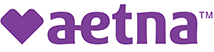
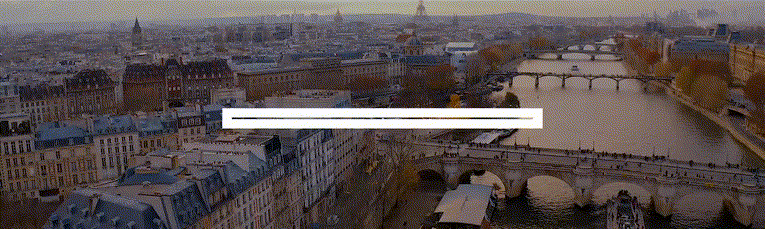
Vaccines for Travel
- Clinical Policy Bulletins
- Medical Clinical Policy Bulletins
Number: 0473
Table Of Contents
Footnotes † Persons who are immunocompromised because of immune deficiency diseases, leukemia, lymphoma, generalized cancer, or the acquired immunodeficiency syndrome, or who are receiving immunosuppressive therapy with corticosteroids, alkylating agents, anti-metabolites, or radiation.
Footnotes †† LYMErix was withdrawn from the U.S. market in February 2002.
Footnotes * Most Aetna benefit plans exclude coverage of vaccines for work. Please check benefit plan descriptions.
Note : The Advisory Committee on Immunization Practices (1996) states that plague vaccination is not indicated for most travelers to countries in which cases of plague have been reported.
Experimental and Investigational
The following vaccines for travel are considered experimental and investigational (not an all-inclusive list):
- Malaria vaccine for travel because an effective malaria vaccine has yet to be developed.
- Oral or skin-patch cholera vaccine for prevention of entero-toxigenic Escherichia coli diarrhea because their clinical value has not been established.
Policy Limitations and Exclusions
Note: Most Aetna HMO plans exclude coverage of vaccines for travel. Most Aetna traditional plans cover medically necessary travel vaccines for members of plans with preventive services benefits. Please check benefit plan descriptions.
Note : Many of these vaccines may also be considered medically necessary for reasons other than travel, and may be covered when medically necessary in members with preventive benefits, regardless of whether the plan excludes coverage of travel vaccines.
The Centers for Disease Control and Prevention (CDC)'s recommended vaccinations for travelers can be found at the following website: Destinations and Travelers Health .
In a Cochrane review on vaccines for preventing malaria, Graves and Gelband (2006a) concluded that there is no evidence for protection by SPf66 vaccines against P. falciparum in Africa. There is a modest reduction in attacks of P. falciparum malaria following vaccination with SPf66 in South America. There is no justification for further trials of SPf66 in its current formulation. Further research with SPf66 vaccines in South America or with new formulations of SPf66 may be justified.
In another Cochrane review, Graves and Gelband (2006b) concluded that the MSP/RESA (Combination B) vaccine shows promise as a way to reduce the severity of malaria episodes, but the effect of the vaccine is MSP2 variant-specific. Pre-treatment for malaria during a vaccine trial makes the results difficult to interpret, particularly with the relatively small sample sizes of early trials. The results show that blood-stage vaccines may play a role and merit further development.
Vaughan et al (2009) presented a comprehensive meta-analysis of more than 500 references, describing nearly 5,000 unique B cell and T cell epitopes derived from the Plasmodium genus, and detailing thousands of immunological assays. This was the first inventory of epitope data related to malaria-specific immunology, plasmodial pathogenesis, and vaccine performance. The survey included host and pathogen species distribution of epitopes, the number of antibody versus CD4(+) and CD8(+) T cell epitopes, the genomic distribution of recognized epitopes, variance among epitopes from different parasite strains, and the characterization of protective epitopes and of epitopes associated with parasite evasion of the host immune response. The results identified knowledge gaps and areas for further investigation. This information has relevance to issues, such as the identification of epitopes and antigens associated with protective immunity, the design and development of candidate malaria vaccines, and characterization of immune response to strain polymorphisms.
Currently, there is an ongoing phase III clinical trial of a candidate vaccine for malaria, but the study has not been completed (Birkett, 2010).
The Advisory Committee on Immunization Practices (ACIP) of the CDC provided the following recommendations regarding the prevention of plague (1996):
- Routine plague vaccination is not necessary for individuals living in areas in which plague is enzootic.
- Plague vaccination is not indicated for hospital staff or other medical personnel in such areas.
- Plague vaccination is not indicated for most travelers to countries in which cases of plague have been reported.
In a Cochrane review, Ahmed et al (2013) evaluated the safety, effectiveness, and immunogenicity of vaccines for preventing entero-toxigenic Escherichia coli (ETEC) diarrhea. These investigators searched the Cochrane Infectious Disease Group Specialized Register, the Cochrane Central Register of Controlled Trials (CENTRAL), MEDLINE, EMBASE, LILACS, and ClinicalTrials up to December 2012. Randomized controlled trials (RCTs) and quasi-RCTs comparing use of vaccines to prevent ETEC with use of no intervention, a control vaccine (either an inert vaccine or a vaccine normally given to prevent an unrelated infection), an alternative ETEC vaccine, or a different dose or schedule of the same ETEC vaccine in healthy adults and children living in endemic regions, intending to travel to endemic regions, or volunteering to receive an artificial challenge of ETEC bacteria were included for analysis. Two authors independently assessed each trial for eligibility and risk of bias. Two independent reviewers extracted data from the included studies and analyzed the data using Review Manager (RevMan) software. They reported outcomes as risk ratios (RR) with 95 % confidence intervals (CI) and assessed the quality of the evidence using the GRADE approach. A total of 24 RCTs, including 53,247 participants, met the inclusion criteria – 4 studies assessed the protective efficacy of oral cholera vaccines when used to prevent diarrhea due to ETEC and 7 studies assessed the protective efficacy of ETEC-specific vaccines. Of these 11 studies, 7 studies presented efficacy data from field trials and 4 studies presented efficacy data from artificial challenge studies. An additional 13 trials contributed safety and immunological data only. The currently available, oral cholera killed whole cell vaccine (Dukoral®) was evaluated for protection of people against "travelers' diarrhea" in a single RCT in people arriving in Mexico from the USA. These researchers did not identify any statistically significant effects on ETEC diarrhea or all-cause diarrhea (1 trial, 502 participants; low-quality evidence). Two earlier trials, one undertaken in an endemic population in Bangladesh and one undertaken in people travelling from Finland to Morocco, evaluated a precursor of this vaccine containing purified cholera toxin B subunit rather than the recombinant subunit in Dukoral®. Short-term protective efficacy against ETEC diarrhea was demonstrated, lasting for around 3 months (RR 0.43, 95 % CI: 0.26 to 0.71; 2 trials, 50,227 participants). This vaccine is no longer available. An ETEC-specific, killed whole cell vaccine, which also contains the recombinant cholera toxin B-subunit, was evaluated in people traveling from the USA to Mexico or Guatemala, and from Austria to Latin America, Africa, or Asia. These investigators did not identify any statistically significant differences in ETEC-specific diarrhea or all-cause diarrhea (2 trials, 799 participants), and the vaccine was associated with increased vomiting (RR 2.0, 95 % CI: 1.16 to 3.45; 9 trials, 1,528 participants). The other ETEC-specific vaccines in development have not yet demonstrated clinically important benefits. The authors concluded that there is currently insufficient evidence from RCTs to support the use of the oral cholera vaccine Dukoral® for protecting travelers against ETEC diarrhea. Moreover, they stated that further research is needed to develop safe and effective vaccines to provide both short- and long-term protection against ETEC diarrhea.
Also, an UpToDate review on "Travelers' diarrhea" (Wanke, 2014) states that "Use of vaccines to protect against travelers’ diarrhea is hindered by the varied pathogens that can cause it. Although enterotoxigenic E. coli (ETEC) predominates as an etiology of travelers’ diarrhea, vaccination strategies that have focused on this pathogen as a target have been suboptimal. Although vaccination to protect against cholera is not routinely recommended for travelers, a number of trials suggest that the oral, killed whole-cell vaccine given with the nontoxic B subunit of cholera toxin (Dukoral) provides protection for travelers against ETEC infection. The rationale for such protection is that the B subunit of cholera is antigenically similar to the heat-labile enterotoxin of ETEC. In two randomized trials, the killed whole-cell vaccine combined with the B subunit of cholera toxin reduced the incidence of diarrhea caused by ETEC by 67 percent in a trial in Bangladesh and 52 percent among travelers to Morocco. The Dukoral vaccine was approved in the United States in late 2006 for use as a travelers' diarrhea vaccine. However, a conservative estimate that took into account the incidence of ETEC infection throughout the world and the efficacy of the vaccine suggested that it may prevent ≤7 percent of travelers' diarrhea cases. The 2006 guidelines on travel medicine from the Infectious Diseases Society of America concluded that the decision to use the vaccine to prevent travelers' diarrhea must balance its cost, adverse effects, and limited utility against the known effectiveness and costs of self-treatment as described above. A separate vaccination strategy for ETEC also appears to have limited utility. Despite initial promising data on vaccination with heat-labile enterotoxin from ETEC via a skin patch, it was not effective in decreasing the incidence of moderate to severe diarrhea due to either ETEC or any cause in a randomized, placebo controlled trial that included 1,644 individuals who traveled to Mexico or Guatemala. In a subgroup analysis, the vaccine provided modest protection against ETEC that produced only heat-labile enterotoxin (vaccine efficacy 61 percent [95 % CI, 7 to 84 percent]), but not ETEC that produced heat-stable toxin or both. This highlights the limitations of a single-antigen vaccine for travelers’ diarrhea".
An UpToDate review on "Immunizations for travel" (Freedman and Leder, 2015) states that "In general, severely immunocompromised patients should not receive live vaccines. Live vaccines include yellow fever vaccine, oral typhoid vaccine, nasal influenza vaccine, oral polio vaccine (OPV), MMR, and varicella vaccine. Inactivated vaccines include meningococcal vaccine, parenteral typhoid vaccine, hepatitis A and B vaccines, rabies vaccine, Japanese encephalitis vaccine, inactivated influenza vaccine, inactivated polio vaccine (IPV), Tdap, and Td".
Chikungunya Vaccine
Chikungunya is primarily a mosquito-borne alphavirus caused by the chikungunya virus (CHIKV) that is often associated with fever and debilitating joint pain. Rarely, the virus can be transmitted via blood products, laboratory and maternal-fetal transmission. Outbreaks typically occur in tropical and subtropical regions of Africa, Asia, Oceania, and parts of the Americas and Europe where chikungunya virus-carrying mosquitos are endemic (Bettis et al, 2022; Wilson and Lenschow, 2022). Between 2014 and 2016, 3,941 cases were reported in the United States among travelers; 92% were associated with travel in the Americas (most commonly the Dominican Republic, Puerto Rico, and Haiti). The remaining 8% had traveled to Asia, Africa, or the Western Pacific (Lindsey et al, 2018; Wilson and Lenschow, 2022).
Management of the chikungunya virus is supportive (i.e., rest, fluids, antiinflammatory and analgesic agents). Systemic glucocorticoids or treatment with a disease-modifying antirheumatic drug (DMARD) has been used in refractory or chronic arthritis cases. The cornerstone of prevention has been minimizing mosquito exposure (Lenschow and Wilson, 2023). In November 2023, the FDA approved the first chikungunya vaccine (Ixchiq, Valneva Scotland Ltd) for the prevention of disease caused by chikungunya virus (CHIKV) in individuals 18 years of age and older who are at increased risk of exposure to CHIKV. "This indication is approved under accelerated approval based on anti-CHIKV neutralizing antibody titers. Continued approval for this indication may be contingent upon verification and description of clinical benefit in confirmatory studies" (Valneva, 2023).
FDA approval was based on two clinical trials conducted in North America that evaluated the safety of Ixchiq in adults 18 years or older. In clinical studies, the most common solicited injection site reaction (greater than 10%) was tenderness (10.6%). The most common solicited systemic adverse reactions (greater than 10%) were headache (31.6%), fatigue (28.5%), myalgia (23.9%), arthralgia (17.2%), fever (13.5%) and nausea (11.2%). The effectiveness was based on immune response data from a clinical study conducted in the US in adults. "In this study, the immune response of 266 participants who received the vaccine was compared to the immune response of 96 participants who received placebo. The level of antibody evaluated in study participants was based on a level shown to be protective in non-human primates that had received blood from people who had been vaccinated. Almost all vaccine study participants achieved this antibody level" (FDA, 2023).
Ixchiq is administered as a single intramuscular injection. Ixchiq contains a live, weakened version of the chikungunya virus and may cause symptoms in the vaccine recipient similar to those experienced by people who have chikungunya disease. It is contraindicated in immunocompromised individuals or those with a history of a severe allergic reaction to any component of Ixchiq. Vertical transmission of wild-type CHIKV from pregnant individuals with viremia at delivery is common and can cause potentially fatal CHIKV disease in neonates. Vaccine viremia occurs in the first week following administration of Ixchiq, with resolution of viremia by 14 days after vaccination. It is not known if the vaccine virus can be vertically transmitted and cause fetal or neonatal adverse reactions. A decision to administer during pregnancy should take into consideration the individual’s risk of wild-type CHIKV infection, gestational age, and risks to the fetus or neonate from vertical transmission of wild-type CHIKV. Labeled warnings and precautions also include risk of syncope associated with administration of injectable vaccines (Valneva, 2023).
The Centers for Disease Control's (CDC) Advisory Committee on Immunization Practices (ACIP) met in October 2023 to discuss recommendations for the chikungunya vaccine. Draft recommendations include chikungunya vaccine recommendation for persons 18 years of age and older traveling to a country or territory where there is a chikungunya outbreak. In addition, the vaccine may be considered for persons traveling to a country or territory without an outbreak but with evidence of chikungunya virus transmission among humans within the last 5 years:
- Older persons (e.g., >65 years), particularly those with underlying medical conditions, who are likely to have at least moderate exposure (moderate exposure could include travelers who might have at least 2 weeks (cumulative) of exposure to mosquitoes in indoor and/or outdoor settings) to mosquitoes
- Persons staying for a cumulative period of 6 months or more during a 2-year period
Japanese Encephalitis Vaccine for Pediatric Travelers
Taucher and colleagues (2020) stated that in an initial study among children from non-Japanese encephalitis (JE)-endemic countries, sero-protection rates (SPRs) remained high 6 months following completion of the primary series with IXIARO. In an open-label, follow-up study, a subset of 23 children who received a 2-dose primary series of IXIARO in the parent study, were examined for safety and neutralizing antibody persistence for 36 months. SPRs remained high but declined from 100 % 1 month after primary immunization to 91.3 % at month 7 and 89.5 % at month 36. Geometric mean titers (GMTs) declined considerably from 384.1 by day 56-60.8 at month 36. No long-term safety concerns were identified. The authors concluded that the substantial decline in GMT observed in this study, together with previously published data on children vaccinated with IXIARO supported the recommendation for a booster dose in children who remain at risk of JE from 1 year after the primary series of IXIARO, consistent with the recommendation for adults.
Jost and colleagues (2015) evaluated the relevance of travel-related measles, a highly transmissible and vaccine-preventable disease. Between 2001 and 2013, surveillance and travel-related measles data were systematically reviewed according to the PRISMA guidelines with extraction of relevant articles from Medline, Embase, GoogleScholar and from public health authorities in the Region of the Americas, Europe and Australia. From a total of 960 records, 44 articles were included and they comprised 2,128 imported measles cases between 2001 and 2011. The proportion of imported cases in Europe was low at 1 to 2 %, which reflected the situation in a measles-endemic region. In contrast, imported and import-related measles accounted for up to 100 % of all cases in regions with interrupted endemic measles transmission; 11 air-travel related reports described 132 measles index cases leading to 47 secondary cases. Secondary transmission was significantly more likely to occur if the index case was younger or when there were multiple infectious cases on board. Further spread to health care settings was found. Measles cases associated with cruise ship travel or mass gatherings were sporadically observed. The authors concluded that within both, endemic and non-endemic home countries, pre-travel health advice should assess MMR immunity routinely to avoid measles spread by non-immune travelers. They stated that to identify measles spread as well as to increase and sustain high vaccination coverages, joint efforts of public health specialists, health care practitioners and travel medicine providers are needed.
An UpToDate review on "Immunizations for travel" (Freedman and Leder, 2016) states that "Children traveling outside the United States should receive MMR vaccination sooner than the standard immunization schedule. Prior to departure, children 12 months of age or older should have received 2 doses of MMR vaccine separated by at least 28 days, with the first dose administered on or after the first birthday. Children aged 6 to 11 months should receive 1 dose of MMR before departure. MMR vaccination for adults is indicated for individuals born in 1957 or later in the United States (before 1970 in Canada; before 1966 in Australia) without evidence of immunity or without evidence of 2 doses of an adequate live vaccine at any time after age 12 months. Although individuals born before 1957 in the United States are presumed to be immune (exceptions include United States healthcare workers and women of childbearing age), 2 doses of MMR vaccine spaced by 1 month should be strongly considered for unvaccinated individuals without other evidence of immunity who were born before 1957 (in the United States) and are traveling for purposes of healthcare or humanitarian work potentially entailing close contact with ill individuals. MMR vaccination is contraindicated in pregnant and immunocompromised patients".
The CDC (2015) states that anyone who is not protected against measles is at risk of getting infected when they travel internationally. It recommends the following: Centers for Disease Control and Prevention .
- Infants 6 months through 11 months of age should receive 1 dose of MMR vaccine Footnotes for MMR vaccines for infants †
- Children 12 months of age and older should receive 2 doses of MMR vaccine separated by at least 28 days.
- Teenagers and adults who do not have evidence of immunity Footnotes for Acceptable presumptive evidence of immunity against measles * against measles should get 2 doses of MMR vaccine separated by at least 28 days.
Footnotes † Infants who get 1 dose of MMR vaccine before their first birthday should get 2 more doses (1 dose at 12 through 15 months of age and another dose at least 28 days later).
Footnotes * Acceptable presumptive evidence of immunity against measles includes at least one of the following: written documentation of adequate vaccination, laboratory evidence of immunity, laboratory confirmation of measles, or birth in the United States before 1957.
Contraindications and Precautions: Vaccine Recommendations and Guidelines of the ACIP .
Contraindications
- Severe allergic reaction (e.g., anaphylaxis) after a previous dose or to a vaccine component
- Known severe immunodeficiency (e.g., from hematologic and solid tumors, receipt of chemotherapy, congenital immunodeficiency, or long-term immunosuppressive therapy Footnotes for deferred vaccine † or patients with human immunodeficiency virus [HIV] infection who are severely immunocompromised) Footnotes for HIV infected children *
Footnotes † Vaccine should be deferred for the appropriate interval if replacement immune globulin products are being administered.
Footnotes * HIV-infected children may receive varicella and measles vaccine if CD4+ T-lymphocyte count is greater than 15 %.
Precautions
- Moderate or severe acute illness with or without fever
- Recent (within 11 months) receipt of antibody-containing blood product (specific interval depends on product) Footnotes for immune globulin products †
- History of thrombocytopenia or thrombocytopenic purpura
- Need for tuberculin skin testing Footnotes for Measles vaccination *
Footnotes † Vaccine should be deferred for the appropriate interval if replacement immune globulin products are being administered
Footnotes * Measles vaccination might suppress tuberculin reactivity temporarily. Measles-containing vaccine can be administered on the same day as tuberculin skin testing. If testing cannot be performed until after the day of MMR vaccination, the test should be postponed for at least 4 weeks after the vaccination. If an urgent need exists to skin test, do so with the understanding that reactivity might be reduced by the vaccine.
