunton-Clark, J., et al. (1998). Pelagic community structure of the subtropical convergence region south of Africa and in the mid-Atlantic Ocean. Deep Sea Res. I 45, 1663–1687. doi: 10.1016/S0967-0637(98)00037-5
BirdLife International (2004). “Tracking ocean wanderers: the global distribution of albatrosses and petrels,” in Results from the Global Procellariiform Tracking Workshop, (1-5 September, 2003) (Gordon's Bay; Cambridge, UK: BirdLife International).
Charnov, E. L. (1976). Optimal foraging, the marginal value theorem. Theor. Popul. Biol. 9, 129–136. doi: 10.1016/0040-5809(76)90040-X
PubMed Abstract | CrossRef Full Text | Google Scholar
Conners, M. G. (2015). Comparative Behavior, Diet, and Post-Breeding Strategies of Two Sympatric North Pacific albatross Species (Phoebastria sp.) . Ph.D. dissertation, University of California, Santa Cruz.
Conners, M. G., Hazen, E. L., Costa, D. P., and Shaffer, S. A. (2015). Shadowed by scale: subtle behavioral niche partitioning in two sympatric, tropical breeding albatross species. Mov. Ecol. 3, 28. doi: 10.1186/s40462-015-0060-7
Costa, D. P. (1991). Reproductive and foraging energetics of high latitude penguins, albatrosses and pinnipeds: implications for life history patterns. Am. Zool. 31, 111–130. doi: 10.1093/icb/31.1.111
Costa, D. P. (1993). The relationship between reproductive and foraging energetics and the evolution of the Pinnipedia. Symp. Zool. Soc. Lond. 66, 293–314.
Costa, D. P., and Prince, P. A. (1987). Foraging energetics of gray-headed albatrosses Diomedea Chrysostoma at Bird Island, South Georgia. Ibis 129, 149–158. doi: 10.1111/j.1474-919X.1987.tb03196.x
Costa, D. P., and Shaffer, S. A. (2008). “Physiological constraints on the foraging ecology and energetics of albatrosses and other large seabirds,” in 4th CPB Meeting in Africa: Mara 2008. Molecules to Migration: The Pressures of Life , eds S. Morris and A. Vosloo (Bologna: Medimond Publishing).
Costa, D. P., and Shaffer, S. A. (2012). “Seabirds and marine mammals,” in Metabolic Ecology: A Scaling Approach , eds R. M. Sibly, J. H. Brown, and A. Kodric-Brown (Oxford: John Wiley & Sons, Ltd.), 225–233.
Croll, D. A., Marinovic, B., Benson, S., Chavez, F. P., Black, N., Ternullo, R., et al. (2005). From wind to whales: trophic links in a coastal upwelling system. Mar. Ecol. Prog. Ser. 289, 117–130. doi: 10.3354/meps289117
Ellis, H. I., and Jehl, J. R. (1991). Total body water and body composition in phalaropes and other birds. Physiol. Zool. 64, 973–984. doi: 10.1086/physzool.64.4.30157952
Emlen, J. M. (1966). The role of time and energy in food preference. Am. Nat. 100, 611–617. doi: 10.1086/282455
Fauchald, P., Erikstad, K. E., and Skarsfjord, H. (2000). Scale-dependent predator-prey interactions: the hierarchical spatial distribution of seabirds and prey. Ecology 81, 773–783. doi: 10.3354/meps231279
Fernández, P., and Anderson, D. J. (2000). Nocturnal and diurnal foraging activity of Hawaiian albatrosses detected with a new immersion monitor. Condor 102, 577–584. doi: 10.1650/0010-5422(2000)102[0577:NADFAO]2.0.CO;2
Fernández, P., Anderson, D. J., Sievert, P. R., and Huyvaert, K. P. (2001). Foraging destinations of three low-latitude albatross ( Phoebastria ) species. J. Zool. 254, 391–404. doi: 10.1017/S0952836901000899
Flint, E. N., and Nagy, K. A. (1984). Flight energetics of free-living sooty terns. Auk 101, 288–294.
Franks, P. J. S. (1992). Sink or swim: accumulation of biomass at fronts. Mar. Ecol. Prog. Ser. 82, 1–12. doi: 10.3354/meps082001
Freitas, C., Lydersen, C., Fedak, M. A., and Kovacs, K. M. (2008). A simple new algorithm to filter marine mammal Argos locations. Mar. Mamm. Sci. 24, 315–325. doi: 10.1111/j.1748-7692.2007.00180.x
Fridolfsson, A.-K., and Ellegren, H. (1999). A simple and universal method for molecular sexing of non-ratite birds. J. Avian Biol. 30, 116–121. doi: 10.2307/3677252
Gong, Y., Kim, S., and An, D. H. (1993). Abundance of neon flying squid in relation to oceanographic conditions in the North Pacific. Int. N. Pac. Fish. Comm. Bull. 53, 191–204.
Govoni, J. J., and Grimes, C. B. (1992). The surface accumulation of larval fishes by hydrodynamic convergence within the Mississippi River plume front. Cont. Shelf Res. 12, 1265–1276. doi: 10.1016/0278-4343(92)90063-P