Tick-Borne Encephalitis Vaccine
Rampa et al (2020) state that tick-borne encephalitis (TBE) is increasing in Europe and has become one of the most important causes of viral encephalitis, as well as the most frequent cause of viral meningitis, in Europe. The authors note that there is no antiviral treatment against TBE and that active vaccination is a practical preventive measure to reduce the number of cases. There are two inactivated virus vaccines licensed in Europe: FSME-Immun® (Pfizer), in some countries distributed as Ticovac®, and Encepur® (Bavarian Nordic). FSME-Immun is based on the TBE virus strain Neudoerfl (Nd), whereas Encepur is based on the TBE virus strain Karlsruhe-23 (K23). Both vaccines have a pediatric TBE vaccine variant. Thus, the authors conducted a systematic review (registered at PROSPERO (#CRD42020155737) and conducted in accordance with PRISMA guidelines) of the immunogenicity and safety of the tick-borne encephalitis vaccine (2009-2019). Of a total of 2464 records, 49 original research publications included evaluation for immunogenicity and safety. The authors found that TBE-vaccines showed adequate immunogenicity, good safety and interchangeability in adults and children with some differences in long-term protection (seropositivity in 90.6–100% after primary vaccination; 84.9%–99.4% at 5 year follow up). Primary conventional vaccination schedule (days 0, 28, and 300) demonstrated the best immunogenic results (99–100% of seropositivity). Mixed brand primary vaccination presented adequate safety and immunogenicity with some exceptions. After booster follow-ups, accelerated conventional and rapid vaccination schedules were shown to be comparable in terms of immunogenicity and safety. First booster vaccinations five years after primary vaccination were protective in adults aged less than 50 years, leading to protective antibody levels from at least 5 years up to 10 years after booster vaccination. In older vaccinees, 50 years and older, lower protective antibody titers were found. Allergic individuals showed an adequate response and immunosuppressed individuals a diminished response to TBE-vaccination. The authors concluded that the TBE-vaccination with Encepur or FSME-Immun to be highly immunogenic, well tolerated and in all studies except one to be interchangeable. Schedules should, if possible, use the same vaccine brand (non-mixed). TBE-vaccines are immunogenic in terms of antibody response but less so when vaccination is started after the age of 50 years. Age at priming is a key factor in the duration of protection. In terms of safety, the European, licensed vaccines were found to be well tolerated in both children (aged 1–17 years) and in adults, with local injection site reactions in 24.8% (4.3–54%) and systematic reactions in 30% (0.6–45.9%) of vaccinees. Vaccine related serious adverse events (SAE) were rare.
In February 2021, the U.S. FDA accepted for Priority Review Pfizer’s Biologics License Application (BLA) for TicoVac, its tick-borne encephalitis (TBE) vaccine for active immunization to prevent TBE in individuals 1 year of age and older. If approved, TicoVac would be the first vaccine in the U.S. to help protect adults and children who are visiting or living in TBE endemic areas. In line with Priority Review designation, the FDA will target an action within six months of the application submission date, with the anticipated Prescription Drug User Fee Act (PDUFA) action date expected for August 2021.
The BLA is based on results from "more than 40 years of experience and evidence outside the U.S. In clinical trials, the safety and immunogenicity of TicoVac was assessed across two age groups (1-15 years of age and 16-65 years of age). In these studies, pooled seropositivity rates were 99-100% in 1-15 year olds and 94-99% in adults >15 years following three doses. Clinical studies demonstrated that TicoVac was well-tolerated with no unexpected adverse events or vaccine-related serious adverse events observed. Subsequent real-world studies have shown that the vaccine is 96-99% effective in people who have received at least two doses of the vaccine, and two to three doses of the vaccine were shown to be sufficient to provide a long-lasting immune memory” (Pfizer, 2021b).
On August 13, 2021, the U.S. FDA approved Ticovac (Pfizer Inc.), tick-borne encephalitis (TBE) vaccine, for active immunization to prevent TBE in individuals 1 year of age and older. FDA approval was based on the safety and immunogenicity of Ticovac that were assessed cross two age groups (Study 209: 1 to15 years of age and Studies 213 and 690601: persons 16 years of age and older). In these studies, seropositivity rates were 99.5% in the group of 1 to 15 year olds and 98.7-100% in persons older than 15 years following three primary doses. Clinical studies demonstrated that Ticovac was generally well-tolerated with no unexpected adverse events or vaccine-related serious adverse events observed. The most common adverse reactions across both age groups were local tenderness, headache, local pain, fever, restlessness, fatigue, and muscle pain. Real-world studies from Austria have shown that the vaccine is 96-98.7% effective in people who have received at least three doses of the vaccine (Pfizer, 2021a, 2021c).
Two open-label, multi-center, follow-up studies which enrolled subjects who were seropositive 1 month after the third vaccination from Studies 213 (N=252, ages 16 through 65 at the time of first TICOVAC dose) and 209 (N=358, ages 1 through 15 at the time of first Ticovac dose) were conducted to assess the seropersistence of TBE antibodies after completion of the primary vaccination series and the antibody response to a booster administration. Three years after the primary series of Ticovac , neutralization test (NT) seropositivity in follow-up studies 223 and 700401 ranged from 82.9% to 100% depending on age. Following a booster dose the NT seropositivity rates were 100% (Pfizer, 2021a).
Vaccines for Pregnant Travelers
Nasser and colleagues (2020) noted that pregnant travelers and their offspring are vulnerable to severe outcomes following a wide range of infections. Vaccine-preventable diseases can have a particularly severe course in pregnant women, but little is known about the safety of travel vaccines in pregnant women. These investigators carried out a systematic review of all published literature concerning the safety of vaccines frequently given to travelers such as yellow fever, MMR (mumps, measles and rubella), influenza, Tdap (tetanus, diphtheria and pertussis), meningococcus, hepatitis A and B, rabies, polio, typhoid fever, tick-borne encephalitis and Japanese encephalitis vaccines. They included case series, cohort studies and RCTs. For the meta-analysis, these researchers included only RCTs that compared the administration of a vaccine to placebo or to no vaccine. Outcome measures included severe systemic adverse events (AEs), maternal outcomes related to the course of pregnancy, neonatal outcomes and local AEs. They calculated the RR and its 95 % CI as the summary measure. The safety of influenza vaccine is supported by high-quality evidence. For Tdap vaccine, no evidence of any harm was found in the meta-analysis of RCTs. A slight increase in chorioamnionitis rate was reported in 3 out of 12 observational studies. However, this small possible risk is far out-weighed by a much larger benefit in terms of infant morbidity and mortality. Meningococcal vaccines are probably safe during pregnancy, as supported by RCTs comparing meningococcal vaccines to other vaccines. Data from observational studies support the safety of hepatitis A, hepatitis B and rabies vaccines, as well as that of the live attenuated yellow fever vaccine. The authors found little or no data about the safety of polio, typhoid, Japanese encephalitis, tick-borne encephalitis and MMR vaccines during pregnancy.
Yellow Fever Vaccine Safety in Immunocompromised Individuals
de Araujo Lagos et al (2023) stated that yellow fever (YF) is an arbovirus with variable severity, including severe forms with high mortality; and vaccination is the most effective measure to protect against the disease . Non-serious and serious AEs have been described in immunocompromised individuals; however, previous studies have failed to show this association. In a systematic review, these investigators examined the risk of AEs following YF vaccination in immunocompromised individuals compared with its use in non-immunocompromised individuals. They carried out a literature search in the Medline, LILACS, Embase, SCOPUS, DARE, Toxiline, Web of Science and grey literature databases for publications until February 2021. Randomized and quasi-randomized clinical trials and observational studies that included immunocompromised subjects (individuals with HIV infection, organ transplantation, cancer, who used immunosuppressive drugs for rheumatologic diseases and those on immunosuppressive therapy for other diseases) were selected. The methodological quality of observational or non-randomized studies was assessed by the ROBINS-I tool. These researchers carried out 2 meta-analyses, proportion and risk factor analyses, to identify the summary measure of RR in the studies that had variables suitable for combination. A total of 25 studies were included, most with risk of bias classified as critical; 13 studies had enough data to perform the proposed meta-analyses; 7 studies without a comparator group had their results aggregated in the proportion meta-analysis, identifying an 8.5 % (95 % CI: 0.07 to 21.8] risk of immunocompromised individuals presenting AEs following vaccination; 6 cohort studies were combined, with an RR of 1.00 (95 % CI: 0.78 to 1.29). Subgroup analysis was conducted according to the etiology of immunosuppression and was also unable to identify an increased risk of AEs following vaccination. The authors concluded that it was not possible to affirm that immunocompromised individuals, regardless of etiology, had a higher risk AEs, following receiving the YF vaccine.
Zaire Ebolavirus Vaccine
The Ebola virus [Zaire ebolavirus (EBOV)] vaccine is a replication-competent, live, attenuated recombinant vesicular stomatitis virus (rVSV) vaccine. It contains a gene from the Ebola virus, not the whole virus, which means persons cannot become infected with EBOV from the vaccine. The vaccine is known as rVSVΔG-ZEBOV-GP Ebola vaccine, brand name Ervebo (manufactured by Merck). The vaccine was approved by the U.S. FDA on December 19, 2019, for the prevention of Ebola virus disease (EVD) caused by EBOV in people 18 years of age and older, based on the data from 12 clinical trials that included a total of 15,399 adults (CDC, 2021).
Study 3 (Ring vaccination study) was an open-label, randomized cluster (ring) vaccination study conducted in the Republic of Guinea during the 2014 outbreak. Each cluster was composed of contacts and contacts of contacts of individuals with laboratory-confirmed Ebola virus disease (EVD). Clusters were randomized to receive either an “immediate” vaccination or a 21-day “delayed” vaccination. In the primary efficacy analysis, 3,537 subjects 18 years of age and older were considered contacts and contacts of contacts of an index case with laboratory-confirmed EVD. Of these, 2,108 were included in 51 immediate vaccination clusters, and 1,429 were included in 46 delayed vaccination clusters. In the primary efficacy analysis, the number of cases of laboratory-confirmed EVD in subjects vaccinated in immediate vaccination clusters was compared to the number of cases in subjects in delayed vaccination clusters. Cases of EVD that occurred between Day 10 and Day 31 post-randomization of the cluster were included in the analysis. Vaccine efficacy was 100%; no cases of confirmed EVD were observed in the immediate vaccination clusters, and 10 confirmed cases of EVD were observed in a total of 4 delayed vaccination clusters between Day 10 and Day 31 post-randomization (Merck, 2019).
On February 26, 2020, the Advisory Committee on Immunization Practices (ACIP) recommended pre-exposure vaccination with Ervebo® for adults aged 18 years or older in the U.S. population who are at potential risk of exposure to EBOV. This recommendation includes adults who are responding or may respond to an outbreak of EVD; laboratorians or other staff working at biosafety-level 4 facilities in the United States; or healthcare personnel (HCP) working at federally designated Ebola Treatment Centers in the United States. HCP refers to all paid and unpaid persons serving in healthcare settings who have the potential for direct or indirect exposure to patients or infectious materials, including body substances (e.g., blood, tissue, and specific body fluids); contaminated medical supplies, devices, and equipment; contaminated environmental surfaces; or contaminated air. These HCP include, but are not limited to, emergency medical service personnel, nurses, nursing assistants, physicians, technicians, clinical laboratory personnel, autopsy personnel, therapists, phlebotomists, pharmacists, students and trainees, contractual staff not employed by the healthcare facility, and persons not directly involved in patient care, but who could be exposed to infectious agents that can be transmitted in the healthcare setting (e.g., clerical, dietary, environmental services, laundry, security, engineering and facilities management, administrative, billing, and volunteer personnel) (CDC, 2021).
Ervebo (Merck Sharp & Dohme Corporation) is a vaccine indicated for the prevention of disease caused by Zaire ebolavirus in individuals 18 years of age and older. Immunization with Ervebo results in an immune response and protection from disease caused by Zaire ebolavirus. The relative contributions of innate, humoral and cell-mediated immunity to protection from Zaire ebolavirus are unknown.
Limitations of use include:
- The duration of protection conferred by Ervebo is unknown;
- Ervebo does not protect against other species of Ebolavirus or Marburgvirus;
- Effectiveness of the vaccine when administered concurrently with antiviral medication, immune globulin (IG), and/or blood or plasma transfusions is unknown.
Ervebo is available as 1 mL suspension for injection supplied as a single-dose vial and is to be administered intramuscularly.
Ervebo label carries warnings and precautions for anaphylaxis. Vaccinated individuals should continue to adhere to infection control practices to prevent Zaire ebolavirus infection and transmission. Vaccine virus RNA has been detected in blood, saliva, urine, and fluid from skin vesicles of vaccinated adults; transmission of vaccine virus is a theoretical possibility. The most common injection-site adverse events were injection-site pain (70%), swelling (17%), and redness (12%). The most common systemic adverse events reported were headache (37%), feverishness (34%), muscle pain (33%), fatigue (19%), joint pain (18%), nausea (8%), arthritis (5%), rash (4%) and abnormal sweating (3%) (Merck, 2019).
The above policy is based on the following references:
- Ahmed T, Bhuiyan TR, Zaman K, et al. Vaccines for preventing enterotoxigenic Escherichia coli (ETEC) diarrhoea. Cochrane Database Syst Rev. 2013;7:CD009029.
- American Academy of Pediatrics Committee on Infectious Diseases. Poliovirus. Pediatrics. 2011;128(4):805-808.
- Arguin PM, Kozarsky PE, Reed C, eds. CDC Health Information for International Travel, 2008. St. Louis, MO: Mosby; 2007.
- Bettis AA, L'Azou Jackson M, et al. The global epidemiology of chikungunya from 1999 to 2020: A systematic literature review to inform the development and introduction of vaccines. PLoS Negl Trop Dis. 2022;16(1):e0010069.
- Birkett A. MVI discusses what's on the horizon for malaria vaccine development. Bethesda, MD: The PATH Malaria Vaccine Initiative; 2010.
- Carroll ID, Williams DC. Pre-travel vaccination and medical prophylaxis in the pregnant traveler. Travel Med Infect Dis. 2008;6(5):259-275.
- Centers for Disease Control and Prevention (CDC), National Center for Emerging and Zoonotic Infectious Diseases (NCEZID), Division of High-Consequence Pathogens and Pathology (DHCPP), Viral Special Pathogens Branch (VSPB). Ebola vaccine: Information about Ervebo. Atlanga, GA: CDC; February 25, 2021. Available at: https://www.cdc.gov/vhf/ebola/clinicians/vaccine/index.html. Accessed June 6, 2021.
- Centers for Disease Control and Prevention (CDC), National Center for Emerging and Zoonotic Infectious Diseases (NCEZID). Evidence to recommendations for chikungunya vaccine use among adult travelers. ACIP meeting; October 26, 2023. Available at: https://www.cdc.gov/vaccines/acip/meetings/downloads/slides-2023-10-25-26/02-Chikungunya-Hills-508.pdf. Accessed November 20, 2023.
- Centers for Disease Control (CDC). Rabies prevention -- United States, 1984. MMWR Morbid Mortal Wkly Rep. 1984;33(28):393-402, 407-408.
- Centers for Disease Control and Prevention (CDC). Inactivated Japanese encephalitis virus vaccine. Recommendations of the Advisory Committee on Immunization Practices (ACIP). MMWR Recomm Rep. 1993;42(RR-1):1-15.
- Centers for Disease Control and Prevention (CDC). Prevention and control of meningococcal disease: Recommendations of the Advisory Committee on Immunization Practices (ACIP). MMWR Morbid Mortal Wkly Rep. 2000;49(RR-7):1-10.
- Centers for Disease Control and Prevention (CDC). Prevention of hepatitis A through active or passive immunization: Recommendations of the Advisory Committee on Immunization Practices (ACIP). MMWR Morbid Mortal Wkly Rep. 1999;48(RR-12):1-37.
- Centers for Disease Control and Prevention (CDC). Recommendations for the use of Lyme disease vaccine. Recommendations of the Advisory Committee on Immunization Practices (ACIP). MMWR Recomm Rep. 1999;48(RR-7):1-17, 21-25.
- Centers for Disease Control and Prevention (CDC). Recommendations of the Advisory Committee on Immunization Practices: Revised recommendations for routine poliomyelitis vaccination. MMWR Morbid Mortal Wkly Rep. 1999;48(27):590.
- Centers for Disease Control and Prevention (CDC). The Yellow Book. CDC Health Information for International Travel 2012. New York, NY: Oxford University Press; 2011.
- Centers for Disease Control and Prevention (CDC). The Yellow Book. CDC Health Information for International Travel 2018. New York, NY: Oxford University Press; 2017.
- Centers for Disease Control and Prevention (CDC). Travelers' health: Chapter 4: Travel-related infectious diseases: Hepatitis B. The Yellow Book. Atlanta, GA: CDC; reviewed July 1, 2019. Available at: https://wwwnc.cdc.gov/travel/yellowbook/2020/travel-related-infectious-diseases/hepatitis-b. Accessed April 12, 2022.
- Centers for Disease Control and Prevention (CDC). Typhoid immunization. Recommendations of the Advisory Committee on Immunization Practices (ACIP). MMWR Morbid Mortal Wkly Rep. 1990;39(RR-10):1-5.
- Centers for Disease Control and Prevention (CDC). Update: Recommendations to prevent hepatitis B virus transmission -- United States. MMWR Morbid Mortal Wkly Rep. 1995:44(30):574-575.
- Centers for Disease Control and Prevention (CDC). Update: Recommendations to prevent hepatitis B virus transmission -- United States. MMWR Morbid Mortal Wkly Rep. 1999;48(2):33-34.
- Centers for Disease Control and Prevention. Prevention of plague: Recommendations of the Advisory Committee on Immunization Practices (ACIP). MMWR. 1996;45(No. RR-14).
- Cetron MS, Marfin AA, Julian KG, et al. Yellow fever vaccine: Recommendations of the Advisory Committee on Immunization Practices (ACIP), 2002. MMWR Morbid Mortal Wkly Rep. 2002;51(RR-17):1-11.
- de Araujo Lagos LW, de Jesus Lopes de Abreu A, Caetano R, Braga JU. Yellow fever vaccine safety in immunocompromised individuals: A systematic review and meta-analysis. J Travel Med. 2023;30(2):taac095.
- Fischer M, Lindsey N, Staples JE, Hills S; Centers for Disease Control and Prevention (CDC). Japanese encephalitis vaccines: Recommendations of the Advisory Committee on Immunization Practices (ACIP). MMWR Recomm Rep. 2010;59(RR-1):1-27.
- Fraser A, Goldberg E, Acosta CJ, et al. Vaccines for preventing typhoid fever. Cochrane Database Syst Rev. 2007;(3):CD001261.
- Freedman D, Leder K. Immunizations for travel. UpToDate [online serial]. Waltham, MA: UpToDate; reviewed March 2015; March 2016.
- Garcia Garrido HM, Wieten RW, Grobusch MP, Goorhuis A. Response to hepatitis A vaccination in immunocompromised travelers. J Infect Dis. 2015;212(3):378-385.
- Gautret P, Wilder-Smith A. Vaccination against tetanus, diphtheria, pertussis and poliomyelitis in adult travellers. Travel Med Infect Dis. 2010;8(3):155-160.
- Graves P, Gelband H. Vaccines for preventing malaria (blood-state). Cochrane Database Syst Rev. 2006b;(4):CD006199.
- Graves P, Gelband H. Vaccines for preventing malaria (SPf66). Cochrane Database Syst Rev. 2006a;(2):CD005966.
- Graves PM, Deeks JJ, Demicheli V, Jefferson T. Vaccines for preventing cholera: Killed whole cell or other subunit vaccines (injected). Cochrane Database Syst Rev. 2001;(1):CD000974.
- Houle SKD, Eurich DT. Completion of multiple-dose travel vaccine series and the availability of pharmacist immunizers: A retrospective analysis of administrative data in Alberta, Canada. PLoS One. 2019;14(1):e0211006.
- Hyle EP, Rao SR, Bangs AC, et al. Clinical practices for measles-mumps-rubella vaccination among US pediatric international travelers. JAMA Pediatr. 2020;174(2):e194515.
- Jelinek T. Ixiaro: A new vaccine against Japanese encephalitis. Expert Rev Vaccines. 2009;8(11):1501-1511.
- Jost M, Luzi D, Metzler S, et al. Measles associated with international travel in the region of the Americas, Australia and Europe, 2001-2013: A systematic review. Travel Med Infect Dis. 2015;13(1):10-18.
- Lamarche L, Taucher C. Travel vaccines: Update. Can Pharm J (Ott). 2020;153(2):72-73.
- Lenchow DJ, Wilson ME. Chikungunya fever: Treatment and prevention. UpToDate [online serial]. Waltham, MA: UpToDate; reviewd October 2023.
- Lindsey NP, Staples JE, Fischer M. Chikungunya Virus Disease among Travelers-United States, 2014-2016. Am J Trop Med Hyg. 2018;98(1):192-197.
- Marfin AA, Eidex RS, Kozarsky PE, Cetron MS. Yellow fever and Japanese encephalitis vaccines: Indications and complications. Infect Dis Clin North Am. 2005;19(1):151-168, ix.