Groscolas, R., Schreiber, L., and Morin, F. (1991). The use of tritiated water to determine protein and lipid utilization in fasting birds: a validation study in incubating great-winged petrels, Pterodroma macroptera . Physiol. Zool. 64, 1217–1233. doi: 10.1086/physzool.64.5.30156241
Guinet, C., Koudil, M., Bost, C.-A., Durbec, J. P., Georges, J. Y., Mouchot, M. C., et al. (1997). Foraging behaviour of satellite-tracked king penguins in relation to sea-surface temperatures obtained by satellite telemetry at Crozet Archipelago, a study during three austral summers. Mar. Ecol. Prog. Ser. 150, 11–20. doi: 10.3354/meps150011
Harding, A., Paredes, R., Suryan, R., Roby, D., Irons, D., Orben, R., et al. (2013). Does location really matter? An inter-colony comparison of seabirds breeding at varying distances from productive oceanographic features in the Bering Sea. Deep-Sea Res. II 94, 178–191. doi: 10.1016/j.dsr2.2013.03.013
Harrison, C. S., Hida, T. S., and Seki, M. P. (1983). Hawaiian seabird feeding ecology. Wildlife Wildl. Monogr. 85, 1–71.
Hays, G. C., Åkesson, S., Godley, B. J., Luschi, P., and Santidrian, P. (2001). The implications of location accuracy for the interpretation of satellite-tracking data. Anim. Behav. 61, 1035–1040. doi: 10.1006/anbe.2001.1685
CrossRef Full Text
Hays, G. C., Ferreira, L. C., Sequeira, A. M. M., Meekan, M. G., Duarte, C. M., Bailey, H., et al. (2016). Key questions in marine megafauna movement ecology. Trends Ecol. Evol. 31, 463–475. doi: 10.1016/j.tree.2016.02.015
Hothorn, T., Bretz, F., and Westfall, P. (2008). Simultaneous inference in general parametric models. Biometr. J. 50, 346–363. doi: 10.1002/bimj.200810425
Houston, A. I. (1993). The efficiency of mass loss in breeding birds. Proc. R. Soc. Lond. B 254, 221–225. doi: 10.1098/rspb.1993.0149
Hunt, G. L. (1997). Physics, zooplankton, and the distribution of least auklets in the Bering Sea - a review. ICES J. Mar. Sci. 54, 600–607. doi: 10.1006/jmsc.1997.0267
Hunt, G. L., Russell, R. W., Coyle, K. O., and Weingartner, T. (1998). Comparative foraging ecology of planktivorous auklets in relation to ocean physics and prey availability. Mar. Ecol. Prog. Ser. 167, 241–259. doi: 10.3354/meps167241
Hyrenbach, K. D., Fernandez, P., and Anderson, D. J. (2002). Oceanographic habitats of two sympatric North Pacific albatrosses during the breeding season. Mar. Ecol. Prog. Ser. 233, 283–301. doi: 10.3354/meps233283
Jouventin, P., and Weimerskirch, H. (1990). Satellite tracking of wandering albatrosses. Nature 343, 746–748. doi: 10.1038/343746a0
Kappes, M. A., Shaffer, S. A., Tremblay, Y., Foley, D. G., Palacios, D. M., Bograd, S. J., et al. (2015). Reproductive constraints influence habitat accessibility, segregation, and preference of sympatric albatross species. Mov. Ecol. 3:34. doi: 10.1186/s40462-015-0063-4
Kappes, M. A., Shaffer, S. A., Tremblay, Y., Foley, D. G., Palacios, D. M., Robinson, P. W., et al. (2010). Hawaiian albatrosses track interannual variability of marine habitats in the North Pacific. Prog. Oceanogr. 86, 246–260. doi: 10.1016/j.pocean.2010.04.012
Keating, K. A. (1994). An alternative index of satellite telemetry location error. J. Wildl. Manage. 58, 414–421. doi: 10.2307/3809311
Keiper, C. A., Ainley, D. G., Allen, S. G., and Harvey, J. T. (2005). Marine mammal occurrence and ocean climate off central California, 1986 to 1994 and 1997 to 1999. Mar. Ecol. Prog. Ser. 289, 285–306. doi: 10.3354/meps289285
Levins, R. (1968). Evolution in Changing Environments: Some Theoretical Explorations . Princeton, NJ: Princeton University Press.
Lewin-Koh, N. J., and Bivand, R. (2010). Maptools: Tools for Reading and Handling Spatial Objects. R Package Version 0.7-34.
Lifson, N., and McClintock, R. (1966). Theory of use of the turnover rates of body water for measuring energy and material balance. J. Theor. Biol. 12, 46–74. doi: 10.1016/0022-5193(66)90185-8
Lutjeharms, J. R. E., and Valentine, H. R. (1984). Southern Ocean thermal fronts south of Africa. Deep Sea Res. 31, 1461–1475. doi: 10.1016/0198-0149(84)90082-7
MacArthur, R. H., and Pianka, E. R. (1966). On optimal use of a patchy environment. Am. Nat. 100, 603–609. doi: 10.1086/282454