- Merck Sharp & Dohme Corporation. Ervebo (Ebola Zaire vaccine, live) suspension for intramuscular injection. Prescribing Information. Whitehouse Station, NJ: Merck; 2019.
- Nasser R, Rakedzon S, Dickstein Y, et al. Are all vaccines safe for the pregnant traveller? A systematic review and meta-analysis. J Travel Med. 2020;27(2).
- Nelson NP, Link-Gelles R, Hofmeister MG, et al. Update: recommendations of the Advisory Committee on Immunization Practices for use of hepatitis A vaccine for postexposure prophylaxis and for preexposure prophylaxis for international travel. MMWR Morb Mortal Wkly Rep. 2018;67(43):1216-1220.
- No authors listed. Cholera vaccine. MMWR Morbid Mortal Wkly Rep. 1988;37(40):617-618, 623-624.
- Pfizer Inc. Ticovac (tick-borne encephalitis vaccine), suspension for intramuscular injection. Prescribing Information. New York, NY: Pfizer; revised August 2021a.
- Pfizer Inc. U.S. FDA accepts for priority review Pfizer’s application for TicoVac (tick-borne encephalitis vaccine). Press Release. New York, NY: Pfizer; February 23, 2021. Available at: https://www.pfizer.com/news/press-release/press-release-detail/us-fda-accepts-priority-review-pfizers-application. Accessed June 7, 2021b.
- Pfizer Inc. U.S. FDA approves Ticovac, Pfizer's tick-borne encephalitis (TBE) vaccine. Press Release. New York, NY: Pfizer; August 13, 2021c.
- Rampa JE, Askling HH, Lang P, et al. Immunogenicity and safety of the tick-borne encephalitis vaccination (2009–2019): A systematic review. Travel Medicine and Infectious Disease. 2020;37:101876.
- Ritz N, Connell TG, Curtis N. To BCG or not to BCG? Preventing travel-associated tuberculosis in children. Vaccine. 2008;26(47):5905-5910.
- Schioler KL, Samuel M, Wai KL Vaccines for preventing Japanese encephalitis. Cochrane Database Syst Rev. 2007;(3):CD004263.
- Staples JE, Gershman M, Fischer M; Centers for Disease Control and Prevention (CDC). Yellow fever vaccine: Recommendations of the Advisory Committee on Immunization Practices (ACIP). MMWR Recomm Rep. 2010;59(RR-7):1-27.
- Taucher C, Barnett ED, Cramer JP, et al. Neutralizing antibody persistence in pediatric travelers from non-JE-endemic countries following vaccination with IXIARO® Japanese encephalitis vaccine: An uncontrolled, open-label phase 3 follow-up study. Travel Med Infect Dis. 2020;34:101616.
- U.S. Food and Drug Administration (FDA). FDA approves first vaccine to prevent disease caused by chikungunya virus. FDA News Release. Silver Spring, MD: FDA; November 13, 2023.
- Valneva Scotland Ltd. Ixchiq (chikungunya vaccine, live) solution for intramuscular injection. Prescribing Information. Livingston, United Kingdom; revised November 2023.
- Vaughan K, Blythe M, Greenbaum J, et al. Meta-analysis of immune epitope data for all Plasmodia: Overview and applications for malarial immunobiology and vaccine-related issues. Parasite Immunol. 2009;31(2):78-97.
- Wanke CA. Travelers' diarrhea. UpToDate [online serial]. Waltham, MA: UpToDate; reviewed March 2014.
- Wilder-Smith A. Meningococcal disease in international travel: Vaccine strategies. J Travel Med. 2005;12 Suppl 1:S22-S29.
- Wilson ME, Lenschow DJ. Chikungunya fever: Epidemiology, clinical manifestations, and diagnosis. UpToDate [online serial]. Waltham, MA: UpToDate; reviewed January 2022.
- Wong KK, Burdette E, Mahon BE, et al. Recommendations of the Advisory Committee on Immunization Practices for Use of Cholera Vaccine. MMWR. 2017;66(18):482-485.
Policy History

Effective: 04/13/2001
Next Review: 05/09/2024
Review History
Definitions
Additional Information
Clinical Policy Bulletin Notes
You are now leaving the Aetna website .
Links to various non-Aetna sites are provided for your convenience only. Aetna Inc. and its subsidiary companies are not responsible or liable for the content, accuracy, or privacy practices of linked sites, or for products or services described on these sites.


Amazon Prime members get special One Medical membership pricing.
Your Guide To Travel Vaccine Costs and Insurance
How do I know if I have coverage?
Navigating the world of healthcare may seem intimidating at first, but we’ve designed it to be easy to use. With a variation of plans not all vaccinations are covered by insurance.
We have a step by step guide below that will help you check your coverage! Even if you think you may need to pay for travel medications or vaccines out-of-pocket, it is important to first start with finding out the details from your insurer. If you have any questions at all about paying directly, please call your office and an administrator will talk you through all the details.
1.Find the Customer Support number on the back of your insurance card and call to ask what your specific policy covers as far as travel-related benefits and coverage.
- Follow phone prompts to connect with a representative.
- You may need to go through an automated system before talking with a live representative
- You may also need to provide your insurance information during this step, or with the representative during the next step
- When connected with a representative you can say:
- “I would like to check my coverage for the typhoid vaccine. I have the billing codes if that will help.”
- The representative may reference “CPT codes” which just means the billing codes for the vaccine
- Find your vaccine or titer requested below and read the corresponding codes:
Vaccines (read BOTH codes if two codes listed)
- Hepatitis A: 90632 & 90471
- Hepatitis B: 90746 & 90471
- Tetanus & Diphtheria: 90714 & 90471
- Tetanus, Diphtheria & Acellular Pertussis: 90715 & 90471
- Measles, Mumps & Rubella: 90707 & 90471
- Typhoid: 90691 & 90471
- Yellow Fever: 90717 & 90471
- Meningococcus: 90734 & 90471
- Polio - 90713 & 90471
- Hepatitis A Virus (HAV) Antibody, Total - 86708
- Hepatitis B Surface Antibody, Quantitative - 86317
- Measles, Mumps, Rubella (MMR) Immunity Profile - This code varies based upon service area. 86735; 86762; 86765 (feel free to reach out to our administrative team to confirm).
Please note malaria prevention, altitude treatment and motion sickness prevention are pharmacy prescriptions. You may reach out to your local pharmacy to determine the cost.
- The representative will tell you whether or not your plan covers the vaccine
- If you have a deductible that has not been met, the vaccine may be billed against that, which means you will be responsible for the cost.
Please note there is a corresponding vaccination administration fee when you receive the injection in the office for vaccines. This is paid at the office during your vaccination visit. If you have any further questions about the cost of travel-related health care, give us a call or send us a message, and an administrator will make sure you have the information you need.
Learn how UpToDate can help you.
Select the option that best describes you
- Medical Professional
- Resident, Fellow, or Student
- Hospital or Institution
- Group Practice
- Patient or Caregiver
- Find in topic
RELATED TOPICS
INTRODUCTION
Immunization needs are based on the traveler's prior immunizations, health conditions, and likely exposures while traveling ( table 1 ). Those exposures depend upon the countries and regions to be visited and on the nature of potential exposures to infectious agents. For example, travelers with short-term tourism itineraries may have different requirements from those with longer-term occupational exposures. A pretravel consultation enables updating of routine immunizations to protect against illness due to infections for which there is an increased risk of exposure during travel (such as diphtheria, measles, mumps, and varicella) [ 3 ].
Issues related to immunizations for travelers are reviewed here. Other travel-related medical issues and measures to prevent malaria are discussed separately. (See "Travel advice" and "Prevention of malaria infection in travelers" .)
WEBSITES FOR ADDITIONAL GUIDANCE
● United States Centers for Disease Control and Prevention (CDC) – Information on the indications, dosing, side effects, timing, and contraindications for immunizations in travelers are provided by the CDC in a biennial, Health Information for International Travel [ 4 ], with ongoing updates in an online version.
● World Health Organization (WHO) – The WHO also has online information that includes vaccines or dosing regimens approved outside the United States [ 5 ]. Information may be found on the CDC website and the WHO website . Guidance may also be found via GlobalTravEpiNet (GTEN), which has web-based tools for providers and patients based on CDC recommendations.
You are using an outdated browser. Upgrade your browser today or install Google Chrome Frame to better experience this site.
Need travel vaccines? Plan ahead.
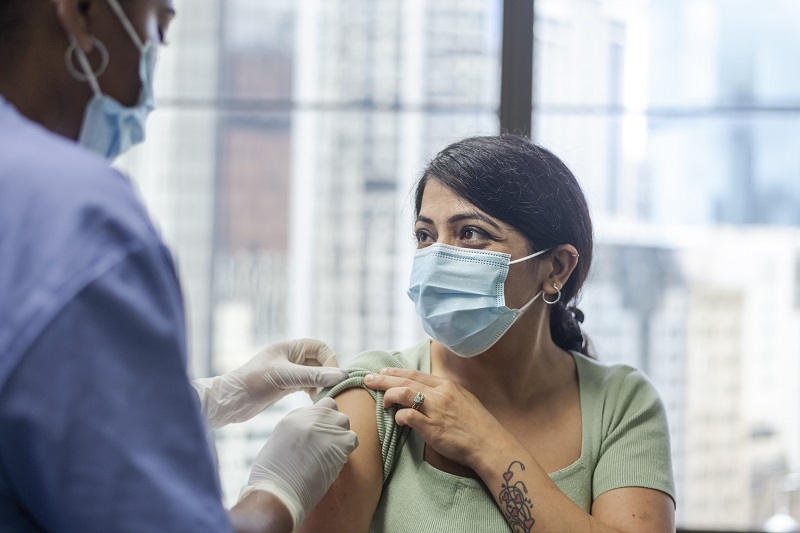
International travel increases your chances of getting and spreading diseases that are rare or not found in United States. Find out which travel vaccines you may need to help you stay healthy on your trip.
Before Travel
Make sure you are up-to-date on all of your routine vaccines . Routine vaccinations protect you from infectious diseases such as measles that can spread quickly in groups of unvaccinated people. Many diseases prevented by routine vaccination are not common in the United States but are still common in other countries.
Check CDC’s destination pages for travel health information . Check CDC’s webpage for your destination to see what vaccines or medicines you may need and what diseases or health risks are a concern at your destination.
Make an appointment with your healthcare provider or a travel health specialist that takes place at least one month before you leave. They can help you get destination-specific vaccines, medicines, and information. Discussing your health concerns, itinerary, and planned activities with your provider allows them to give more specific advice and recommendations.
Because some vaccines require multiple doses, it’s best to see your health care provider as soon as possible.
Medicines to prevent malaria are pills that you start to take before travel. Take recommended medicines as directed. If your health care provider prescribes medicine for you, take the medicine as directed before, during, and after travel.
Where can I get travel vaccines?
You may be able to get some travel vaccines from your primary healthcare provider. If you or your healthcare provider need help finding a location that provides certain vaccines or medicines, visit CDC’s Find a Clinic page.
If yellow fever vaccine is recommended or required for your destination, you’ll need to go to a vaccine center authorized to give yellow fever vaccinations. Many yellow fever vaccine centers also provide other pre-travel health care services. Find an authorized US yellow fever vaccine center .
Examples of Vaccines
Here is a list of possible vaccines that you may need to get for the first time or boosters before you travel.
- Cholera
- Flu (Influenza)
- Hepatitis A
- Hepatitis B
- Japanese encephalitis
- MMR (Measles, Mumps, Rubella)
- Meningococcal
- Pneumococcal
- Polio
- Rabies
- Tdap (Tetanus, Diphtheria, Pertussis)
- Typhoid
- Yellow fever
More Information
CDC Yellow Book: Travel Vaccine Summary Table
File Formats Help:
- Adobe PDF file
- Microsoft PowerPoint file
- Microsoft Word file
- Microsoft Excel file
- Audio/Video file
- Apple Quicktime file
- RealPlayer file
- Zip Archive file
Exit Notification / Disclaimer Policy
- The Centers for Disease Control and Prevention (CDC) cannot attest to the accuracy of a non-federal website.
- Linking to a non-federal website does not constitute an endorsement by CDC or any of its employees of the sponsors or the information and products presented on the website.
- You will be subject to the destination website's privacy policy when you follow the link.
- CDC is not responsible for Section 508 compliance (accessibility) on other federal or private website.
Get Vaccinated Before You Travel
It’s important to plan ahead to get the shots required for all countries you and your family plan to visit.
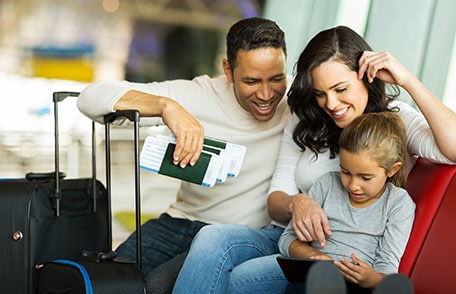
Protect your child and family when traveling in the United States or abroad by:
- Getting the shots required for all countries you and your family plan to visit during your trip
- Making sure you and your family are up-to-date on all routine U.S. vaccines
- Staying informed about travel notices and alerts and how they can affect your family’s travel plans
Avoid getting sick or coming back home and spreading the disease to others.
Vaccinate at least a month before you travel
See your doctor when you start to plan your trip abroad. It’s important to do this well in advance.
- Your body needs time to build up immunity.
- You may need several weeks to get all the doses of the vaccine.
- Your primary doctor may not stock travel vaccines. Visit a travel medical clinic .
- You’ll need time to prepare for your pre-travel appointment .
- If the country you visit requires a yellow fever vaccine , only a limited number of clinics have the vaccine and will probably be some distance from where you live. You must get it at least 10 days before travel.
Find out which vaccines are recommended or required for the countries you plan to visit .
TIP : Save time by getting routine vaccines during the same doctor visit. Use the Vaccine Self-Assessment Tool and discuss the results with your doctor. It tells you which U.S. recommended vaccines you (19 years and older) or your child (birth – 18 years) might need.
Last-minute travelers
When traveling to another country be aware your doctor may not carry a travel vaccine and you may have to visit a medical clinic.
Many travel vaccines require multiple shots or take time to become fully effective. But some multiple-dose vaccines (like hepatitis A) can still give you partial protection after just one dose. Some can also be given on an “accelerated schedule,” meaning doses are given in a shorter period of time.
- Discover and learn about specific diseases that can affect you while traveling
- What to do if you get sick after traveling
- Vaccines & Immunizations
Exit Notification / Disclaimer Policy
- The Centers for Disease Control and Prevention (CDC) cannot attest to the accuracy of a non-federal website.
- Linking to a non-federal website does not constitute an endorsement by CDC or any of its employees of the sponsors or the information and products presented on the website.
- You will be subject to the destination website's privacy policy when you follow the link.
- CDC is not responsible for Section 508 compliance (accessibility) on other federal or private website.
- Request An Appointment
- Pay a Bill or Get an Estimate
- For Referring Providers
- Pediatric Care
- Cancer Center
- Carver College of Medicine
- Find a Provider
- Share Your Story
- Health Topics
- Educational Resources & Support Groups
- Clinical Trials
- Medical Records
- Info For... Directory
Travel Medicine Clinic
- Call to Schedule 1-319-356-4252
How to prepare for your Travel Clinic visit
Schedule your visit six to eight weeks before your travel departure to allow enough time to receive any needed vaccines and allow for immunity to develop before your trip begins.
Please arrive 15 minutes in advance of your appointment time to allow for check-in.
Bring your travel itinerary, including dates of travel, regions of the countries you plan to visit, expected accommodations, and scheduled airport layovers.
Bring your current medication list and vaccination records that are not already part of your medical record. If you are a UI Health Care employee, please print your vaccine/immunization record from ReadySet and bring it with you.
The clinic provider will not be able to determine insurance coverage for your visit, vaccinations, or prescribed medications. We recommend you review the Centers for Disease Control and Prevention (CDC) Travelers’ Health webpage in advance to learn what are likely vaccines and medications recommended for your destination. Use the drop-down menu under the “Destinations” heading to select the country you’ll be visiting and click “go.” You will need the CPT (Current Procedural Terminology) codes listed in the tables below for vaccine information and some other fees when you contact your health insurance carrier to inquire about coverage. Medications commonly recommended for travel are also found in the tables.
A cost estimator tool for a travel clinic visit can be found within MyChart . However, the tool allows you to only inquire about one vaccine per inquiry. The CPT codes listed below are needed to use the tool. Note that the estimator automatically includes a flat physician fee for a new patient visit and a hospital fee when a vaccine CPT code is entered. However, the provider charge submitted varies with the complexity/duration of the travel assessment/counseling.
To access the estimator from MyChart, click “Menu” in top left corner, scroll down to “Billing,” and then click “Estimates.” Click “Create A New Estimate,” then type in the desired vaccine or its associated CPT code into the search bar. If you have not signed up for a MyChart account, you can use the estimator as a guest.
Vaccines that may be recommended and provided during Travel Medicine Clinic visits
Medications that may be recommended/prescribed during travel medicine clinic visits, what to expect during your travel clinic visit.
- Your provider will review your travel itinerary, planned activities, medical history, medication list, and prior vaccinations. They will also discuss precautions to help you stay well, including recommended vaccines and prescription medications to prevent or treat common or important travel-related illnesses. The vaccines that you want or are required then will be administered, and medications will be prescribed.
- The visit may last up to 75 minutes, including the travel assessment and counseling and administration of vaccines. If more than one person is sharing an appointment, the total visit may require 90 minutes. If a yellow fever vaccine is administered, 15 minutes of monitoring in the clinic is required.
- We see adults and children who are age 15 and older. We also see 14-year-old patients who weigh more than 100 pounds.
Note: Children who do not meet age/weight criteria to be seen in our adult Travel Clinic can be seen in the Pediatric Infectious Diseases Clinic . To schedule an appointment, call 1-888-573-5437 . Please inform the scheduler it is for a pre-travel visit.
Our Care Team
Infectious disease specialist.
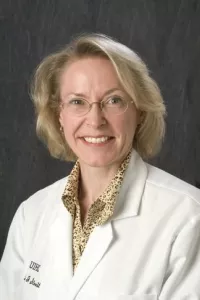
Judy Streit MD
- Infectious Diseases
Physician Assistant
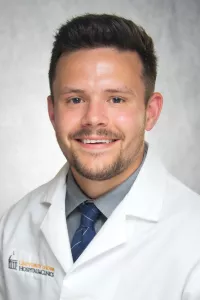
Troy Smith PA-C
- Orthopedics and Rehabilitation
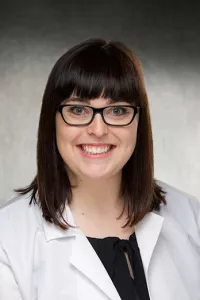
Laura Stulken PA-C, MPH
Locations and offices, medicine specialty clinics.
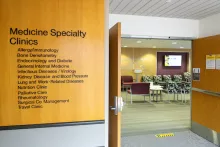
Disclaimer » Advertising
- HealthyChildren.org
- Previous Article
- Next Article
You Code It! Travel Counseling
- Split-Screen
- Article contents
- Figures & tables
- Supplementary Data
- Peer Review
- CME Quiz Close Quiz
- Open the PDF for in another window
- Get Permissions
- Cite Icon Cite
- Search Site
American Academy of Pediatrics; You Code It! Travel Counseling. AAP Pediatric Coding Newsletter February 2020; 15 (5): 8. 10.1542/pcco_book190_document003
Download citation file:
- Ris (Zotero)
- Reference Manager
Code Z71.84 was added to International Classification of Diseases, 10th Revision, Clinical Modification ( ICD-10-CM ) on October 1, 2019, for reporting travel counseling. When travel counseling is the only service provided at an encounter, a preventive medicine counseling code ( 99401–99404 ) is appropriately reported with code Z71.84 . Can you determine the correct codes for travel counseling provided at the same encounter as other services in the following scenarios? Answers can be found later in this newsletter.
A 13-year-old established patient presents for a routine health examination during which her parents request advice on health safety during an upcoming monthlong stay overseas. The pediatrician spends approximately 10 minutes discussing potential health concerns, including recommended prophylactic immunization, medical evacuation insurance, and referral to a travel clinic that can provide a recommended immunization not available in the pediatrician’s practice. Diagnoses are routine health examination and pretravel counseling.
A 45-month-old girl presents with an injury to her left foot from stepping on a roofing staple in her backyard. The child’s parents note concerns that they will travel overseas to visit family in 6 weeks. They ask questions about health concerns and receiving health care during their visit.