MacKas, D. L., and Boyd, C. M. (1979). Spectral analysis of zooplankton spatial heterogeneity. Science 204, 62–64. doi: 10.1126/science.204.4388.62
Nagy, K. A. (1980). CO 2 production in animals: analysis of potential errors in the doubly labeled water method. Am. J. Physiol. 238, R466–R473.
PubMed Abstract | Google Scholar
Nagy, K. A. (2005). Field metabolic rate and body size. J. Exp. Biol. 208, 1621–1625. doi: 10.1242/jeb.01553
Olson, D. B. (2001). Biophysical dynamics of western transition zones: a preliminary synthesis. Fish. Oceanogr. 10, 133–150. doi: 10.1046/j.1365-2419.2001.00161.x
Olson, D. B., and Backus, R. H. (1985). The concentrating of organisms at fronts: a cold-water fish and a warm-core ring. J. Mar. Res. 43, 113–137. doi: 10.1357/002224085788437325
Olson, D. B., Hitchcock, G. L., Mariano, A. J., Ashjian, C. J., Peng, G., Nero, R. W., et al. (1994). Life on the edge: marine life and fronts. Oceanography 7, 52–59. doi: 10.5670/oceanog.1994.03
Olsson, O., and Bolin, A. (2014). A model for habitat selection and species distribution derived from central place foraging theory. Oecologia 175, 537–548. doi: 10.1007/s00442-014-2931-9
Orians, G. H., and Pearson, N. E. (1979). “On the theory of central place foraging,” in Analysis of Ecological Systems , eds D. J. Horn, G. R. Stairs, and R. D. Mitchell (Columbus, OH: Ohio State University Press), 155–177.
Ortiz, C. L., Costa, D. P., and Le Boeuf, B. J. (1978). Water and energy flux in elephant seal pups fasting under natural conditions. Physiol. Zool. 51, 166–178. doi: 10.1086/physzool.51.2.30157864
Pearcy, W. G., Fisher, J. P., Anma, G., and Meguro, T. (1996). Species associations of epipelagic nekton of the North Pacific Ocean, 1978-1993. Fish. Oceanogr. 5, 1–20. doi: 10.1111/j.1365-2419.1996.tb00013.x
Pennycuick, C. J. (1982). The flight of petrels and albatrosses ( Procellariiformes ), observed in South Georgia and its vicinity. Philos. Trans. R. Soc. Lond. B 300, 75–106. doi: 10.1098/rstb.1982.0158
Pennycuick, C. J., Croxall, J. P., and Prince, P. A. (1984). Scaling of foraging radius and growth rate in petrels and albatrosses ( Procellariiformes ). Ornis Scand. 15, 145–154. doi: 10.2307/3675955
Pettit, T. N., Nagy, K. A., Ellis, H. I., and Whittow, G. C. (1988). Incubation energetics of the Laysan albatross. Oecologia 74, 546–550. doi: 10.1007/BF00380052
Phalan, B., Phillips, R. A., Silk, J. R. D., Afanasyev, V., Fukuda, A., Fox, J., et al. (2007). Foraging behaviour of four albatross species by night and day. Mar. Ecol. Prog. Ser. 340, 271–286. doi: 10.3354/meps340271
Phillips, R. A., Xavier, J. C., and Croxall, J. P. (2003). Effects of satellite transmitters on albatrosses and petrels. Auk 120, 1082–1090. doi: 10.1642/0004-8038(2003)120[1082:EOSTOA]2.0.CO;2
Pinaud, D., Cherel, Y., and Weimerskirch, H. (2005). Effect of environmental variability on habitat selection, diet, provisioning behaviour and chick growth in yellow-nosed albatrosses. Mar. Ecol. Prog. Ser. 298, 295–304. doi: 10.3354/meps298295
Pinaud, D., and Weimerskirch, H. (2005). Scale-dependent habitat use in a long-ranging central place predator. J. Anim. Ecol. 74, 852–863. doi: 10.1111/j.1365-2656.2005.00984.x
Polovina, J. J., Howell, E., Kobayashi, D. R., and Seki, M. P. (2001). The transition zone chlorophyll front, a dynamic global feature defining migration and forage habitat for marine resources. Prog. Oceanogr. 49, 469–483. doi: 10.1016/S0079-6611(01)00036-2
Pyke, G. H. (1984). Optimal foraging theory: a critical review. Annu. Rev. Ecol. Syst. 15, 523–575. doi: 10.1146/annurev.es.15.110184.002515
R Development Core Team (2010). R: A Language and Environment for Statistical Computing. Vienna: R Foundation for Statistical Computing.
Read, J. F., Lucas, M. I., Holley, S. E., and Pollard, R. T. (2000). Phytoplankton, nutrients and hydrography in the frontal zone between the Southwest Indian Subtropical gyre and the Southern Ocean. Deep Sea Res. I 47, 2341–2368. doi: 10.1016/S0967-0637(00)00021-2
Reilly, J. J., and Fedak, M. A. (1990). Measurement of the body composition of living gray seals by hydrogen isotope dilution. J. Appl. Physiol. 69, 885–891.
Ricklefs, R. E. (1983). Some considerations on the reproductive energetics of pelagic seabirds. Stud. Avian Biol. 8, 84–94.