A pediatrician evaluates and bandages the small puncture wounds and recommends early administration of diphtheria, tetanus, and acellular pertussis (DTaP) vaccine and other immunizations that would otherwise be given when the patient is 4 years old. The pediatrician documents 15 minutes spent reviewing concerns for the areas the family will visit and providing recommendations. Routine immunizations are provided with the pediatrician counseling for DTaP, poliovirus (IPV), and measles, mumps, rubella, and varicella (MMRV) vaccines. A referral to a travel clinic is provided for other immunizations not available in the pediatric practice.
The pediatrician’s total face-to-face time of the visit is 25 minutes. Diagnoses are puncture wound left foot, routine immunization, and counseling for travel.
Recipient(s) will receive an email with a link to 'You Code It! Travel Counseling' and will not need an account to access the content.
Subject: You Code It! Travel Counseling
(Optional message may have a maximum of 1000 characters.)
Citing articles via
Email alerts.
Advertising Disclaimer »
Affiliations
- CEU Quizzes
- Coding Hotline
- Online ISSN 1934-5143
- Print ISSN 1934-5135
- Pediatrics Open Science
- Hospital Pediatrics
- Pediatrics in Review
- AAP Grand Rounds
- Latest News
- Pediatric Care Online
- Red Book Online
- Pediatric Patient Education
- AAP Toolkits
- AAP Pediatric Coding Newsletter
First 1,000 Days Knowledge Center
Institutions/librarians, group practices, licensing/permissions, integrations, advertising.
- Privacy Statement | Accessibility Statement | Terms of Use | Support Center | Contact Us
- © Copyright American Academy of Pediatrics
This Feature Is Available To Subscribers Only
Sign In or Create an Account
An official website of the United States government
Here's how you know
Official websites use .gov A .gov website belongs to an official government organization in the United States.
Secure .gov websites use HTTPS A lock ( ) or https:// means you've safely connected to the .gov website. Share sensitive information only on official, secure websites.
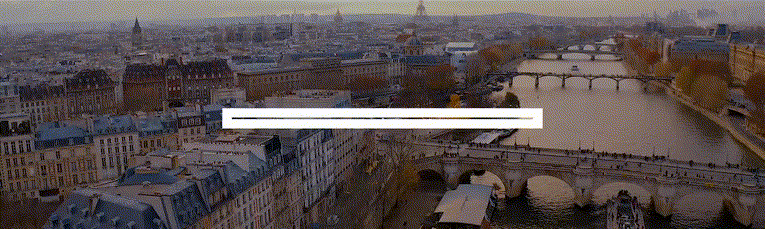
CMS Newsroom
Search cms.gov.
- Physician Fee Schedule
- Local Coverage Determination
- Medically Unlikely Edits
List of CPT/HCPCS Codes
We maintain and annually update a List of Current Procedural Terminology (CPT)/Healthcare Common Procedure Coding System (HCPCS) Codes (the Code List), which identifies all the items and services included within certain designated health services (DHS) categories or that may qualify for certain exceptions. We update the Code List to conform to the most recent publications of CPT and HCPCS codes and to account for changes in Medicare coverage and payment policies. Code List updates for years 2022 and earlier were published in the Federal Register as an addendum to the annual Physician Fee Schedule final rule.
Beginning with the Code List effective January 1, 2023, updates are published solely on this webpage. On or before December 2 nd of each year, we will publish the annual update to the Code List and provide a 30-day public comment period using www.regulations.gov . To be considered, comments must be received within the stated 30-day timeframe. We anticipate that most comments will be addressed by April 1 st ; however, a longer timeframe may be necessary to address complex comments or those that require coordination with external parties. If no comments are received, in lieu of a comment response, we will publish a note below the applicable Code List year stating so.
2024 Annual Update to the Code List
Below you will find the Calendar Year (CY) 2024 Code List published November 29, 2023 and a description of the revisions for CY 2024, our response to comments on that Code List, and the updated CY 2024 Code List, which is effective January 1, 2024 unless otherwise indicated on the Code List.
- UPDATED list of codes effective January 1, 2024, published March 1, 2024 (all codes effective January 1, 2024 unless otherwise indicated on the Code List) (ZIP)
- List of codes effective January 1, 2024, published November 29, 2023 (ZIP)
- Annual Update to the List of CPT/HCPCS Codes Effective January 1, 2024 (PDF)
We received one comment related to the additions, deletions, and corrections to the codes on the Code List effective January 1, 2024. Our response to this comment is below. We also received one comment related to Medicare coverage for platelet-rich plasma treatments. We consider this comment to be outside the scope of the annual update. CMS does not respond to out of scope comments on the annual updates to the Code List.
Comment : One commenter noted that, although most Hepatitis B vaccine codes are identified on the Code List as CPT/HCPCS codes to which the exception for preventive screening tests and vaccines at § 411.355(h) applies, the Hepatitis B vaccine associated with CPT code 90739 was not listed. The commenter requested that CPT code 90739 be added to the list of vaccine codes to which the exception for preventive screening tests and vaccines at §411.355(h) applies, effective retroactively to January 1, 2024.
Response : We agree with the commenter that the exception for preventive screening tests and vaccines at § 411.355(h) should apply to CPT code 90739 and are revising the Code List accordingly. The applicability of the exception for preventive screening tests and vaccines to CPT code 90739 is prospective only and effective on the date indicated on the UPDATED list of codes.
In considering this comment, we also identified two CPT codes (90653 and 90658, both flu vaccines) that were inadvertently left off of the list of codes to which the exception for preventive screening tests and vaccines at § 411.355(h) should apply. Accordingly, we are adding these CPT codes to the list of codes to which the exception at § 411.355(h) applies, effective on the date indicated on the UPDATED list of codes.
2023 Annual Update to the Code List
Below you will find the Code List that is effective January 1, 2023 and a description of the revisions effective for Calendar Year 2023.
- List of codes effective January 1, 2023, published December 1, 2022
- Annual Update to the List of CPT/HCPCS Codes Effective January 1, 2023, published December 1, 2022 (PDF)
The comment period ended December 30, 2022. We did not receive any comments related to the additions, deletions, and corrections to the codes on the Code List effective January 1, 2023. We received one (1) comment related to the supervision level required for specific services. We consider this comment to be outside the scope of the annual update. CMS does not respond to out of scope comments on the annual updates to the Code List.
DHS Categories
The DHS categories defined by the Code List are:
- clinical laboratory services;
- physical therapy services, occupational therapy services, outpatient speech-language pathology services;
- radiology and certain other imaging services; and
- radiation therapy services and supplies.
The Code List also identifies those items and services that may qualify for either of the following two exceptions to the physician self-referral prohibitions:
- EPO and other dialysis-related drugs (42 CFR § 411.355(g)).
- Preventive screening tests and vaccines (42 CFR § 411.355(h)).
NOTE: The following DHS categories are defined at 42 CFR §411.351 without reference to the Code List:
- durable medical equipment and supplies;
- parenteral and enteral nutrients, equipment and supplies;
- prosthetics, orthotics, and prosthetic devices and supplies;
- home health services;
- outpatient prescription drugs; and
- inpatient and outpatient hospital services.
Related Links
- List of codes effective January 1, 2022, published November 19, 2021
- List of codes effective January 1, 2021, issued December 1, 2020
- List of codes effective January 1, 2020, published December 2, 2019
- List of codes effective January 1, 2019, published November 23, 2018
- List of codes effective January 1, 2018, published November 3, 2017 [ZIP, 59KB]
- List of codes effective January 1, 2017, published November 16, 2016 [ZIP, 54KB]
- List of codes effective January 1, 2016, published October 30, 2015 [ZIP, 58KB]
- List of codes effective January 1, 2015, published November 13, 2014 (79 FR 67972) [ZIP, 54KB]
- List of codes effective January 1, 2014, published December 10, 2013 (78 FR 74791) [ZIP, 54KB]
- List of codes effective January 1, 2013, published November 16, 2012 (77 FR 69334) [ZIP, 54KB]

You must have JavaScript enabled in order to access this part of the site. Please enable JavaScript and then reload this page in order to continue.
WARNING: THIS IS A TEXAS HEALTH AND HUMAN SERVICES INFORMATION RESOURCES SYSTEM THAT CONTAINS STATE AND/OR U.S. GOVERNMENT INFORMATION. BY USING THIS SYSTEM YOU ACKNOWLEDGE AND AGREE THAT YOU HAVE NO RIGHT OF PRIVACY IN CONNECTION WITH YOUR USE OF THE SYSTEM OR YOUR ACCESS TO THE INFORMATION CONTAINED WITHIN IT. BY ACCESSING AND USING THIS SYSTEM YOU ARE CONSENTING TO THE MONITORING OF YOUR USE OF THE SYSTEM, AND TO SECURITY ASSESSMENT AND AUDITING ACTIVITIES THAT MAY BE USED FOR LAW ENFORCEMENT OR OTHER LEGALLY PERMISSIBLE PURPOSES. ANY UNAUTHORIZED USE OR ACCESS, OR ANY UNAUTHORIZED ATTEMPTS TO USE OR ACCESS, THIS SYSTEM MAY SUBJECT YOU TO DISCIPLINARY ACTION, SANCTIONS, CIVIL PENALTIES, OR CRIMINAL PROSECUTION TO THE EXTENT PERMITTED UNDER APPLICABLE LAW. ----------------------- AMA/ADA End User License Agreement LICENSE FOR USE OF CURRENT PROCEDURAL TERMINOLOGY, FOURTH EDITION ("CPT® ")
CPT only copyright 2023 American Medical Association. ALL rights reserved. CPT is a registered trademark of American Medical Association.
You, your employees and agents are authorized to use CPT only as contained in materials on the Texas Medicaid & Healthcare Partnership (TMHP) website solely for your own personal use in directly participating in healthcare programs administered by THHS. You acknowledge that AMA holds all copyright, trademark and other rights in CPT.
Any use not authorized herein is prohibited, including by way of illustration and not by way of limitation, making copies of CPT for resale and/or license, transferring copies of CPT to any party not bound by this agreement, creating any modified or derivative work of CPT, or making any commercial use of CPT. License to use CPT for any use not authorized herein must be obtained through the American Medical Association, Intellectual Property Services, 515 N. State Street, Chicago, Illinois, 60610. Applications are available at the American Medical Association website, www.ama-assn.org/go/cpt .
U.S. Government Rights
This product includes CPT which is commercial technical data and/or computer databases and/or commercial computer software documentation, as applicable which were developed exclusively at private expense by the American Medical Association, 515 North State Street, Chicago, Illinois, 60610. U.S. Government rights to use, modify, reproduce, release, perform, display, or disclose these technical data and/or computer databases and/or computer software and/or computer software documentation are subject to the limited rights restrictions of DFARS 252.227-7015(b)(2) (November 1995) and/or subject to the restrictions of DFARS 227.7202-1(a) (June 1995) and DFARS 227.7202-3(a) (June 1995), as applicable for U.S. Department of Defense procurements and the limited rights restrictions of FAR 52.227-14 (June 1987) and/or subject to the restricted rights provisions of FAR 52.227-14 (June 1987) and FAR 52.227-19 (June 1987), as applicable, and any applicable agency FAR Supplements, for non-Department of Defense Federal procurements.
Disclaimer of Warranties and Liabilities
CPT is provided "as is" without warranty of any kind, either expressed or implied, including but not limited to the implied warranties of merchantability and fitness for a particular purpose. Fee schedules, relative value units, conversion factors and/or related components are not assigned by the AMA, are not part of CPT, and the American Medical Association (AMA) is not recommending their use. The AMA does not directly or indirectly practice medicine or dispense medical services. The responsibility for the content of this product is with THHS, and no endorsement by the AMA is intended or implied. The AMA disclaims responsibility for any consequences or liability attributable to or related to any use, non-use, or interpretation of information contained or not contained in this product.
This Agreement will terminate upon notice if you violate its terms. The AMA is a third party beneficiary to this Agreement.
Should the for egoing terms and conditions be acceptable to you, please indicate your agreement and acceptance by clicking below on the button labeled "accept".
These materials contain Current Dental Terminology, Fourth Edition (CDT), Copyright © 2023 American Dental Association (ADA). All rights reserved. CDT is a trademark of the ADA.
THE LICENSE GRANTED HEREIN IS EXPRESSLY CONTINUED UPON YOUR ACCEPTANCE OF ALL TERMS AND CONDITIONS CONTAINED IN THIS AGREEMENT. BY CLICKING BELOW ON THE BUTTON LABELED "ACCEPT", YOU HEREBY ACKNOWLEDGE THAT YOU HAVE READ, UNDERSTOOD, AND AGREED TO ALL TERMS AND CONDITIONS SET FORTH IN THIS AGREEMENT.
IF YOU DO NO AGREE WITH ALL TERMS AND CONDITIONS SET FORTH HEREIN, CLICK BELOW ON THE BUTTON LABELED "DO NOT ACCEPT" AND EXIT FROM THIS COMPUTER SCREEN.
IF YOU ARE ACTING ON BEHALF OF AN ORGANIZATION, YOU REPRESENT THAT YOU ARE AUTHORIZED TO ACT ON BEHALF OF SUCH ORGANIZATION AND THAT YOUR ACCEPTANCE OF THE TERMS OF THIS AGREEMENT CREATES A LEGALLY ENFORCEABLE OBLIGATION OF THE ORGANIZATION. AS USED HEREIN, "YOU" AND "YOUR" REFER TO YOU AND ANY ORGANIZATION ON BEHALF OF WHICH YOU ARE ACTING.
1. Subject to the terms and conditions contained in this Agreement, you, your employees and agents are authorized to use CDT only as contained in the following authorized materials and solely for internal use by yourself, employees and agents within your organization within the United States and its territories. Use of CDT is limited to use in programs administered by Centers for Medicare & Medicaid Services (CMS). You agree to take all necessary steps to ensure that your employees and agents abide by the terms of this agreement. You acknowledge that the ADA holds all copyright, trademark and other rights in CDT. You shall not remove, alter, or obscure any ADA copyright notices or other proprietary rights included in the materials.
2. Any use not authorized herein is prohibited, including by way of illustration and not by way of limitation, making copies of CDT for resale and/or license, transferring copies of CDT to any party not bound by this agreement, creating any modified or derivative work of CDT, or making any commercial use of CDT. License to use CDT for any use not authorized herein must be obtained through the American Dental Association, 211 East Chicago Avenue, Chicago IL 60611. Applications are available at the American Dental Association web site, http://www.ADA.org .
3. U.S. GOVERNMENT RIGHTS. Applicable Federal Acquisition Regulation Clauses (FARS)\Department of Defense Federal Acquisition Regulation Supplement (DFARS) Restrictions Apply to Government Use. This product includes CDT, which is commercial technical data and/or computer data bases and/or commercial computer software and/or commercial computer software documentation, as applicable, which was developed exclusively at private expense by the American Dental Association, 211 East Chicago Avenue, Chicago Illinois, 60611. U.S. Government rights to use, modify, reproduce, release, perform, display, or disclose these technical data and/or computer data bases and/or computer software and/or computer software documentation are subject to the limited rights restrictions of DFARS 252.227-7015(b)(2) (June 1995) and/or subject to the restrictions of DFARS 227.7202-1(a) (June 1995) and DFARS 227.7202-3(a) (June 1995), as applicable for U.S. Department of Defense procurements and the limited rights restrictions of FAR 52.227-14 (June 1987) and/or subject to the restricted rights provisions of FAR 52.227-14 (June 1987) and FAR 52.227-19 (June 1987), as applicable, and any applicable agency FAR Supplements, for non-Department of Defense Federal Procurements.
4. ADA DISCLAIMER OF WARRANTIES AND LIABILITIES. CDT is provided “as is” without warranty of any kind, either expressed or implied, including but not limited to, the implied warranties of merchantability and fitness for a particular purpose. No fee schedules, basic unit, relative values or related listings are included in CDT. The ADA does no t directly or indirectly practice medicine or dispense dental services. The sole responsibility for the software, including any CDT and other content contained therein, is with TMHP or the CMS; and no endorsement by the ADA is intended or implied. The ADA expressly disclaims responsibility for any consequences or liability attributable to or related to any use, non-use, or interpretation of information contained or not contained in this file/product. This Agreement will terminate upon notice to you if you violate the terms of the Agreement. The ADA is a third party beneficiary to this Agreement.
5. CMS DISCLAIMER. The scope of this license is determined by the ADA, the copyright holder. Any questions pertaining to the license or use of the CDT should be addressed to the ADA. End Users do not act for or on behalf of the CMS. CMS DISCLAIMS RESPONSIBILITY FOR ANY LIABILITY ATTRIBUTABLE TO END USER USE OF THE CDT. CMS WILL NOT BE LIABLE FOR ANY CLAIMS ATTRIBUTABLE TO ANY ERRORS, OMISSIONS, OR OTHER INACCURACIES IN THE INFORMATION OR MATERIAL COVERED BY THIS LICENSE. In no event shall CMS be liable for direct, indirect, special, incidental, or consequential damages arising out of the use of such information or material.
The license granted herein is expressly conditioned upon your acceptance of all terms and conditions contained in this agreement. If the foregoing terms and conditions are acceptable to you, please indicate your agreement by clicking below on the button labeled "ACCEPT". If you do not agree to the terms and conditions, you may not access or use the software. Instead, you must exit from this computer screen.
Vaccine Services Procedure Code 90623 Is Now Available Through TVFC Effective April 1, 2024
Note: Texas Medicaid managed care organizations (MCOs) must provide all medically necessary, Medicaid-covered services to Medicaid members who are enrolled in their MCO. Administrative procedures, such as prior authorization, precertification, referrals, and claims and encounter data filing, may differ from traditional Medicaid (fee-for-service) and from MCO to MCO. Providers should contact the member’s specific MCO for details.
Effective for dates of service on or after April 1, 2024, vaccine services procedure code 90623 is available through the Texas Vaccines for Children (TVFC) program and will now process as informational only for clients who are 10 years through 18 years of age.
Affected claims that are submitted with dates of service from April 1, 2024, through July 1, 2024, will be reprocessed. Providers may receive an additional payment, which will be reflected on future Remittance and Status (R&S) Reports.
For more information, call the TMHP Contact Center at 800-925-9126.
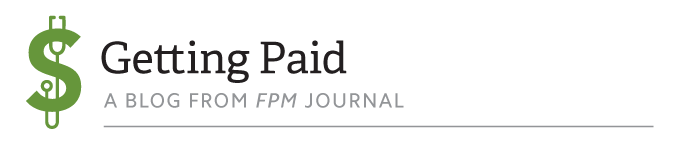
CMS creates new codes for stand-alone vaccine counseling
The Centers for Medicare & Medicaid Services (CMS) released HCPCS codes for vaccine counseling this week. The codes are to be used when a physician counsels a patient about receiving a recommended vaccine and the patient (or patient’s parent or other health care agent) chooses not to have the vaccine administered that day.
In a letter to the states , CMS officials said physicians and other health care clinicians may use the codes to bill for “stand-alone vaccine counseling,” including COVID-19 vaccine counseling provided to Medicaid and Children’s Health Insurance Program (CHIP) beneficiaries who are eligible for coverage as required by the American Rescue Plan and the Medicaid Early and Periodic Screening, Diagnosis and Treatment (EPSDT) provisions.
CMS is allowing the codes to be used for vaccine counseling provided via telehealth as well as in person, but states can decide whether to cover these services via telehealth for Medicaid EPSDT. CMS also said that in this case, “stand-alone” counseling only means that the vaccine discussed is not administered at that visit. The codes may be used for visits that include other services.
G0310 - Immunization counseling by a physician or other qualified health care professional when the vaccine(s) is not administered on the same date of service, 5-15 minutes. (This code is used for Medicaid billing purposes.)
G0311 - Immunization counseling by a physician or other qualified health care professional when the vaccine(s) is not administered on the same date of service, 16-30 minutes. (This code is used for Medicaid billing purposes.)
G0312 - Immunization counseling by a physician or other qualified health care professional when the vaccine(s) is not administered on the same date of service for ages under 21, 5-15 minutes. (This code is used for Medicaid billing purposes.)
G0313 - Immunization counseling by a physician or other qualified health care professional when the vaccine(s) is not administered on the same date of service for ages under 21, 16-30 minutes. (This code is used for Medicaid billing purposes.)