Russell, R. W., Hunt, G. L., Coyle, K. O., and Cooney, R. T. (1992). Foraging in a fractal environment: spatial patterns in a marine predator-prey system. Landsc. Ecol. 7, 195–209. doi: 10.1007/BF00133310
Schneider, D. C. (1990). Seabirds and fronts: a brief overview. Polar Res. 8, 17–21. doi: 10.3402/polar.v8i1.6798
Shaffer, S. A. (2011). A review of seabird energetics using the doubly labeled water method. Comp. Biochem. Physiol. A 158, 315–322. doi: 10.1016/j.cbpa.2010.07.012
Shaffer, S. A., Costa, D. P., and Weimerskirch, H. (2001a). Behavioural factors affecting foraging effort of breeding wandering albatrosses. J. Anim. Ecol. 70, 864–874. doi: 10.1046/j.0021-8790.2001.00548.x
Shaffer, S. A., Costa, D. P., and Weimerskirch, H. (2001b). Comparison of methods for evaluating energy expenditure of incubating wandering albatrosses. Physiol. Biochem. Zool. 74, 823–831. doi: 10.1086/323650
Shaffer, S. A., Costa, D. P., and Weimerskirch, H. (2003). Foraging effort in relation to the constraints of reproduction in free-ranging albatrosses. Funct. Ecol. 17, 66–74. doi: 10.1046/j.1365-2435.2003.00705.x
Shaffer, S. A., Costa, D. P., and Weimerskirch, H. (2004). Field metabolic rates of black-browed albatrosses Thalassarche melanophrys during the incubation stage. J. Avian Biol. 35, 551–558. doi: 10.1111/j.0908-8857.2004.03264.x
Shaffer, S. A., Gabrielsen, G. W., Verreault, J., and Costa, D. P. (2006). Validation of water flux and body composition in glaucous gulls ( Larus hyperboreus ). Physiol. Biochem. Zool. 79, 836–845. doi: 10.1086/504611
Sibly, R. M., Brown, J. H., and Kodric-Brown, A. (2012). Metabolic Ecology: A Scaling Approach. Oxford: John Wiley & Sons, Ltd.
Speakman, J. R. (1997). Doubly Labelled Water: Theory and Practice. London: Chapman and Hall.
Spear, L. B., and Ainley, D. G. (1997). Flight speed of seabirds in relation to wind speed and direction. Ibis 139, 234–251. doi: 10.1111/j.1474-919X.1997.tb04621.x
Suryan, R. M., Anderson, D. J., Shaffer, S. A., Roby, D. D., Tremblay, Y., Costa, D. P., et al. (2008). Wind, waves, and wing loading: morphological specialization may limit range expansion of endangered albatrosses. PLoS ONE 3:e4016. doi: 10.1371/journal.pone.0004016
Suryan, R. M., Sato, F., Balogh, G. R., Hyrenbach, K. D., Sievert, P. R., and Ozaki, K. (2006). Foraging destinations and marine habitat use of short-tailed albatrosses: a multi-scale approach using first-passage time analysis. Deep-Sea Res. II 53, 370–386. doi: 10.1016/j.dsr2.2006.01.012
Tickell, W. L. N. (1968). The biology of the great albatrosses, Diomedea exulans and Diomedea epomophora . Antarct. Res. Ser. 12, 1–55.
Tickell, W. L. N. (2000). Albatrosses. New Haven, CT: Yale University Press.
Weimerskirch, H. (2007). Are seabirds foraging for unpredictable resources? Deep-Sea Res. II 54, 211–223. doi: 10.1016/j.dsr2.2006.11.013
Weimerskirch, H., and Cherel, Y. (1998). Feeding ecology of short-tailed shearwaters: breeding in Tasmania and foraging in the Antarctic? Mar. Ecol. Prog. Ser. 167, 261–274. doi: 10.3354/meps167261
Weimerskirch, H., Cherel, Y., Cuenot-Chaillet, F., and Ridoux, V. (1997). Alternative foraging strategies and resource allocation by male and female wandering albatrosses. Ecology 78, 2051–2063. doi: 10.1890/0012-9658(1997)078[2051:AFSARA]2.0.CO;2
Weimerskirch, H., Gault, A., and Cherel, Y. (2005). Prey distribution and patchiness: factors in foraging success and efficiency of wandering albatrosses. Ecology 86, 2611–2622. doi: 10.1890/04-1866
Weimerskirch, H., and Guionnet, T. (2002). Comparative activity pattern during foraging of four albatross species. Ibis 144, 40–50. doi: 10.1046/j.0019-1019.2001.00021.x
Weimerskirch, H., Guionnet, T., Martin, J., Shaffer, S. A., and Costa, D. P. (2000). Fast and fuel efficient? Optimal use of wind by flying albatrosses. Proc. R. Soc. Lond. B 267, 1869–1874. doi: 10.1098/rspb.2000.1223
Weimerskirch, H., and Lys, P. (2000). Seasonal changes in the provisioning behaviour and mass of male and female wandering albatrosses in relation to the growth of their chick. Polar Biol. 23, 733–744. doi: 10.1007/s003000000144