G0314 - Immunization counseling by a physician or other qualified health care professional for COVID-19, ages under 21, 16-30 minutes. (This code is used for the Medicaid EPSDT benefit).
G0315 - Immunization counseling by a physician or other qualified health care professional for COVID-19, ages under 21, 5-15 minutes. (This code is used for the Medicaid EPSDT benefit).
CMS officials noted that Medicaid beneficiaries have some of the lowest COVID-19 vaccination rates among patients for whom COVID shots are recommended and that vaccination rates for other shots have also fallen for Medicaid beneficiaries during the pandemic. The agency wrote that the counseling represented by the new codes “has been shown to help address vaccine hesitancy by helping beneficiaries and their families learn about vaccines from trusted health care providers.”
— Kent Moore, senior strategist for physician payment, American Academy of Family Physicians
Posted on June 13, 2022, by Kent Moore
Get "Quick Tips" in your inbox
Sign up to receive FPM 's free, weekly e-newsletter, "Quick Tips & Insights," featuring practical, peer-reviewed advice for improving practice, enhancing the patient experience, and developing a rewarding career.
- Chronic care
- Medicare/Medicaid
- Physician compensation
- Practice management
- Reimbursement
- Value-based payment
Other Blogs
- Quick Tips from FPM journal
- AFP Community Blog
- FPs on the Front Lines
- Fresh Perspectives
- In the Trenches
- Leader Voices
- RSS ( About RSS )
Disclaimer: The opinions and views expressed here are those of the authors and do not necessarily represent or reflect the opinions and views of the American Academy of Family Physicians. This blog is not intended to provide medical, financial, or legal advice. Some payers may not agree with the advice given. This is not a substitute for current CPT and ICD-9 manuals and payer policies. All comments are moderated and will be removed if they violate our Terms of Use .
Copyright © 2024 American Academy of Family Physicians. All Rights Reserved.
Thank you for visiting nature.com. You are using a browser version with limited support for CSS. To obtain the best experience, we recommend you use a more up to date browser (or turn off compatibility mode in Internet Explorer). In the meantime, to ensure continued support, we are displaying the site without styles and JavaScript.
- View all journals
- My Account Login
- Explore content
- About the journal
- Publish with us
- Sign up for alerts
- Open access
- Published: 18 April 2024
An Omicron-specific, self-amplifying mRNA booster vaccine for COVID-19: a phase 2/3 randomized trial
- Amit Saraf ORCID: orcid.org/0009-0007-7952-9212 1 na2 ,
- Rohan Gurjar ORCID: orcid.org/0000-0003-3495-2563 1 na2 ,
- Swarnendu Kaviraj 1 ,
- Aishwarya Kulkarni ORCID: orcid.org/0009-0001-3509-0398 1 ,
- Durgesh Kumar ORCID: orcid.org/0009-0001-7413-3987 1 ,
- Ruta Kulkarni 2 ,
- Rashmi Virkar ORCID: orcid.org/0000-0002-3764-9626 2 ,
- Jayashri Krishnan 3 ,
- Anjali Yadav 3 ,
- Ekta Baranwal 3 , 4 ,
- Ajay Singh ORCID: orcid.org/0009-0008-9619-2364 1 ,
- Arjun Raghuwanshi 1 ,
- Praveen Agarwal ORCID: orcid.org/0009-0005-4551-9118 1 ,
- Laxman Savergave 1 ,
- Sanjay Singh ORCID: orcid.org/0000-0003-4332-4606 1 &
the GEMCOVAC-OM Study Investigators
Nature Medicine ( 2024 ) Cite this article
1 Altmetric
Metrics details
- Randomized controlled trials
- RNA vaccines
Here we conducted a multicenter open-label, randomized phase 2 and 3 study to assess the safety and immunogenicity of a severe acute respiratory syndrome coronavirus 2 (SARS-CoV-2) Omicron-specific (BA.1/B.1.1.529), monovalent, thermostable, self-amplifying mRNA vaccine, GEMCOVAC-OM, when administered intradermally as a booster in healthy adults who had received two doses of BBV152 or ChAdOx1 nCoV-19. GEMCOVAC-OM was well tolerated with no related serious adverse events in both phase 2 and phase 3. In phase 2, the safety and immunogenicity of GEMCOVAC-OM was compared with our prototype mRNA vaccine GEMCOVAC-19 (D614G variant-specific) in 140 participants. At day 29 after vaccination, there was a significant rise in anti-spike (BA.1) IgG antibodies with GEMCOVAC-OM ( P < 0.0001) and GEMCOVAC-19 ( P < 0.0001). However, the IgG titers (primary endpoint) and seroconversion were higher with GEMCOVAC-OM ( P < 0.0001). In phase 3, GEMCOVAC-OM was compared with ChAdOx1 nCoV-19 in 3,140 participants (safety cohort), which included an immunogenicity cohort of 420 participants. At day 29, neutralizing antibody titers against the BA.1 variant of SARS-CoV-2 were significantly higher than baseline in the GEMCOVAC-OM arm ( P < 0.0001), but not in the ChAdOx1 nCoV-19 arm ( P = 0.1490). GEMCOVAC-OM was noninferior (primary endpoint) and superior to ChAdOx1 nCoV-19 in terms of neutralizing antibody titers and seroconversion rate (lower bound 95% confidence interval of least square geometric mean ratio >1 and difference in seroconversion >0% for superiority). At day 29, anti-spike IgG antibodies and seroconversion (secondary endpoints) were significantly higher with GEMCOVAC-OM ( P < 0.0001). These results demonstrate that GEMCOVAC-OM is safe and boosts immune responses against the B.1.1.529 variant. Clinical Trial Registry India identifier: CTRI/2022/10/046475 .
As of 3 March 2024, there have been 774,834,251 confirmed cases of COVID-19 with 7,037,007 deaths 1 . Vaccines designed for SARS-CoV-2 have been effective in mitigating the COVID-19 pandemic 2 .
Various platforms have been used to develop COVID-19 vaccines. These include inactivated whole virion, protein subunit and adenoviral vector platforms. However, one of the most prominent achievements during this pandemic has been the approval of messenger RNA-based vaccines for the first time for any disease.
mRNA vaccines have substantial advantages over the traditional vaccines. mRNA does not integrate into the host DNA and is noninfectious. Furthermore, mRNA vaccines are produced synthetically in a cell-free environment allowing a scalable, cost-effective and rapid production 3 . This makes the platform ideal to target emerging variants of concern, an important advantage given the rapidly evolving nature of SARS-CoV-2. Consequently, the first Omicron-specific adapted vaccines were mRNA based.
Notwithstanding these advantages, access to mRNA-based vaccines has been a challenge, especially for low- to middle-income countries (LMICs). The transport and storage of mRNA vaccines require a subzero temperature cold chain. Such infrastructure is not readily available in LMICs and is costly to implement.
The BA.1 (B.1.1.529) Omicron variant, first identified in Botswana, became dominant worldwide quickly and was seen to evade immunity acquired from vaccines that were designed against the ancestral strain 4 . An Omicron-adapted vaccine was needed to provide protection against this variant. To cater to this unmet need, we developed GEMCOVAC-OM, a monovalent, Omicron-specific (BA.1) booster mRNA vaccine for COVID-19 (ref. 5 ). It is distinct from the other two US Food and Drug Administration (FDA)-approved mRNA vaccines (from Pfizer-BioNTech and Moderna) in several ways. First, GEMCOVAC-OM is a self-amplifying mRNA (samRNA) vaccine, which can lower the dose for administration 6 . In contrast to the nonamplifying mRNA, the open reading frame of a samRNA encodes a replicase that is a complex of four nonstructural proteins (nsP1–nsP4). These nsPs interact to form an RNA-dependent RNA polymerase (RdRp) that drives the self-amplification of the mRNA inside the cell 7 . Second, GEMCOVAC-OM is lyophilized and stable at 2–8 °C for 12 months. Third, GEMCOVAC-OM is delivered intradermally through a needle-free injection system using a device called Tropis (PharmaJet).
In this Article, we describe the results of a phase 2 and 3 study, designed to assess the safety and immunogenicity of GEMCOVAC-OM as a heterologous booster in healthy adults (18 years of age and older). GEMCOVAC-OM has received an Emergency Use Authorization from the Central Licensing Authority in India on 19 June 2023. We present the immunogenicity and safety results from the phase 2 and 3 study.
GEMCOVAC-OM is a SARS-CoV-2 Omicron-specific (B.1.1.529), monovalent, thermostable, samRNA vaccine. Details on the self-amplifying nature of GEMCOVAC-OM and the methods used for its characterization and production can be found in Supplementary Information .
Study design
We conducted a multicenter, randomized phase 2 seamlessly followed by phase 3 study to assess the safety and immunogenicity of GEMCOVAC-OM in healthy adults who had received two doses of either BBV152 (COVAXIN) or ChAdOx1 nCoV-19 (COVISHIELD) as their primary vaccination at least 4 months before screening. In the phase 2 study, 140 participants were randomized to either GEMCOVAC-OM or the prototype vaccine GEMCOVAC-19, which was designed against the D614G variant of SARS-CoV-2. Primary endpoints of phase 2 were to compare the safety and anti-spike IgG antibodies between the two vaccinated arms at a prespecified interim analysis at day 29 post-vaccination. Secondary endpoints included comparison of seroconversion as assessed by a ≥2-fold rise in anti-spike IgG antibody titers from baseline, percentage neutralization by a surrogate neutralization (cPass) assay and cellular immune responses at day 29. Additionally, exploratory endpoints included comparison of anti-spike IgG antibodies, percentage neutralization by cPass assay and cellular immune responses at day 90.
Ideally, in phase 3, GEMCOVAC-OM should have been compared with an Omicron-specific mRNA vaccine. However, at the time of the study, neither mRNA nor Omicron-specific vaccines were approved or available in India. Hence, in phase 3, we compared GEMCOVAC-OM with ChAdOx1 nCoV-19, which was designed against the Wuhan strain and was approved for administration as a booster in India. The primary endpoint of the study was to demonstrate the noninferiority of GEMCOVAC-OM to ChAdOx1 nCoV-19 in terms of neutralizing antibody titers assessed by least square geometric mean ratio (LSGMR) and difference in seroconversion at day 29. Secondary endpoints included comparison of anti-spike IgG antibody titers, percentage neutralization by cPass assay, cellular immune responses at day 29 and safety for the duration of the study (day 180). Additionally, exploratory endpoints were comparison of humoral and cellular immune responses at day 90.
Participants
In phase 2, a total of 140 participants received GEMCOVAC-19 and GEMCOVAC-OM in a 1:1 ratio between 18 October 2022 and 20 October 2022. All 140 individuals completed the study up to the day 180 follow-up. In phase 3, a total of 3,140 participants were enrolled from 15 November 2022 to 24 November 2022; 3,000 and 140 participants were enrolled in the GEMCOVAC-OM and the ChAdOx1 nCoV-19 arms, respectively. Of these, three participants withdrew their consent before vaccination. After vaccination on day 1, it was found that 14 participants (9 in GEMCOVAC-OM arm and 5 in ChAdOx1 nCoV-19 arm) had already received a booster vaccine for COVID-19. This was a major protocol deviation and, hence, these participants were excluded from the safety and immunogenicity analysis. Additionally, in the GEMCOVAC-OM group, two participants withdrew consent, one individuals was lost to follow-up and one participant missed the day 29 visit. The day 29 visit was completed by 3,119 participants of which 404 (271 in the GEMCOVAC-OM arm and 133 in ChAdOx1 nCoV-19 arm) were included in the primary immunogenicity endpoint. Subsequently, in the GEMCOVAC-OM group, one individual withdrew consent, eight participants missed their day 90 visit, two participants were lost to follow-up and three exited the study. There was one protocol deviation where it was revealed that, for one participant, the time between the second dose of the primary vaccination and the booster vaccination was less than 4 months (exclusion criteria), which led to the discontinuation of the participant from the study. The last visit of day 180 was completed by 2,980 participants in the GEMCOVAC-OM group and 133 participants in the ChAdOx1 nCoV-19 group (Fig. 1 ).
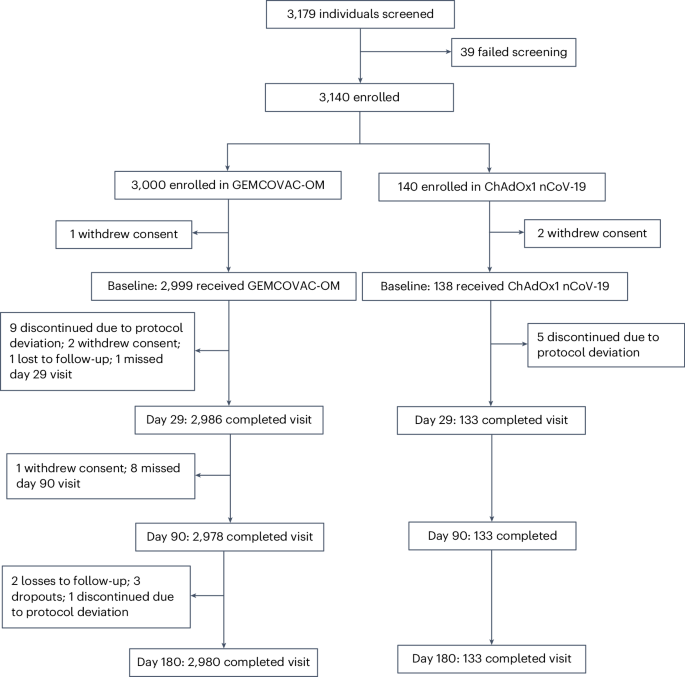
CONSORT diagram .
Baseline and demographic characteristics
The median age of participants was comparable in both phase 2 (GEMCOVAC-OM: 32 years and GEMCOVAC-19: 30 years) and phase 3 (32 years in both GEMCOVAC-OM and ChAdOx1 nCoV-19 arms). Of note, there were considerably more men in both phase 2 (87.1% in GEMCOVAC-OM and 91.4% in GEMCOVAC-19) and phase 3 (68.2% in GEMCOVAC-OM and 79.7% in ChAdOx1 nCoV-19). There was no notable difference in the mean weight and body mass index of participants in both phase 2 and phase 3 (Table 1 ).
Humoral Immunogenicity
All immunogenicity assessments were conducted against the BA.1 Omicron variant of SARS-CoV-2. Since none of the ancestral strains (Wuhan and D614G) was in circulation, immunogenicity against them was not assessed.
In phase 2, there was a statistically significant rise in the geometric mean titers (GMT) of anti-spike IgG antibodies from baseline (30,048, 95% confidence interval (CI) 23,910–37,763) to day 29 (244,440, 95% CI 229,122–260,782, P < 0.0001) with GEMCOVAC-OM (Extended Data Table 1 ). Similar increase in anti-spike IgG antibodies from baseline (35,676, 95% CI 29,401–43,289) to day 29 (75,683, 95% CI 61,687–92,853, P < 0.0001) was observed with GEMCOVAC-19. The geometric mean fold rise (GMFR, post-booster/prebooster vaccination) in anti-spike IgG antibodies for GEMCOVAC-OM and GEMCOVAC-19 was 8.13 and 2.12, respectively. The LSGMR of anti-spike IgG antibodies (GEMCOVAC-OM/GEMCOVAC-19) calculated using analysis of covariance (ANCOVA) was 3.40 (95% CI 2.79–4.13, P < 0.0001). At day 29, more participants (92.9%) in the GEMCOVAC-OM group achieved a ≥2-fold rise (seroresponse) in antibody titers as compared with participants (57.1%) in the GEMCOVAC-19 group. The seroresponse rate difference (secondary endpoint) calculated using the Miettinen–Nurminen method was 35.71 (95% CI 22.35–48.52, P < 0.0001). Furthermore, at day 90, anti-spike IgG GMT in both GEMCOVAC-OM and GEMCOVAC-19 groups was higher than baseline. However, these titers were higher with GEMCOVAC-OM compared with GEMCOVAC-19 (LSGMR: 3.31, 95% CI 2.72–4.02, P < 0.0001). Change in mean percentage neutralization (secondary endpoint), assessed by the surrogate neutralization assay (cPass), from baseline to day 29, was higher with GEMCOVAC-OM (19.3, standard error (s.e.) 1.40) compared with GEMCOVAC-19 (8.4, s.e. 1.40, P < 0.0001). Additionally, at day 90, the mean percentage neutralization for GEMCOVAC-OM and GEMCOVAC-19 was 96.3 (s.d. 8.35) and 90.0 (s.d. 21.11), respectively (Supplementary Table 3 ).
In phase 3, there was a statistically significant rise in the GMT of neutralizing antibodies (PRNT 50 ) against SARS-CoV-2 from baseline (623.9, 95% CI 533.3–729.9) to day 29 (1,099.9, 95% CI 1,000.0–1,209.9, P < 0.0001) with GEMCOVAC-OM. In contrast, no significant change in the GMT of neutralizing antibodies was observed with ChAdOx1 nCoV-19 from baseline (775.3, 95% CI 620.2–969.2) to day 29 (754.9, 95% CI 631.5–902.5, P = 0.1490; Fig. 2a ). GMFR in neutralizing antibodies for GEMCOVAC-OM and ChAdOx1 nCoV-19 were 1.76 and 0.97, respectively. LSGMR of the neutralizing antibodies for the treatment groups (GEMCOVAC-OM/ChAdOx1 nCoV-19) at day 29 was 1.58 (95% CI 1.36–1.84; Table 2 ). The lower bound 95% CI of LSGMR was above the prespecified criteria of noninferiority (>0.67). At day 29, more participants in the GEMCOVAC-OM arm (39.5%) showed a seroresponse (≥2-fold rise in PRNT 50 ) as compared with the participants in the ChAdOx1 nCoV-19 arm (19.5%; Table 2 ). The seroresponse rate difference between GEMCOVAC-OM and ChAdOx1 nCoV-19 was 19.93 (95% CI 10.57–28.43), which was statistically significant ( P < 0.0001). The lower bound 95% CI of the difference in seroconversion was above the predefined criteria for noninferiority (>−10%). Moreover, the lower bound 95% CI for LSGMR and the lower bound 95% CI of difference in seroconversion were above the superiority criteria of >1 and >0%, respectively. Furthermore, at day 90, GMT of neutralizing antibodies was higher with GEMCOVAC-OM (754.0, 95% CI 682.0–833.6) compared with ChAdOx1 nCoV-19 (383.1, 95% CI 319.3–459.6; Fig. 2a ). The GMFR from baseline with GEMCOVAC-OM (1.21) was numerically higher than ChAdOx1 nCoV-19 (0.49). LSGMR of the neutralizing antibodies at day 90 was 2.09 (95% CI 1.75–2.49, P < 0.0001; Table 2 ).
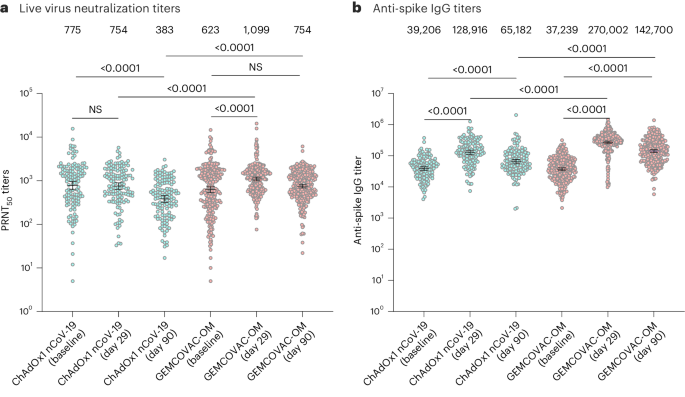
a , b , Humoral immune response with ChAdOx1 nCoV-19 ( n = 133) and GEMCOVAC-OM ( n = 271) assessed by PRNT 50 ( a ) and anti-spike IgG antibodies ( b ). The data are presented as geometric mean with 95% CI. LSGMR with 95% CI at day 29 and day 90 along with the P value was calculated using ANCOVA with baseline values as covariates. Change in titers from baseline to day 29 and day 90 was calculated by using a two-sided paired t -test or Wilcoxon signed-rank test based on normality. NS, not significant.