Weimerskirch, H., Salamolard, M., Sarrazin, F., and Jouventin, P. (1993). Foraging strategy of wandering albatrosses through the breeding season: a study using satellite telemetry. Auk 110, 325–342.
Welcker, J., Harding, A. M. A., Kitaysky, A. S., Speakman, J. R., and Gabrielsen, G. W. (2009). Daily energy expenditure increases in response to low nutritional stress in an Arctic-breeding seabird with no effect on mortality. Funct. Ecol. 23, 1081–1090. doi: 10.1111/j.1365-2435.2009.01585.x
Wilson, R. P., Weimerskirch, H., and Lys, P. (1995). A device for measuring seabird activity at sea. J. Avian Biol. 26, 172–175. doi: 10.2307/3677067
Yatsu, A., Shimada, H., and Murata, M. (1993). Distributions of epipelagic fishes, squids, marine mammals, seabirds and sea turtles in the Central North Pacific. Int. N. Pac. Fish Comm. Bull. 53, 111–146.
Keywords: Laysan albatross, black-footed albatross, Indian yellow-nosed albatross, foraging behavior, activity patterns, satellite tracking, doubly labeled water, energetics
Citation: Antolos M, Shaffer SA, Weimerskirch H, Tremblay Y and Costa DP (2017) Foraging Behavior and Energetics of Albatrosses in Contrasting Breeding Environments. Front. Mar. Sci . 4:414. doi: 10.3389/fmars.2017.00414
Received: 19 September 2017; Accepted: 01 December 2017; Published: 15 December 2017.
Reviewed by:
Copyright © 2017 Antolos, Shaffer, Weimerskirch, Tremblay and Costa. This is an open-access article distributed under the terms of the Creative Commons Attribution License (CC BY) . The use, distribution or reproduction in other forums is permitted, provided the original author(s) or licensor are credited and that the original publication in this journal is cited, in accordance with accepted academic practice. No use, distribution or reproduction is permitted which does not comply with these terms.
*Correspondence: Michelle Antolos, [email protected]
† Present Address: Michelle Antolos, Department of Fisheries and Wildlife, Oregon State University, Corvallis, OR, United States Scott A. Shaffer, Department of Biological Sciences, San Jose State University, San Jose, CA, United States Yann Tremblay, UMR MARBEC, IRD - Ifremer - Univ. Montpellier - CNRS, Sète, France
share this!
January 23, 2024
This article has been reviewed according to Science X's editorial process and policies . Editors have highlighted the following attributes while ensuring the content's credibility:
fact-checked
trusted source
written by researcher(s)
Albatrosses are threatened with extinction, and climate change could put nesting sites at risk
by Mia Momberg, The Conversation
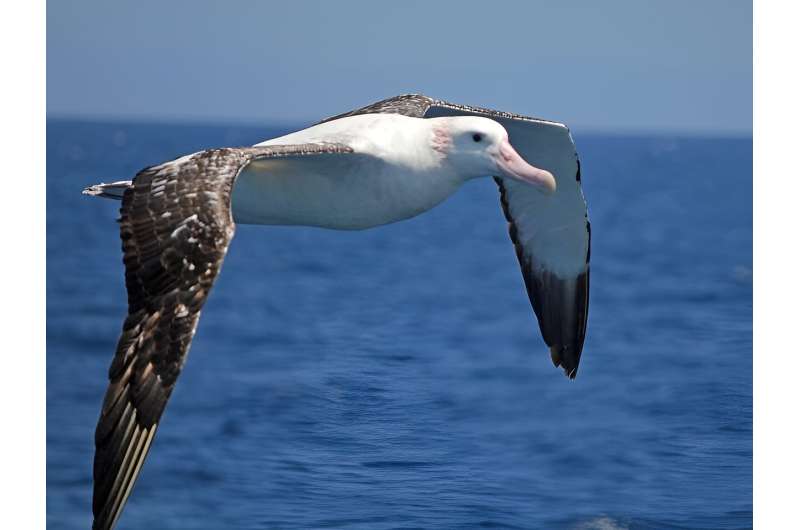
The wandering albatross (Diomedea exulans) is the world's largest flying bird , with a wingspan reaching an incredible 3.5 meters. These birds are oceanic nomads: they spend most of their 60 years of life at sea and only come to land to breed approximately every two years once they have reached sexual maturity.
Their playground is the vast Southern Ocean —the region between the latitude of 60 degrees south and the continent of Antarctica—and the scattered islands within this ocean where they make their nests.
Marion Island and Prince Edward Island , about 2,300km south of South Africa, are some of the only land masses for thousands of kilometers in the Southern Ocean.
Together, these two islands support about half of the entire world's wandering albatross breeding population, estimated at around 20,000 mature individuals . Every year scientists from South African universities survey Marion Island to locate and record each wandering albatross nest.
The species, listed as vulnerable by the International Union for Conservation of Nature , faces huge risks while in the open ocean, in particular due to bycatch from longline fishing trawlers. This makes it important to understand their breeding ecology to ensure that the population remains stable.
I was part of a study during 2021 to investigate which environmental variables affect the birds' choice of nest site on Marion Island. The birds make their nests—a mound of soil and vegetation—on the ground. We looked at wind characteristics, vegetation and geological characteristics at nest locations from three breeding seasons.