In phase 3, there was an increase in the anti-spike IgG GMT from baseline to day 29 in both vaccinated groups (Fig. 2b ). GMFR was numerically higher in GEMCOVAC-OM (7.25) compared with ChAdOx1 nCoV-19 (3.29). LSGMR of anti-spike IgG antibody titers (GEMCOVAC-OM/ChAdOx1 nCoV-19) at day 29 was 2.15 (95% CI 1.83–2.52, P < 0.0001). Seroresponse in terms of anti-spike IgG antibodies were higher with GEMCOVAC-OM (93.0%) compared with ChAdOx1 nCoV-19 (76.7%) with a difference of 16.30 (95% CI 9.02–24.64, P < 0.0001; Supplementary Table 6 ). Similarly, at day 90, anti-spike IgG GMT was significantly higher with GEMCOVAC-OM compared with ChAdOx1 nCoV-19 with a LSGMR of 2.23 (95% CI 1.87–2.66, P < 0.0001).
Mean percentage neutralization assessed by cPass assay was higher at day 29 (94.0%, s.d. 11.30) compared with baseline (68.1%, s.d. 27.07) with GEMCOVAC-OM. A similar increase was observed with ChAdOx1 nCoV-19 at day 29 (94.3%, s.d. 12.26) compared with baseline (68.6%, s.d. 26.10). There was no difference in the increase in the mean percentage neutralization between the two groups ( P = 0.8559). However, at day 90, mean percentage neutralization was numerically higher with GEMCOVAC-OM (91.7%, s.d. 11.45) compared with ChAdOx1 nCoV-19 (81.3%, s.d. 19.74; Supplementary Table 7 ).
We conducted a subgroup analysis of the humoral immunogenicity data based on primary vaccination in both phase 2 and phase 3 and observed that the differences in immune responses between GEMCOVAC-OM and the comparators were consistent. Additionally, we assessed the humoral response (PRNT and IgG titers) and safety of phase 3 using data disaggregated by sex. In both the vaccine arms, women had numerically higher neutralizing and anti-spike IgG antibody titers at baseline compared with men. We considered these baseline titers as covariates in the ANCOVA model that was used to assess differences in the humoral immunogenicity in male and female participants. In the ChAdOx1 nCoV-19 arm, there were no significant differences between the two sexes in the neutralizing and anti-spike IgG titers at day 29 and day 90. Similarly, in the GEMCOVAC-OM group, there were no significant difference in the neutralizing antibodies at day 29 and anti-spike IgG antibodies at days 29 and 90 between female and male participants. However, at day 90, neutralizing antibody titers were significantly higher in women compared with men who received GEMCOVAC-OM (LSGMR: 0.79, 95% CI 0.63–0.98, P = 0.0333; Supplementary Figs. 9 and 10 ).
Cell-mediated immunogenicity
Cellular responses against the B.1.1.529 (BA.1) variant were assessed by stimulating peripheral blood mononuclear cells (PBMCs) with an Omicron-specific peptide pool. Cellular immunogenicity assessed at day 29 was the secondary objective for both phase 2 and phase 3 part of the study. The cellular response at day 90 was a part of the exploratory endpoints.
In phase 2, the cellular immune response was assessed in 20% of the participants (14 in each arm). Lymphocyte counts were comparable in both the vaccinated groups (Supplementary Fig. 2 ). A statistically significant increase in IFNγ + CD4 + T cells from baseline to day 29 was observed in participants who received GEMCOVAC-19 ( P = 0.0076) and GEMCOVAC-OM ( P = 0.007). A similar increase was also observed in IL-2 + CD4 + T cells in participants who received GEMCOVAC-19 ( P = 0.0005) and GEMCOVAC-OM ( P < 0.0001). At day 29, IFNγ + CD4 + T cells and IL-2 + CD4 + T cells were comparable in GEMCOVAC-19 and GEMCOVAC-OM (Supplementary Fig. 3 ). In terms of CD8 + T cells, GEMCOVAC-19 and GEMCOVAC-OM showed a significant increase in IFNγ + CD8 + T cells ( P = 0.0004 and P = 0.0009 respectively) and IL-2 + CD8 + T cells ( P = 0.0002 and P = 0.0001, respectively) from baseline to day 29. Moreover, a significant increase in TNF + CD8 + T cells from baseline to day 29 was observed in GEMCOVAC-OM ( P = 0.002; Supplementary Fig. 4 ). Additionally, at day 90, GEMCOVAC-19 and GEMCOVAC-OM had significantly higher IFNγ + CD4 + T cells ( P = 0.001 and P = 0.0465, respectively) and IFNγ + CD8 + T cells ( P = 0.0002 and P = 0.0027, respectively) compared with baseline. TNF + CD8 + T cells were higher with GEMCOVAC-OM ( P = 0.0003) at day 90 compared with baseline. At day 90, TNF + CD4 + T cells ( P = 0.0055) and IL-2 + CD8 + T cells ( P = 0.0271) were higher with GEMCOVAC-OM compared with GEMCOVAC-19. Spike-specific T helper 2 (T H 2) cell cytokine (IL-4 and IL-13) expression in the T cells from both the vaccinated cohorts was significantly lower at day 29 and day 90 compared to baseline (Supplementary Fig. 5 ).
In the phase 3 study, cellular immunity was assessed in subset of participants (~25% from each arm). Hence, a total of 106 samples (GEMCOVAC-OM: 71 and ChAdOx1 nCoV-19: 35) were included in the analysis. Lymphocyte counts were comparable across both the vaccinated groups (Extended Data Fig. 1a–c ). There was a statistically significant increase in IFNγ + CD4 + T cells from participants vaccinated with ChAdOx1 nCoV-19 ( P < 0.0001) and in IL-2 + CD4 + T cells from recipients of GEMCOVAC-OM ( P < 0.0001) from baseline to day 29. However, at day 29, there was no significant difference in the IFNγ + CD4 + T cells in both the groups. GEMCOVAC-OM samples showed significantly higher TNF + CD4 + and IL-2 + CD4 + T cells compared with ChAdOx1 nCoV-19 ( P < 0.0001; Fig. 3a–c ) at day 29. With regard to cytotoxic T cells, a significant rise in IFNγ + CD8 + T cells was seen with both ChAdOx1 nCoV-19 ( P < 0.0001) and GEMCOVAC-OM ( P = 0.0079). ChAdOx1 nCoV-19 samples showed a significant increase in TNF + CD8 + T cells from baseline to day 29. However, at day 29, there was no difference in TNF + CD8 + T cells in GEMCOVAC-OM and ChAdOx1 nCoV-19. GEMCOVAC-OM samples had a significantly higher percentage of IL-2 + CD8 + T cells at day 29 compared with baseline ( P < 0.0001). The percentage of IL-2 + CD8 + T cells was also higher than ChAdOx1 nCoV-19 samples at day 29 ( P < 0.0001; Fig. 3d–f ). Additionally, at day 90, the percentage of IFNγ + CD4 + , IFNγ + CD8 + and TNF + CD8 + T cells were higher in ChAdOx1 nCoV-19, whereas IFNγ + CD8 + and TNF + CD8 + T cells were higher in GEMCOVAC-OM compared with baseline. GEMCOVAC-OM showed significantly higher Omicron B.1.1.529-reactive TNF + CD4 + ( P < 0.001), IL-2 + CD4 + ( P < 0.0001) and IL-2 + CD8 + ( P < 0.0001) T cells compared with ChAdOx1 nCoV-19 (Fig. 3a–f ).
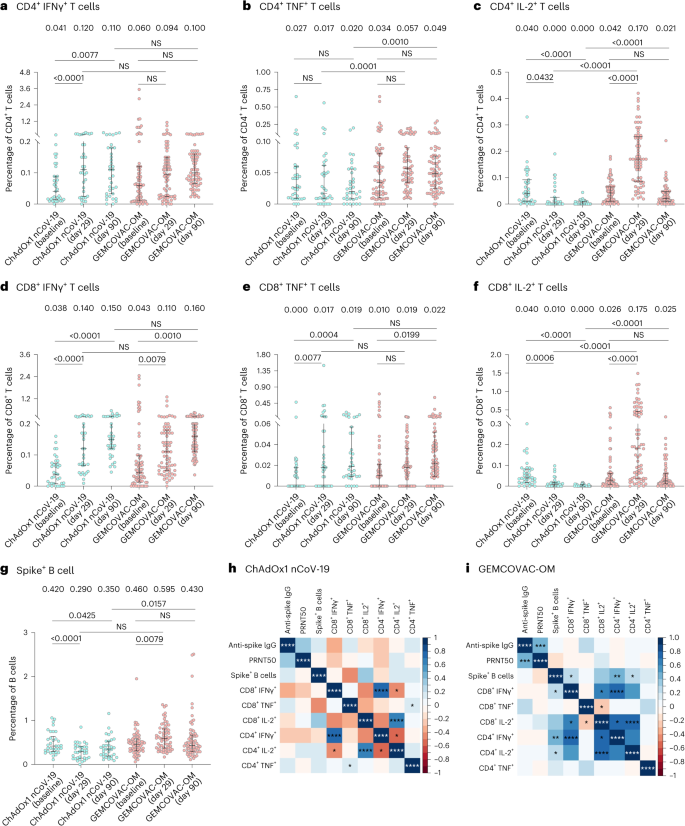
a – f , For T cell response analysis, PBMCs from ChAdOx1 nCoV-19 ( n = 35) and GEMCOVAC-OM ( n = 71) cohorts were stimulated with Omicron spike-specific PepTivator. Change in CD4 + T cells expressing IFNγ ( a ), TNF ( b ) and IL-2 ( c ) and CD8 + T cells expressing IFNγ ( d ), TNF ( e ) and IL-2 ( f ). g , Omicron B.1.1.529 spike + CD19 + CD20 + B cells in ChAdOx1 nCoV-19 ( n = 34) and GEMCOVAC-OM ( n = 67) cohorts. The data are presented as median with interquartile range. Change in cytokine expression from baseline to day 29 and day 90 was assessed using a two-sided paired t -test or Wilcoxon signed-rank test based on normality. Expression at day 29 and day 90 in both the groups was compared using a two-sided t -test or Wilcoxon rank sum test based on normality. h , i , Spearman correlation between humoral and cellular responses in ChAdOx1 nCoV-19 ( h ) and GEMCOVAC-OM ( i ) cohorts measured at day 29. In corrplot, the blue boxes represent positive correlations and the red boxes represents negative correlation. Significant correlations were represented as an asterisk in the boxes. * P < 0.05, ** P < 0.01, *** P < 0.001 and **** P < 0.0001.
B.1.1.529 spike-specific T H 2 cell cytokine expressions (IL-4 and IL-13) in T cells in both phase 2 and phase 3 study were lower at day 29 and day 90 compared with their baselines (Extended Data Fig. 2 ).
B.1.1.529 spike-specific B cells following booster dose were also quantified. Total CD19 + CD20 + B cells were comparable in both the arms at day 29 (Extended Data Fig. 1d ). GEMCOVAC-OM displayed a significant increase in B.1.1.529 spike-specific B cells compared with baseline ( P = 0.0013) and ChAdOx1 nCoV-19 at day 29 ( P < 0.0001; Fig. 3g ). However, at day 90, Omicron B.1.1.529-specific B cells in the GEMCOVAC-OM study group were similar to baseline level but significantly higher than ChAdOx1 nCoV-19 ( P = 0.0157).
A post-hoc Spearman correlation analysis was conducted to elucidate the relationships between immunogenic responses measured on day 29 with GEMCOVAC-OM and ChAdOx1 nCoV-19 (Fig. 3h,i ). A significant positive correlation between anti-spike IgG (binding) antibody titers and neutralizing antibody titers was observed with GEMCOVAC-OM, but not with ChAdOx1 nCoV-19. In both, GEMCOVAC-OM and ChAdOx1 nCoV-19 cohorts, a strong positive correlation was seen between the IFNγ + CD4 + and IFNγ + CD8 + T cells ( P < 0.0001). ChAdOx1 nCoV-19 also showed a significant positive correlation between TNF-expressing CD4 + and CD8 + T cells ( P < 0.05) as well as IL-2 + CD4 + and IL-2 + CD8 + T cells ( P < 0.0001). Specifically, a noteworthy finding was the significant positive correlation between spike + B cells and IFNγ + CD4 + , IFNγ + CD8 + and IL-2 + CD4 + T cells with GEMCOVAC-OM. Significant positive correlations between IL-2-expressing CD4 + and CD8 + T cells ( P < 0.0001) as well as IFNγ + CD8 + and IL-2 + CD8 + T cells ( P < 0.05) were also observed with GEMCOVAC-OM.
Safety was the co-primary endpoint in phase 2 and secondary endpoint in phase 3. As this was a seamless study, the adverse event (AE) data 7 days post vaccination in phase 2 was analyzed and presented to the Data Safety Monitoring Board (DSMB). After approval by the DSMB, phase 3 was initiated. Safety data of both phase 2 and phase 3 study were reviewed periodically by DSMB.
In phase 2, there were a total of 14 AEs of which 5 occurred in participants who received GEMCOVAC-OM and 9 in those who received GEMCOVAC-19 (Supplementary Table 4 ). No unsolicited events or serious AEs were reported till the end of the study.
In phase 3, no substantial difference in the AEs was observed in participants who received GEMCOVAC-OM and ChAdOx1 nCoV-19 (Fig. 4 ). In the GEMCOVAC-OM arm, 602 participants (20.1%) reported at least one AE compared with 33 (24.8%) in the ChAdOx1 nCoV-19 arm. Most of the reported AEs were mild. In the GEMCOVAC-OM arm, the most common local solicited AE was injection site pain (9.2%), followed by erythema (2.8%), pruritus (2.0%) and swelling (1.6%). The most common systemic solicited AE was fever (6.6%), followed by headache (4.5%), myalgia (2.1%), fatigue (1.7%), arthralgia (0.7%), chills (0.7%) and nausea (0.2%). A total 1.40% of participants in GEMCOVAC-OM and 2.26% in ChAdOx1 nCoV-19 reported unsolicited events until day 180. Three participants from the GEMCOVAC-OM arm reported serious AEs of omphalitis, spontaneous abortion and pulmonary tuberculosis, while one participant who received ChAdOx1 nCoV-19 suffered a ligament rupture. These events were determined unlikely due to vaccination by the study site investigators. There was no death in the study. In phase 3, there was no notable difference in the AEs reported between men and women who received ChAdOx1 nCoV-19. However, in the GEMCOVAC-OM group, more women than men reported local (unadjusted odds ratio (OR) 0.78, 95% CI 0.64–0.96) and systemic (OR 0.73, 95% CI 0.60–0.90) AEs (Supplementary Table 11 ).
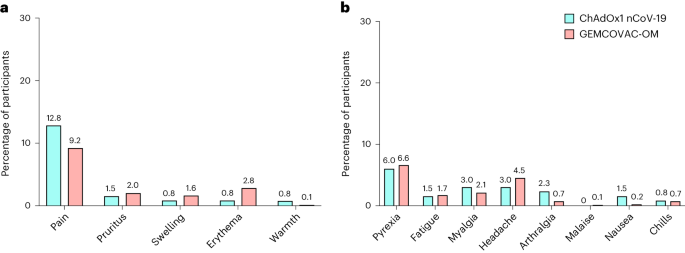
a , b , Percentage of participants in whom local ( a ) and systemic ( b ) solicited AEs were observed in the first 7 days after a booster dose of ChAdOx1 nCoV-19 ( n = 133) and GEMCOVAC-OM ( n = 2,990).
We report the humoral and cellular immunogenicity as well as the safety results of the phase 2 and 3 study of GEMCOVAC-OM versus GEMCOVAC-19 and ChAdOx1 nCoV-19, respectively, administered as a heterologous booster in participants who received either BBV152 or ChAdOx1 nCoV-19 as their primary vaccination. GEMCOVAC-OM was found to be safe and well tolerated and generated significant humoral as well as cellular immune responses against the B.1.1.529 variant. GEMCOVAC-OM is thermostable and the first samRNA vaccine to receive an Emergency Use Authorization. Moreover, it is the only Omicron-specific vaccine that is approved in India.
Recently, a samRNA vaccine (ARCT-154) by Arcturus Therapeutics and CSL received a full approval as a COVID-19 booster in Japan 8 . This approval was based on a phase 3 study in which ARCT-154 administered at 5 μg dose resulted in higher immune responses than 30 μg Comirnaty (Pfizer/BioNTech) 9 . Several companies across the globe 10 , 11 are developing samRNA vaccine candidates against COVID-19 that are already in clinical trials.
In phase 2 and phase 3, the safety and tolerability of GEMCOVAC-OM was comparable to GEMCOVAC-19 and ChAdOx1 nCoV-19. Most of the AEs were mild to moderate and resolved on their own. No vaccine-related serious AEs or deaths were reported. mRNA vaccines have been associated with myocarditis, and this AE was included in the study trial as an AE of special interest. None of the participants reported any signs or symptoms of myocarditis that would warrant further investigation, which is expected given the incidence of 1–5 cases per 100,000 in the general population 12 .
The Omicron variant (B.1.1.529/BA.1), first detected in Botswana, harbors up to 59 mutations, of which 34 occur within the spike protein 13 . These mutations are associated with escape from natural and vaccine-induced neutralizing antibodies 14 , 15 and increased affinity to angiotensin-converting enzyme 2 (ACE2), thereby increasing infectivity 16 . The mutations have been shown to reduce the effectiveness of vaccines that were developed against the Wuhan strain resulting in breakthrough infections and hospitalizations 17 , 18 , 19 . Based on the WHO Technical Advisory Group on COVID-19 Vaccine Composition (TAG-CO-VAC) guidelines, Moderna 20 and Pfizer 21 updated their vaccines to a bivalent booster containing mRNAs targeting the Wuhan as well as the Omicron variant to provide broader protection to vaccine recipients. However, the benefit of adding the Wuhan-specific mRNA was limited due to immune imprinting (antigenic sin) 22 . People who were immunized with the bivalent vaccines were already primed to respond to Wuhan and generated antibodies against Wuhan even when boosted with the Omicron-specific vaccine. Subsequently, TAG-CO-VAC upgraded its recommendations to develop a monovalent booster vaccine for COVID-19 (ref. 23 ). In line with this guideline, GEMCOVAC-OM is a monovalent booster vaccine against the BA.1 variant.
Neutralizing antibody response is considered to be a surrogate for efficacy against severe disease 24 and has been used in immunobridging studies, especially for variant-updated COVID-19 vaccines 20 . Live virus neutralization assays are considered to be the gold standard for assessing immune responses 25 . ChAdOx1 nCoV-19, the comparator vaccine in phase 3, was designed against the Wuhan strain of SARS-CoV-2 and is expected to have lower immunogenic responses against Omicron than the Omicron-specific (BA.1) vaccine GEMCOVAC-OM. Consequently, GEMCOVAC-OM showed a 1.76-fold increase in neutralizing antibody titers against Omicron BA.1, while no significant change in the neutralization titers was observed with ChAdOx1 nCoV-19, when administered as a booster. At day 29, the lower bound 95% CI of LSGMR and the lower bound of 95% CI of seroconversion difference between GEMCOVAC-OM and ChAdOx1 nCoV-19 was above the predefined margins of noninferiority (>0.67 and >−10%, respectively) as well as superiority (>1 and >0%, respectively) 26 . Neutralizing antibody titers with GEMCOVAC-OM were 1.58 times that of ChAdOx1 nCoV-19 at day 29. These results are similar to the neutralizing antibody titer ratios observed with the other approved BA.1-adapted mRNA vaccines when compared with their prototype vaccines. At 28 days after booster vaccination, neutralizing antibody titers with Moderna’s mRNA-1 273.214 (25 μg each of ancestral Wuhan-Hu-1 and Omicron B.1.1.529 (BA.1) spike mRNAs) were 1.75 times those elicited with mRNA.1273 (ref. 20 ). Similarly, at 1 month after booster vaccination, neutralizing antibody titers with Pfizer/BioNTech’s 30 μg (15 μg each of BNT162b2 and BA.1) were 1.56 times those induced by BNT162b2 (ref. 21 ).
Furthermore, at day 90, the neutralizing antibodies further dropped in the ChAdOx1 nCoV-19 group while the titers in the GEMCOVAC-OM group returned to levels close to the baseline (GMFR 1.21). Neutralizing antibody titers with GEMCOVAC-OM were significantly higher than with ChAdOx1 nCoV-19 at day 90 (LSGMR 2.09). It is important to note that the baseline neutralizing titers in this study were already high and could potentially impact the boosting ability of the vaccines. Nonetheless, GEMCOVAC-OM elicits higher neutralization of BA.1 for at least 3 months compared with ChAdOx1 nCoV-19.