Elevation turned out to be the most important variable—the albatrosses preferred a low (warmer) site and coastal vegetation. But these preferences also point to dangers for the birds from climate change. The greatest risk to the availability of nesting sites will be a much smaller suitable nesting range in future than at present. This could be devastating to the population.
Variables influencing nest site selection
Marion Island is of volcanic origin and has a rough terrain . Some areas are covered in sharp rock and others are boggy, with very wet vegetation. There is rain and strong wind on most days. Conducting research here requires walking long distances in all weathers—but the island is ideal for studying climate change , because the Southern Ocean is experiencing some of the largest global changes in climate and it is relatively undisturbed by humans.
Using GPS coordinate nest data from the entire breeding population on Marion Island, we aimed to determine which factors affected where the birds breed. With more than 1,900 nests, and 10,000 randomly generated points where nests are not present, we extracted:
- elevation (which on this island is also a proxy for temperature)
- terrain ruggedness
- distance to the coast
- vegetation type
- wind turbulence
- underlying geology.
The variables were ranked according to their influence on the statistical model predicting the likelihood of a nest being present under the conditions found at a certain point.
The most important variable was elevation. The majority of the nests were found close to the coast, where the elevation is lower. These areas are warmer, which means that the chicks would be less exposed to very cold temperatures on their open nests.
The probability of nests being present also declined with distance from the coast, probably because there are more suitable habitats closer to the coast.
Vegetation type was strongly determined by elevation and distance from the coast. This was an important factor, as the birds use vegetation to build their nests. In addition, dead vegetation contributes to the soil formation on the island, which is also used in nest construction.
The probability of encountering nests is lower as the terrain ruggedness increases since these birds need a runway of flat space to use for take-off and landing. During incubation, the adults take turns to remain on the nest. Later they will leave the chick on its own for up to 10 days at a time. They continue to feed the chick for up to 300 days.
Areas with intermediate wind speeds were those most likely to have a nest. At least some wind is needed for flight, but too much wind may cause chicks to blow off the nests or become too cold.
Delicate balance
Changing climates may upset this delicate balance. Human-driven changes will have impacts on temperature, rainfall and wind speeds, which in turn affect vegetation and other species distribution patterns .
By 2003, Marion Island's temperature had increased by 1.2°C compared to 50 years before. Precipitation had decreased by 25% and cloud cover also decreased, leading to an increase in sunshine hours . The permanent snowline which was present in the 1950s no longer exists . These changes have continued in the 20 years since their initial documentation, and are likely to continue.
Strong vegetation shifts were already documented in the sub-Antarctic years ago. Over 40 years, many species have shifted their ranges to higher elevations where the temperatures remain cooler. Wind speeds have also already increased in the Southern Ocean and are predicted to continue doing so, which may have effects on the size of areas suitable for nesting.
If nesting sites move to higher elevations on Marion Island as temperatures warm, and some areas become unsuitable due to changes in vegetation or wind speeds, it is likely that the suitable nesting area on the island will shrink considerably.
Our study adds to what is known about the elements affecting nest-site selection in birds. Notably, we add knowledge of wind , an underexplored element, influencing nest -site selection in a large oceanic bird. The results could also provide insights that apply to other surface-nesting seabirds.
Provided by The Conversation
Explore further
Feedback to editors

Accelerating the discovery of new materials via the ion-exchange method
2 minutes ago

Weather prediction models can also forecast satellite displacements
4 minutes ago

Researchers set new standards for nanoparticles, helping patients with MS, ALS, Parkinson's disease
33 minutes ago

Global study finds there really are more insects out after dark
51 minutes ago

Scientists trigger mini-earthquakes in the lab

Seeing is believing: Scientists reveal connectome of the fruit fly visual system

Why zebrafish can regenerate damaged heart tissue, while other fish species cannot

Development of organic semiconductors featuring ultrafast electrons
2 hours ago

Uncovering key players in gene silencing: Insights into plant growth and human diseases
3 hours ago

Many prisoners go years without touching a smartphone—it means they struggle to navigate life on the outside
Relevant physicsforums posts, if theres a 15% probability each month of getting a woman pregnant..., can four legged animals drink from beneath their feet.
Apr 15, 2024
Mold in Plastic Water Bottles? What does it eat?
Apr 14, 2024
Dolphins don't breathe through their esophagus
Is this egg-laying or something else.
Apr 13, 2024
Color Recognition: What we see vs animals with a larger color range
Apr 12, 2024
More from Biology and Medical
Related Stories

South Africa's Agulhas long-billed lark: Adapting, surviving despite farming taking over their nesting grounds
Jan 23, 2024

Sea level rises could threaten sea turtle breeding grounds
Apr 20, 2023

Seabird nests are full of discarded plastic debris
May 5, 2020

First US study of nest temperature impacts on leatherback hatchlings
Sep 7, 2023

Heat waves harm bird reproduction on agricultural lands, research suggests
Oct 19, 2023

Canberra's superb parrots caught up in housing crisis
Feb 10, 2023
Recommended for you

Mycoheterotrophic plants as a key to the 'Wood Wide Web'