In phase 2 and phase 3, anti-spike IgG antibodies measured using enzyme-linked immunosorbent assay (ELISA) against the BA.1 variant of SARS-CoV-2 were significantly higher with GEMCOVAC-OM compared with GEMCOVAC-19 and ChAdOx1 nCoV-19 at day 29 and day 90. This difference can be attributed to the fact that GEMCOVAC-19 and ChAdOx1 nCoV-19 are designed against the D614G and Wuhan variants while GEMCOVAC-OM is designed against BA.1.
Percentage neutralization, when assessed with a surrogate virus neutralization assay (cPass) in phase 3, showed no difference in GEMCOVAC-OM and ChAdOx1 nCoV-19 arms at day 29. In both arms, the percentage neutralization was >90%, which is representative of high neutralization activity. These results are in contrast with the neutralization assessed using PRNT 50 assay and may be due to differences in the assays. cPass is a semi-quantitative assay and has an upper limit cutoff of 100%. Differences in the vaccines beyond the cutoff cannot be observed. Importantly, live virus neutralization is considered to be the gold standard of immunogenicity assessment and a correlate of protection.
Omicron variants, although more infective, are associated with lower rates of hospitalization and death compared with previous SARS-CoV-2 strains. Studies have shown that the intrinsic severity of the Omicron variant is similar to that of its predecessors and cross-reactive protection from vaccination and/or infection plays an important role in reducing the severity of Omicron-associated COVID-19 (ref. 27 ). A study published by Naranbhai et al. 28 showed that T cell cross-reactivity to the SARS-CoV-2 Omicron variant remained intact in most of the previously infected and vaccinated individuals. However, a subset of individuals (approximately 21%) exhibited a more than 50% decrease in T cell reactivity to the Omicron spike 28 . We observed similar findings in which GEMCOVAC-19 and ChAdOx1 nCoV-19 showed cross-reactive cellular responses against Omicron. However, the cellular responses with the Omicron-specific GEMCOVAC-OM were higher. In phase 2, GEMCOVAC-19 exhibited cross-reactive IFNγ + T cells against the BA.1 variant. However, at day 90, the TNF + CD4 + and IL-2 + CD8 + T cell responses were higher in GEMCOVAC-OM compared with GEMCOVAC-19. Similarly, in phase 3, GEMCOVAC-OM showed an increase in IFNγ + CD8 + T cells from baseline to day 29 and a significantly higher expression of TNF + CD4 + , IL-2 + CD4 + and IL-2 + CD8 + T cells at day 29 compared with ChAdOx1 nCoV-19. Recent findings have shown that vaccine-induced CD8 + T cell responses are important for long-term immunity and should also be considered as a correlate of protection 29 , 30 . Both IFNγ and TNF play crucial roles in controlling intracellular pathogen infections. It is worth noting that IFNγ co-operates with other T H 1 cell cytokines and acts synergistically to enhance their ability to eliminate pathogens 31 , 32 . IL-2 serves various functions in the development of effector and memory CD8 + T cell responses. Kahan et al. 33 have demonstrated that a specific subset of CD8 + T cells, capable of intrinsic IL-2 expression, exhibit stem-like characteristics, display a memory phenotype, can withstand exhaustion and effectively regulate chronic viral infections 33 . Additionally, at day 90, GEMCOVAC-OM showed significantly higher TNF + CD4 + T cells and IL-2 + CD8 + T cells compared with GEMCOVAC-19 (phase 2) and higher TNF + CD4 + , IL-2 + CD4 + and IL-2 + CD8 + T cells compared with ChAdOx1 nCoV-19 (phase 3), indicating a durable T cell protection with BA.1-specific vaccine.
In addition to T cell responses, B cell responses play an important role in generating durable immunity. T H 1 and T H 2 cell responses support B cell activation and differentiation for antigen-specific antibody production and long-term memory development through germinal cell responses 34 , 35 . In our study, we observed a significant increase in the Omicron-specific B cell population at day 29 following vaccination. This response was not observed with ChAdOx1 nCoV-19.
In adults, women have shown to mount higher immune responses than men and are more likely to report adverse reactions 36 . We conducted a sex-disaggregated analysis on the humoral immunogenicity and safety data from phase 3. There were no significant differences in the neutralizing antibodies and anti-spike IgG titers between men and women who received ChAdOx1 nCoV-19 at day 29 and day 90. Additionally, no difference in AEs was observed. These findings contrast with sex-disaggregated data published by Marchevsky et al. 37 where a small, statistically significant difference was found in the anti-spike IgG titers, with higher titers in female participants and there were twice as many systemic reactions reported by women. However, it is important to note that there were many differences in the study design and the populations (sample size, primary versus booster vaccination, and ethnicity) assessed in these two studies that can have a substantial impact on outcomes. There were no significant differences in the neutralizing and anti-spike IgG titers between men and women receiving GEMCOVAC-OM, except at day 29, where neutralizing titers were significantly higher in women. Interestingly, female participants in the GEMCOVAC-OM group reported significantly higher local and systemic AEs. These findings are in line with the real-world evidence on COVID-19 mRNA vaccines (BNT162b2, mRNA-1273) which describe higher reactogenicity in females compared with males 38 , 39 .
The study has several limitations. (1) The study participants were predominantly male, and as such, the results may be more representatives of men than women. We have conducted a sex-disaggregated analysis; however, the analysis was not powered. (2) Ideally, GEMCOVAC-OM should have been assessed in a clinical trial using another Omicron-specific mRNA vaccine as a comparator. However, the mRNA vaccines from Moderna and Pfizer were not approved or available in India due to which ChAdOx1 nCoV-19, which was designed against the ancestral spike (Wuhan), was used as a comparator. This makes it difficult to accurately compare and characterize GEMCOVAC-OM. (3) Blinding of participants in the study was not possible due to the different mechanisms of delivery, which has the potential to introduce a bias in the safety assessment. (4) Given the multiple COVID-19 waves and asymptomatic infections, accurate data on past COVID-19 infections could not be obtained. This makes analysis of the impact of previous infection on the safety and immunogenicity of the vaccine difficult. (5) At the time of the study, booster vaccines were approved in India and, therefore, using a placebo as an arm in the clinical trial would have been unethical. An efficacy study was not possible and, hence, an immunobridging approach was used.
The platform used to develop GEMCOVAC-OM has certain advantages over the other mRNA vaccines approved for COVID-19. GEMCOVAC-OM is administered intradermally using a needle-free injection system called Tropis. The dermis has a rich network of dendritic cells, macrophages and T cells 40 . Vaccination into the dermis provides a more potent and broader immunogenic response than vaccinating into the muscle 24 . Additionally, the absence of a needle obviates challenges of need for sharps disposal, needle-stick injuries, cross-contamination and needle phobia. These advantages have translated into the field where vaccinators and caregivers expressed a preference for Tropis due to the ease of use, appearance, response to vaccination and increased coverage 41 . The device has been well characterized and approved by numerous regulatory bodies including the World Health Organization. Each Tropis device can inject up to 20,000 vaccines, and the additional cost of the device is negligible and outweighed by advantages. Thermostability is another critical factor in ensuring equitable access to effective mRNA vaccines in LMICs 42 . The degradation of mRNA in the presence of water, attributed to oxidation and hydrolysis 43 , required mRNA vaccines to be stored and transported at subzero temperatures. This makes the vaccine inaccessible to LMICs due to lack of infrastructure and the cost of establishing subzero cold chains. GEMCOVAC-OM is a lyophilized vaccine and can be transported and stored at 2–8 °C for 12 months, facilitating democratization of access to vaccine. Lastly, the samRNA platform used for GEMCOVAC-OM offers a promising avenue for effective and safe immunization against infectious diseases. samRNA vaccines can induce potent immune responses at lower doses due to their ability to amplify antigen production within the body, potentially reducing the risk of adverse reactions 6 , 44 .
In summary, these results show that GEMCOVAC-OM is safe and generates immunogenic responses when administered as a booster. This self-amplifying, thermostable mRNA platform delivered intradermally provides a framework for next-generation vaccines that can improve accessibility and global equity.
This was a prospective, multicenter, open-label, randomized phase 2 seamlessly followed by a phase 3 study to evaluate the safety, tolerability and immunogenicity of GEMCOVAC-OM as a booster in participants 18 years of age and older. In this seamless study, phase 2 safety data till day 7 were analyzed and presented to an independent DSMB. The DSMB evaluated these data and provided their approval to initiate the phase 3 part of the study. The phase 3 study was conducted at 20 hospitals in 13 cities across India in compliance with the principles defined in the Declaration of Helsinki, International Conference for Harmonisation Good Clinical Practice Guideline. The study protocol was approved by the local ethics committee at each study site and Central Drugs Standard Control Organisation, the central licensing authority in India. This clinical trial is registered with the Clinical Trial Registry India, CTRI/2022/10/046475. Details on the sites and Ethics Committees can be found in Supplementary Information .
An interim analysis was planned at day 29 of phase 3 where the immunogenicity and safety of the participants was assessed and presented to the Central Drugs Standard Control Organisation for Emergency Use Authorization.
In phase 2, the safety and immunogenicity of GEMCOVAC-OM as a booster was compared with the prototype vaccine GEMCOVAC-19 designed against the spike protein of the D614G strain of SARS-CoV-2 ( n = 140). Participants were randomized to receive the vaccines in a 1:1 ratio.
The phase 3 study comprised a safety and an immunogenicity cohort. The safety cohort consisted of 3,140 participants of whom 3,000 were enrolled into the GEMCOVAC-OM arm and 140 were enrolled into the ChAdOx1 nCoV-19 arm. Within the safety cohort, the immunogenicity cohort consisted of 420 participants of whom 280 were enrolled into the GEMCOVAC-OM arm and 140 were enrolled into the ChAdOx1 nCoV-19 arm. Participants were healthy adults (male or female participants reported by self), 18 years of age or older, who have received two doses of either BBV152 or ChAdOx1 nCoV-19, 4 months before the screening visit. Additionally, the participants should have had no known COVID-19 infection at least 3 months before the screening visit. Key exclusion criteria included pregnant or lactating mothers, individuals with illnesses that in the opinion of the investigator may affect safety, and the immunocompromised. Detailed inclusion and exclusion criteria are provided in the protocol ( Supplementary Information ). Participants were screened on the basis of medical history, vital signs and physical examination before enrollment. Eligible participants provided signed informed consent forms at enrollment. Participants were compensated at every visit for their time and cost of travel.
Randomization and masking
Participants who met the inclusion criteria and successfully completed all screening procedures were randomized in the study by using the interactive web response system (IWRS). Unique randomization codes were assigned to the participants and remained unchanged until the completion of the trial. The randomization codes were generated through Proc Plan using SAS version 9.4 or higher (SAS Institute) by an independent biostatistician. The final randomization list was filed securely by the independent biostatistician and accessible to authorized persons only. Participants were enrolled by investigators with the help of the IWRS.
In phase 3, consecutive 420 in the immunogenicity cohort were randomized in a 2:1 ratio into GEMCOVAC-OM and ChAdOx1 nCoV-19 by stratified block randomization through the IWRS. A randomization code was assigned to each participant in sequence in the order of enrollment, and then the participants received the investigational products labeled with the same code. This was an open-label study, and no masking was performed.
GEMCOVAC-OM consists of an in vitro transcribed mRNA encoding for the spike protein of the Omicron variant of the SARS-CoV-2 virus and cationic lipid nano-emulsion in a buffer containing 10% sucrose in 10 mM sodium citrate, pH 6.5 (ref. 45 ). The complete antigenic sequence that was used has been published in the DDBJ database (accession no. LC769018). GEMCOVAC-OM, 10 µg in 0.1 ml, was administered intradermally using a Tropis needle-free injection system (PharmaJet). More information on the vaccine, mRNA platform and development can be found in Supplementary Information .
ChAdOx1 nCoV-19 (COVISHIELD), the comparator vaccine in phase 3, consisted of Corona Virus Vaccine (Recombinant) 5 × 10 10 viral particles. This vaccine is based on recombinant, replication-deficient chimpanzee adenovirus vector encoding the SARS-CoV-2 spike glycoprotein, produced in genetically modified human embryonic kidney 293 cells. ChAdOx1 nCoV-19 was administered intramuscularly.
GEMCOVAC-19 consists of an in vitro transcribed mRNA encoding for the spike protein of the D614G variant of the SARS-CoV-2 virus and cationic lipid nano-emulsion in a buffer containing 10% sucrose in 10 mM sodium citrate, pH 6.5. The complete antigenic sequence that was used has been published in the DDBJ database (accession no. LC776732.1). GEMCOVAC-19, 10 µg in 0.5 ml, was administered intramuscularly.
The participants were screened on visit 1 (day 1), which included a validated reverse transcription polymerase chain reaction (RT–PCR) for SARS-CoV-2. Regardless of the outcome of the RT–PCR, the participants who fit the inclusion criteria were enrolled and the vaccine was administered on the same day. Those found to be RT–PCR positive would be excluded from the immunogenicity analysis to avoid confounding. Importantly, during the trial, in India, a third dose of BBV152 or ChAdOx1 nCoV-19 (precautionary dose) was approved for participants who had received primary doses of BBV152 or ChAdOx1 nCoV-19, respectively. However, individuals who had taken two doses of BBV152 as their primary vaccination were not eligible for a third dose of ChAdOx1 nCoV-19. Keeping in line with these vaccination guidelines, participants with ChAdOx1 nCoV-19 as their primary vaccination were randomized to get ChAdOx1 nCoV-19 or GEMCOVAC-OM, whereas participants with BBV152 as their primary vaccination received GEMCOVAC-OM only in the clinical trial.
Participants were provided an e-diary or a paper diary to record the solicited AEs till day 7 and unsolicited AEs as well as concomitant medication taken, if any, till the end of the study. A telephone call was placed to all the participants at day 7 to record any additional AEs, if any. Participants visited the study site for visit 2 (day 29 + 7), visit 3 (day 90 + 14) and visit 4 (day 180 + 14). Blood for assessing immunogenicity was drawn at visit 1 before vaccination (baseline), day 29 and day 90. Safety was assessed throughout the duration of the study.
In phase 2, the primary endpoints were to compare the safety and anti-spike IgG antibodies between the two vaccinated arms at day 29. Secondary endpoints included comparison of seroconversion as assessed by ≥2-fold rise in anti-spike IgG antibody titers from baseline, percentage neutralization by a surrogate neutralization (cPass) assay and cellular immune responses at day 29. Exploratory endpoints included comparison of anti-spike IgG antibodies, percentage neutralization by cPass assay and cellular immune responses at day 90.
In phase 3, the primary endpoint was the demonstration of noninferiority of neutralizing antibody GMT assessed by a plaque reduction neutralization test (PRNT 50 ) assay in terms of LSGMR at day 29 and difference in seroconversion (≥2-fold rise in antibody titers at day 29 from baseline) between GEMCOVAC-OM and ChAdOx1 nCoV-19. Secondary endpoints included comparison of safety, LSGMR and seroconversion in terms of anti-spike IgG antibody titers, percentage neutralization by a surrogate virus neutralization assay (cPass assay, GenScript) and cell-mediated immunity assessment by intracellular cytokine expression at day 29. Exploratory endpoints included humoral and cellular immune response assessment at day 90.
Immunogenicity assessment
Although the trial was open-label, laboratory analysis was conducted in a blinded manner. Measurements were taken from distinct samples. Information on the materials used is provided in detail in Supplementary Information .
Neutralizing antibody titers were assessed by the PRNT 50 assay at the Interactive Research School for Health Affairs (IRSHA, Bharati Vidyapeeth, Deemed to be University, Pune) that was previously developed 46 and then optimized for the BA.1 Omicron variant of SARS-CoV-2 (SARS-CoV-2-IND/0005/2022; B.1.1.529.1 lineage). In brief, Vero E6 cells were initially seeded at a density of 1 × 10 5 cells ml −1 in 24-well plates using Minimum Essential Medium (MEM) containing 10% fetal bovine serum (FBS) and antibiotics and allowed to incubate overnight at 37 °C with 5% CO 2 . Serum samples, initially diluted at 1:5 ratios, were subjected to heat inactivation for 30 min at 56 °C. Subsequently, a fourfold serial dilution was executed, and these serum dilutions were mixed in equal proportions with the SARS-CoV-2 virus with a titer ranging from 600 to 1,000 plaque-forming units (pfu) per milliliter. The serum–virus mix was then incubated for 1 h at 37 °C within a humidified incubator with 5% CO 2 . Following incubation, 100 μl of the resultant mixture was introduced into duplicate wells of the seeded 24-well plate and subjected to an additional 3-h incubation at 37 °C in a humidified incubator with 5% CO 2 . Then, 1 ml of an overlay medium, constituting MEM, Aquacide-II, 2% FBS and antibiotics, was added to the Vero cell monolayer. Plates were then incubated for 6 days at 37 °C within a humidified incubator with 5% CO 2 . At the end of this incubation period, the overlay medium was removed, and cells were fixed through the application of 3.7% formaldehyde. After washing with phosphate-buffered saline, cells were stained using 1% crystal violet. Plates were washed once more and air-dried. Viral plaques were counted using the C.T.L. ImmunoSpot platform. PRNT 50 titers were determined using standard logistic regression model. Neutralization was also assessed using a semi-quantitative surrogate virus neutralization assay (cPass, GenScript) 47 for the BA.1 variant.
Anti-IgG responses against the spike glycoprotein of B.1.1.529 Omicron variant of SARS-CoV-2 was assessed by an in-house developed indirect ELISA. In brief, 96-well ELISA plates (Nunc Maxisorp) coated with spike protein (full length from Sino Biologicals, 40589-V08H26) were washed thrice with phosphate-buffered saline-Tween (PBS-T). Plates were blocked with 3% nonfat dried milk. Diluted sera samples were added to the blocked plates and incubated at room temperature for 2 h. Plates were then washed thrice with PBS-T and incubated at room temperature with detection antibody (1:5,000), anti-human IgG (Fc region specific from Sigma A0170) for 1 h at room temperature. After secondary antibody incubation, plates were washed thrice with PBS-T before addition of TMB substrate. Color development was quenched with 3 M HCl after 20 min of incubation at room temperature. Plates were read at 450 nm using a plate reader. The assay background was calculated from the 10 s.d. added to the average of the readouts where there was no sample but diluent in the wells. For all samples, IgG titers were an interpolation of previously calculated assay background in 5 parameter logistic fit of sample dilution versus absorbance.
PBMCs were isolated using BD Vacutainer CPT with sodium citrate tubes following the manufacturer’s guidelines and subsequently cryopreserved in liquid nitrogen. For immune-phenotyping purposes, frozen PBMCs were thawed and allowed to rest in complete RPMI 1640 culture medium (CRPMI) supplemented with 10% FBS, 100 U ml −1 penicillin and 0.1 mg ml −1 streptomycin (1× pen-strep) for 18–22 h. Gating strategies for both T cell and B cell experiments are given in Extended Data Fig. 3 . In T cell response analysis, intracellular cytokine staining (ICS) was performed using 0.5 million PBMCs in 100 µl CRPMI medium per well in a V-bottom plate. These cells were stimulated with a 1 µg ml −1 epitope mapping 15-mer peptide pool derived from the Omicron B.1.1.529/BA.1 spike glycoprotein peptides, specifically the PepTivator SARS-CoV-2 Prot S B.1.1.529/BA.1 Mutation Pool. Stimulation was carried out in the presence of 1 µg ml −1 BD FastImmune (anti-CD28/49d antibody) 48 for 6 h, with the addition of 1 µl of Brefeldin-A during the final 4 h of stimulation. After stimulation, PBMCs were washed and subjected to surface and ICS staining using antibodies targeting CD3 PE-Cy7 (BD 557851, clone SK7, 1:20), CD4 BV480 (BD 566104, clone SK3, 1:20), CD8 FITC (BD 555366, clone RPA-T8, 1:5), IFNγ PE (BD 559327, clone B27, 1:5), TNF APC (BD 551384, clone MAb11, 1:5), IL-2 BV421 (BD 562914, clone 5344.111, 1:20), IL-2 BV786 (BD 564113, clone MP4-25D2, 1:10), IL-13 BV711 (BD 564288, clone JES10-5A2, 1:10) and CD19 PerCP-Cy5.5 (BD 561295, clone HIB19, 1:20) markers. ICS was executed utilizing the BD cytofix/cytoperm kit following the manufacturer’s instructions. Antibody incubation was carried out for 30 min at 4 °C. Phorbol myristate acetate (PMA)–ionomycin was used as positive control. To assess the B cell population specific to the B.1.1.529 spike protein, PBMCs were initially labeled with biotinylated spike protein specific to Omicron B.1.1.529. Subsequently, surface staining was performed with common surface markers CD3 BV605 (BD 563219, clone SK7, 1:20), CD19 PerCP-Cy5.5 (BD 561295, clone HIB19, 1:20), CD20 APC-H7 (BD 560734, clone 2H7, 1:20). Following staining and washing steps, PBMCs were resuspended in fluorescence-activated cell sorting buffer, acquired using the FACSLyric system (BD Biosciences), and analyzed using FlowJo software version 10.8.1 (FlowJo LLC, BD Biosciences).