Baby white sharks prefer being closer to shore, scientists find
12 hours ago
Let us know if there is a problem with our content
Use this form if you have come across a typo, inaccuracy or would like to send an edit request for the content on this page. For general inquiries, please use our contact form . For general feedback, use the public comments section below (please adhere to guidelines ).
Please select the most appropriate category to facilitate processing of your request
Thank you for taking time to provide your feedback to the editors.
Your feedback is important to us. However, we do not guarantee individual replies due to the high volume of messages.
E-mail the story
Your email address is used only to let the recipient know who sent the email. Neither your address nor the recipient's address will be used for any other purpose. The information you enter will appear in your e-mail message and is not retained by Phys.org in any form.
Newsletter sign up
Get weekly and/or daily updates delivered to your inbox. You can unsubscribe at any time and we'll never share your details to third parties.
More information Privacy policy
Donate and enjoy an ad-free experience
We keep our content available to everyone. Consider supporting Science X's mission by getting a premium account.
E-mail newsletter
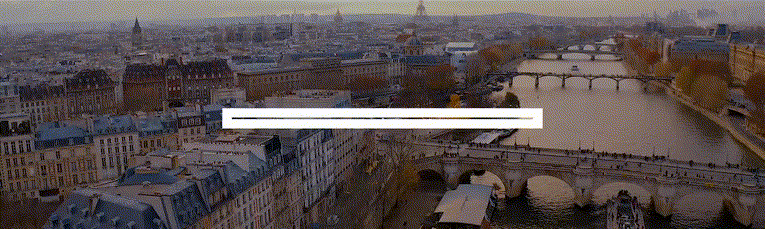
IMAGES
VIDEO
COMMENTS
Length. 107-135. cm inch. Wingspan. 2.5-3.5. m ft. Described as "The bird which made the breeze to blow" the wingspan of a Wandering albatross ( Diomedea exulans) is the longest of any bird. It lives up to its name when it takes fishing trips that last 10-20 days and can cover 10,000 km while using hardly more energy than when sitting on its nest.
The Wandering Albatross is a massive sea bird also known as a Goonie, or White Winged Albatross. Home; ... humans are extremely detrimental to these birds. Sailors have killed birds, both at sea and in nesting colonies, for decades. In fact, humans are the only known predator of adult albatrosses. ... Behavior of the Wandering Albatross. This ...
1. They can travel 120k km (75k) miles in a year. The Wandering albatross might be the most wide-ranging of all foraging sea birds, and maybe of all animals. They've been tracked over 15,000 km in a single foraging trip, capable of speeds of up to 80 kmph and distances of over 900 km per day. 1. 2.
Vulnerable. Known for its majestic wingspan and far-ranging travels, the Wandering Albatross is a captivating presence in the Southern Ocean's expanse. As the bird with the widest wingspan globally, this remarkable creature glides effortlessly across vast oceanic distances, its brilliant white plumage and solitary habits making it a unique ...
The wandering albatross (Diomedea exulans) is the world's largest flying bird, with a wingspan reaching an incredible 3.5 metres.These birds are oceanic nomads: they spend most of their 60 years ...
There is some disagreement over how many subspecies of wandering albatross (Diomedea exulans ... Wandering albatrosses nest in sheltered areas on plateaus, ridges, plains, or valleys. Outside of the breeding season, wandering albatrosses are found only in the open ocean, where food is abundant. ... Behavior. While foraging at sea, wandering ...
The Life Cycle of the Wandering Albatross Breeding and Nesting Patterns. The breeding season for the Wandering Albatross begins in the austral summer months, with courtship rituals that involve intricate displays of dance and vocalizations. These courtship displays are not only a way for the albatrosses to attract a mate but also a means of ...
The average span for a wandering albatross is just over 3 meters (10 feet), with a range between 2.51 and 3.5 meters (8 feet 3 inches-11 feet 6 inches. The largest verified wingspan measurement is 3.7 meters or 12 feet 2 inches. The largest reported wingspan, although unverified, is 5.3 meters (17 feet 5 inches).
On the Southern Ocean's islands where they nest and brood, one wandering albatross parent tends the nest while its partner takes to the sea, traveling as much as 10,000 kilometers as it forages ...
Wandering albatross. (Elizabeth Crapo, NOAA) A wandering albatross has the largest wingspan of any bird, 3.5 meters (11.5 feet) tip to wing tip.
The Wandering Albatross, also known as Diomedea exulans, is a majestic seabird that belongs to the albatross family. ... Nesting on subantarctic islands, foraging in southern ocean waters: Diet: Squid, fish, crustaceans: Lifespan: ... as their territorial disputes are usually settled through displays of courtship or aggressive behavior. These ...
April 1993] Wandering Albatross Foraging Strategy 327 after the last location, the distance covered between the nest and the last location was assumed to be a direct line. Otherwise, only the maximum range was considered for these foraging trips. Transmission fail- ure was caused by broken antennae, exhausted bat-
These remarkably efficient gliders, named after the Greek hero Diomedes, have the largest wingspan of any bird on the planet. Name: Wandering Albatross, Snowy Albatross, White-winged Albatross ( Diomedea exulans) Length: Up to 135 cm. Weight: 6 to 12kg. Location: All oceans except in the North Atlantic.
Size: They measure at around 3 ft 6 in to 4 ft 5 in (1.07-1.35 m). Weight: Adult wandering albatrosses typically weigh between 13 and 28 lbs (5.9-12.7 kg). Color: The plumage for juveniles is chocolate brown which becomes whiter with age. The wings in adults are white with black around the tips while the female's wings have more black on them.
Best Places to see the Wandering Albatross: ... At such nesting sites, it's common to find baby albatross that weigh up to 10kg - heavier sometimes than their parents. Chicks grow to adult birds which are among the largest bird species in the world, weighing between 6 and 12 pounds (2.7 to 5.4 kgs), and growing to be up to 4.4 ft (1.3 metres ...
Studies of wandering albatross have shown over 10% of chicks are extra-pair young; an extremely surprising result since adult wandering albatrosses only breed once every other year when successful. Similar results were seen in black-browed (Thalassarche melanophris) and grey-headed albatrosses (T. chrysostoma) nesting on South Georgia Island.
Wandering Albatross Behavior and Feeding. albatross egg ... Wandering albatrosses defend small nesting territories. The average size of these territories is one square meters. Otherwise the range within which they travel is many thousands of square kilometers. While foraging at sea, wandering albatrosses travel in small groups.
In 2013, 10 wandering albatross chicks (six males and four females), that were about to fledge, were fitted with GPS/Argos satellite transmitters (Platform Terminal Transmitter, PTT 100, Microwave Telemetry, Columbia, USA). These were attached to the central back feathers using adhesive tape (TESA ®) and glue (Loctite ®). GPS tags were ...
The wandering albatross travel enormous distances from their breeding grounds, often going more than 1,000 miles before returning to the nest to relieve their mate from guard duty.
Also known as the wandering albatross, the snowy albatross is the largest of all albatross species. ... Their hunting behavior is opportunistic, taking advantage of both natural prey concentrations and the presence of fishing vessels to scavenge for offal and discarded fish. ... Nesting. Albatrosses typically nest in large colonies on remote ...
Wandering Albatross Behavior. Wandering albatrosses breed on remote islands in the Southern Ocean, and they spend most of their lives at sea, only coming ashore to breed.These birds are highly nomadic, and they often travel thousands of miles in search of food.Wandering albatrosses typically eat squid and fish, but they have also been known to scavenge carrion from ships.
Albatross foraging range is variably constrained during the breeding period, however, due to changing energetic requirements at the nest. During incubation, the fasting capabilities of adults allow breeding pairs to alternate long shifts at the nest (~2-3 weeks) with far-ranging trips to sea.
The wandering albatross (Diomedea exulans) is the world's largest flying bird, with a wingspan reaching an incredible 3.5 meters. ... If nesting sites move to higher elevations on Marion Island as ...
Laysan albatross have a wingspan well over 6 feet and nest on the grassy areas of low, flat islands, according to the National Audubon Society. They have a population of more than 1 million but ...