Safety assessment
Solicited events data were captured up to 7 days after booster vaccine administration through an electronic or paper diary. Local solicited events included pain, redness, swelling, warmth, pruritus and bruising. Systemic solicited events included fever, headache, myalgia, arthralgia, fatigue, malaise, nausea and chills. Unsolicited events were assessed throughout the duration of the study. AE terms were coded using Medical Dictionary for Regulatory Activities. These AEs were graded on the basis of the Division of AIDS criteria 49 . Myocarditis was considered as an AE of special interest; site investigators were asked to thoroughly evaluate participants with any symptoms of chest pain, breathlessness or palpitations.
Statistical analysis
No formal sample size calculations were performed for phase 2. Phase 3 consisted of a safety and an immunogenicity cohort. The safety cohort consisted of 3,140 participants of whom 3,000 were included in the GEMCOVAC-OM arm. The immunogenicity cohort was analyzed for two primary endpoints based on World Health Organization guidelines 50 , with individuals randomized to GEMCOVAC-OM and ChAdOx1 nCoV-19 in a 2:1 ratio. A sample size of 420 (280 in GEMCOVAC-OM and 140 in ChAdOx1 nCoV-19) was found adequate for assessing noninferiority of neutralizing antibody titers if the lower limit of the two-sided 95% CI of the LSGMR (GMT GEMCOVAC-OM /GMT ChAdOx1 nCoV-19 ) was >0.67 considering a standard deviation of 1.82, alpha error of 5%, power of 90% and dropout rate of 20%. A sample size of 381 (254 in GEMCOVAC-OM and 127 in ChAdOx1 nCoV-19) was found adequate for assessing the noninferiority of seroconversion difference considering a margin of −10%, alpha error of 5%, power of 90% and dropout rate of 20%. The sample size of 420 (280 in GEMCOVAC-OM and 140 in ChAdOx1 nCoV-19 arm) was considered in this study to provide adequate numbers for the statistical analysis of both the primary endpoints.
All immunogenicity analysis was performed in the full analysis set (intention to treat) population. The observed GMT and associated 95% CIs (Clopper–Pearson method) were calculated on the basis of log-transformed antibody titers. The rise in neutralizing antibody titers from baseline to day 29 was compared using a paired t -test. The LSGMR of neutralizing antibody titers in both the arms at day 29 from the PRNT 50 (BA.1 strain of SARS-CoV-2) assay was calculated using ANCOVA with baseline titers as covariates. If the lower limit of the two-sided 95% CI of the LSGMR was >0.67, GEMCOVAC-OM would be considered noninferior to ChAdOx1 nCoV-19. Seroconversion in terms of neutralizing antibody titers from PRNT 50 assay was defined as a ≥2-fold rise in titers at day 29 from baseline. The difference in seroconversion was determined using the Meitinen–Nurminen method. If the lower bound of the two-sided 95% CI for seroconversion difference was >−10%, GEMCOVAC-OM would be considered noninferior to ChAdOx1 nCoV-19.
Similarly, for the secondary endpoint, LSGMR of anti-Spike IgG antibody titers from ELISA at day 29 was assessed using ANCOVA, with baseline titers as covariates. The 95% CI was calculated for percentage by using the Clopper–Pearson method. The difference in seroconversion at day 29 for anti-spike IgG antibodies from ELISA were calculated using the Meitinen–Nurminen method. The difference in the change in mean percentage neutralization from baseline to day 29, assessed by the cPass assay, was analyzed using ANCOVA with baseline neutralization considered as a covariate. In cell-mediated immunity, change in expression from baseline to day 29 was assessed by either a two-sided paired t -test or Wilcoxon signed-rank test depending on normality of the data. Expression at day 29 was compared using a two-sided t -test or Wilcoxon rank sum base based on normality. The normality of the data was assessed using a Shapiro–Wilk test. This was also performed for subgroups based on their primary vaccination of either BBV152 or ChAdOx1 nCoV-19.
Sex-disaggregated analysis was conducted for humoral and safety data from phase 3. For humoral immunogenicity, ANCOVA was used to compare the differences in neutralizing antibody and anti-spike IgG between men and women at days 29 and 90, using baseline titers as covariates. For safety, unadjusted OR was calculated along with the 95% CI.
Data were collected using Clinion (version 3.1). Statistical analysis for humoral immunogenicity was performed using Statistical software SAS version 9.4 (SAS Institute). Figures were generated using GraphPad Prism (Version 9.5.1). The Spearman rank correlation coefficient (denoted as ‘ r ’) was computed for all pairs of parameters utilizing the corrplot package (version 0.92) within RStudio (version 2022.12.0.0). To complement the correlogram, two-tailed P values associated with Spearman rank correlations were calculated through the corr.mtest function and visualized using the corrplot function.
Reporting summary
Further information on research design is available in the Nature Portfolio Reporting Summary linked to this article.
Data availability
Individual participant data will be made available for meta-analysis when the trial is complete. The request should be approved by an ethics committee or the institutional review board of the institution to which the person requesting the information belongs. If approved, it should be directed to the corresponding author at [email protected]. The requester will need to sign a data access agreement. Data will be shared through a secure online platform within 2 months from the signing of the access agreement. The aggregated data are included in this manuscript. DDBJ datasets were used to design GEMCOVAC-19 (accession no. LC776732.1) and GEMCOVAC-OM (accession no. LC769018).
Code availability
No custom code or mathematical algorithms were used in this study.
World Health Organization. COVID-19 dashboard; https://covid19.who.int (2024).
Tregoning, J. S., Flight, K. E., Higham, S. L., Wang, Z. & Pierce, B. F. Progress of the COVID-19 vaccine effort: viruses, vaccines and variants versus efficacy, effectiveness and escape. Nat. Rev. Immunol. 21 , 626–636 (2021).
Article CAS PubMed PubMed Central Google Scholar
Gote, V. et al. A comprehensive review of mRNA vaccines. Int. J. Mol. Sci. 24 , 2700 (2023).
Planas, D. et al. Considerable escape of SARS-CoV-2 Omicron to antibody neutralization. Nature 602 , 671–675 (2022).
Article CAS PubMed Google Scholar
Wadapurkar, R., Singh, S. & Singh, A. Leveraging the immunoinformatics approach for designing the SARS-CoV-2 Omicron-specific antigenic cassette of mRNA vaccine. Vaccine 42 , 1630–1647 (2024).
Comes, J. D. G., Pijlman, G. P. & Hick, T. A. H. Rise of the RNA machines—self-amplification in mRNA vaccine design. Trends Biotechnol . https://doi.org/10.1016/j.tibtech.2023.05.007 (2023).
Bloom, K., van den Berg, F. & Arbuthnot, P. Self-amplifying RNA vaccines for infectious diseases. Gene Ther. 28 , 117–129 (2021).
First self-amplifying mRNA vaccine approved. Nat. Biotechnol . 42 , 4 (2024).
Oda, Y. et al. Immunogenicity and safety of a booster dose of a self-amplifying RNA COVID-19 vaccine (ARCT-154) versus BNT162b2 mRNA COVID-19 vaccine: a double-blind, multicentre, randomised, controlled, phase 3, non-inferiority trial. Lancet Infect. Dis. 24 , 351–360 (2024).
Rice, A. et al. Heterologous saRNA prime, DNA dual-antigen boost SARS-CoV-2 vaccination elicits robust cellular immunogenicity and cross-variant neutralizing antibodies. Front. Immunol. 13 , 910136 (2022).
Palmer, C. D. et al. GRT-R910: a self-amplifying mRNA SARS-CoV-2 vaccine boosts immunity for ≥6 months in previously-vaccinated older adults. Nat. Commun. 14 , 3274 (2023).
Bozkurt, B. Shedding light on mechanisms of myocarditis with COVID-19 mRNA vaccines. Circulation 147 , 877–880 (2023).
Garcia-Beltran, W. F. et al. mRNA-based COVID-19 vaccine boosters induce neutralizing immunity against SARS-CoV-2 Omicron variant. Cell 185 , 457–466.e4 (2022).
Garcia-Beltran, W. F. et al. Multiple SARS-CoV-2 variants escape neutralization by vaccine-induced humoral immunity. Cell 184 , 2372–2383.e9 (2021).
Zhou, D. et al. Evidence of escape of SARS-CoV-2 variant B.1.351 from natural and vaccine-induced sera. Cell 184 , 2348–2361.e6 (2021).
Tian, F. et al. N501Y mutation of spike protein in SARS-CoV-2 strengthens its binding to receptor ACE2. eLife 10 , e69091 (2021).
Collie, S., Champion, J., Moultrie, H., Bekker, L.-G. & Gray, G. Effectiveness of BNT162b2 vaccine against Omicron variant in South Africa. N. Engl. J. Med. 386 , 494–496 (2022).
Article PubMed Google Scholar
Tseng, H. F. et al. Effectiveness of mRNA-1273 against SARS-CoV-2 Omicron and Delta variants. Nat. Med. 28 , 1063–1071 (2022).
Pulliam, J. R. C. et al. Increased risk of SARS-CoV-2 reinfection associated with emergence of Omicron in South Africa. Science 376 , eabn4947 (2022).
Chalkias, S. et al. A bivalent Omicron-containing booster vaccine against Covid-19. N. Engl. J. Med. 387 , 1279–1291 (2022).
Winokur, P. et al. Bivalent Omicron BA.1-adapted BNT162b2 booster in adults older than 55 years. N. Engl. J. Med. 388 , 214–227 (2023).
Offit, P. A. Bivalent Covid-19 vaccines—a cautionary tale. N. Engl. J. Med. 388 , 481–483 (2023).
World Health Organization. Statement on the antigen composition of COVID-19 vaccines; https://www.who.int/news/item/18-05-2023-statement-on-the-antigen-composition-of-covid-19-vaccines (2023).
Khoury, D. S. et al. Neutralizing antibody levels are highly predictive of immune protection from symptomatic SARS-CoV-2 infection. Nat. Med. 27 , 1205–1211 (2021).
Bewley, K. R. et al. Quantification of SARS-CoV-2 neutralizing antibody by wild-type plaque reduction neutralization, microneutralization and pseudotyped virus neutralization assays. Nat. Protoc. 16 , 3114–3140 (2021).
Points to consider on switching between superiority and non-inferiority. Br. J. Clin. Pharmacol . 52 , 223–228 (2001).
Wong, J. Y. et al. Intrinsic and effective severity of COVID-19 cases infected with the ancestral strain and Omicron BA.2 variant in Hong Kong. J. Infect. Dis. 228 , 1231–1239 (2023).
Naranbhai, V. et al. T cell reactivity to the SARS-CoV-2 Omicron variant is preserved in most but not all prior infected and vaccinated individuals. Cell https://doi.org/10.1016/j.cell.2022.03.022 (2022).
Antoun, E., Peng, Y. & Dong, T. Vaccine-induced CD8 + T cells are key to protection from SARS-CoV-2. Nat. Immunol. 24 , 1594–1596 (2023).
Painter, M. M. et al. Prior vaccination promotes early activation of memory T cells and enhances immune responses during SARS-CoV-2 breakthrough infection. Nat. Immunol. 24 , 1711–1724 (2023).
Bogdan, C., Moll, H., Solbach, W. & Röllinghoff, M. Tumor necrosis factor-alpha in combination with interferon-gamma, but not with interleukin 4 activates murine macrophages for elimination of Leishmania major amastigotes. Eur. J. Immunol. 20 , 1131–1135 (1990).
Liew, F. Y., Li, Y. & Millott, S. Tumor necrosis factor-alpha synergizes with IFN-gamma in mediating killing of Leishmania major through the induction of nitric oxide. J. Immunol. 145 , 4306–4310 (1990).
Kahan, S. M. et al. Intrinsic IL-2 production by effector CD8 T cells affects IL-2 signaling and promotes fate decisions, stemness, and protection. Sci. Immunol. 7 , eabl6322 (2022).
Graham, B. S. Rapid COVID-19 vaccine development. Science 368 , 945–946 (2020).
Akkaya, M., Kwak, K. & Pierce, S. K. B cell memory: building two walls of protection against pathogens. Nat. Rev. Immunol. 20 , 229–238 (2020).
Flanagan, K. L., Fink, A. L., Plebanski, M. & Klein, S. L. Sex and gender differences in the outcomes of vaccination over the life course. Annu. Rev. Cell Dev. Biol. 33 , 577–599 (2017).
Marchevsky, N. G. et al. An exploratory analysis of the response to ChAdOx1 nCoV-19 (AZD1222) vaccine in males and females. eBioMedicine 81 , 104128 (2022).
Chapin-Bardales, J. et al. Reactogenicity within 2 weeks after mRNA COVID-19 vaccines: findings from the CDC v-safe surveillance system. Vaccine 39 , 7066–7073 (2021).
Menni, C. et al. Vaccine side-effects and SARS-CoV-2 infection after vaccination in users of the COVID Symptom Study app in the UK: a prospective observational study. Lancet Infect. Dis. 21 , 939–949 (2021).
Hettinga, J. & Carlisle, R. Vaccination into the dermal compartment: techniques, challenges, and prospects. Vaccines 8 , 534 (2020).
Daly, C. et al. Needle-free injectors for mass administration of fractional dose inactivated poliovirus vaccine in Karachi, Pakistan: a survey of caregiver and vaccinator acceptability. Vaccine 38 , 1893–1898 (2020).
Lancet Commission on COVID-19 Vaccines and Therapeutics Task Force Members. Urgent needs of low-income and middle-income countries for COVID-19 vaccines and therapeutics. Lancet 397 , 562–564 (2021).
Uddin, M. N. & Roni, M. A. Challenges of storage and stability of mRNA-based COVID-19 vaccines. Vaccines 9 , 1033 (2021).
Pardi, N., Hogan, M. J., Porter, F. W. & Weissman, D. mRNA vaccines—a new era in vaccinology. Nat. Rev. Drug Discov. 17 , 261–279 (2018).
Deo, S. et al. Evaluation of self-amplifying mRNA platform for protein expression and genetic stability: implication for mRNA therapies. Biochem. Biophys. Res. Commun. 680 , 108–118 (2023).
Shrivastava, S. et al. Early and high SARS-CoV-2 neutralizing antibodies are associated with severity in COVID-19 patients from India. Am. J. Trop. Med. Hyg. 105 , 401–406 (2021).
CAS PubMed PubMed Central Google Scholar
Taylor, S. C. et al. A new SARS-CoV-2 dual-purpose serology test: highly accurate infection tracing and neutralizing antibody response detection. J. Clin. Microbiol. 59 , e02438–20 (2021).
Lu, L. L. et al. IFN-γ-independent immune markers of Mycobacterium tuberculosis exposure. Nat. Med. 25 , 977–987 (2019).
National Institute of Allergy and Infectious Diseases. DAIDS adverse event grading tables; https://rsc.niaid.nih.gov/clinical-research-sites/daids-adverse-event-grading-tables (2017).
World Health Organization. Considerations for the assessment of COVID-19 vaccines for listing by WHO; https://www.who.int/publications/m/item/considerations-for-the-assessment-of-covid-19-vaccines-for-listing-by-who (2020).
Download references
Acknowledgements
The authors are grateful to all the study participants, principal investigators and staff from all 20 hospitals across India who were involved in this study. The authors thank the team at IRSHSA for their contributions to the immunogenicity and JSS Medical Research for their role in supporting the clinical trial. The authors thank the DSMB members (M. Gupte, C. Yajnik, S. Salvi and S. Deshpande) for ensuring the safety of the study participants and for their invaluable guidance. A full list of acknowledgements is provided in Supplementary Information . This study was partially funded through grants from the Department of Biotechnology (DBT), Government of India under the Ind CEPI project (BT/COVID0041/01/20) and the Mission COVID Suraksha, which is the Indian COVID-19 Vaccine Development Mission, managed by the Biotechnology Industry Research Assistance Council (BIRAC) and DBT (BT/CS0002/CS/01/20). Additionally, Gennova Biopharmaceuticals Limited sponsored and partially funded the study. All authors were supported by internal organization’s salary structure. Gennova Biopharmaceuticals Limited was involved with study design and assessment.
Author information
These authors contributed equally: Amit Saraf, Rohan Gurjar.
Authors and Affiliations
Gennova Biopharmaceuticals Limited, Pune, India
Amit Saraf, Rohan Gurjar, Swarnendu Kaviraj, Aishwarya Kulkarni, Durgesh Kumar, Ajay Singh, Arjun Raghuwanshi, Praveen Agarwal, Laxman Savergave & Sanjay Singh
Department of Communicable Diseases, Interactive Research School for Health Affairs, Bharati Vidyapeeth (Deemed to Be University), Pune, India
Ruta Kulkarni & Rashmi Virkar
JSS Medical Research, Haryana, India
Jayashri Krishnan, Anjali Yadav & Ekta Baranwal
Cytel, Pune, India
Ekta Baranwal
Ace Hospital and Research Centre, Pune, India
- Himanshu Pophale
Lokamanya Medical Research Centre, Pune, India
Prakash Shende
CIMETs Inamdar Multispeciality Hospital, Pune, India
Ravindra Baban Shinde
Dr. D. Y. Patil Medical College Hospital and Research Centre, Pune, India
Vikram Vikhe
Vedant Multispeciality Hospital, Pune, India
Abhishek Karmalkar
Baramati Hospital, Pune, India
Bhaskar Deshmukh
Dhadiwal Hospital in coalition with Shreeji Healthcare, Nashik, India
Krishna Giri
Ashirwad Hospital and Research Centre, Ulhasnagar, India
Shrikant Deshpande
Meditrina Institute of Medical Sciences, Nagpur, India
All India Institute of Medical Sciences, Raipur, India
Md. Sabah Siddiqui
Downtown Hospital Ltd, Guwahati, India
Swapnav Borthakur
Induss Hospital, Hyderabad, India
V. Reddy Tummuru
St Theresa’s Hospital, Hyderabad, India
A. Venkateshwar Rao
V S General Hospital, Ahmedabad, India
Dhaiwat Shukla
Maharaja Agrasen Super Specialty Hospital, Jaipur, India
Manish Kumar Jain
All India Institute of Medical Sciences, Jodhpur, India
Pankaj Bhardwaj
Supe Heart & Diabetes Hospital and Research Centre, Nashik, India
Pravin Dinkar Supe
SRMSIMS Hospital, Bareilly, India
Manoja Kumar Das
Suyash Institute of Medical Sciences Pvt Ltd, Raipur, India
Manoj Lahoti
Rajarshee Chhatrapati Shahu Maharaj Government Medical College and Chhatrapati Pramila Raje Hospital, Kolhapur, India
Vijaykumar Barge
You can also search for this author in PubMed Google Scholar
- , Prakash Shende
- , Ravindra Baban Shinde
- , Vikram Vikhe
- , Abhishek Karmalkar
- , Bhaskar Deshmukh
- , Krishna Giri
- , Shrikant Deshpande
- , Ajay Bulle
- , Md. Sabah Siddiqui
- , Swapnav Borthakur
- , V. Reddy Tummuru
- , A. Venkateshwar Rao
- , Dhaiwat Shukla
- , Manish Kumar Jain
- , Pankaj Bhardwaj
- , Pravin Dinkar Supe
- , Manoja Kumar Das
- , Manoj Lahoti
- & Vijaykumar Barge
Contributions
A. Saraf and R.G. were involved with design of the clinical study and manuscript preparation. S.K. and A. Kulkarni conducted ELISA. D.K. was involved with cellular immune response assessment and manuscript preparation. R.K. and R.V. conducted PRNT 50 assays. E.B. and A.Y. were involved in the statistical analysis. J.K. led the monitoring of the clinical trial. A. Singh, A.R., P.A., L.S., S.K. and S.S. were involved with the development of the vaccine. All authors contributed and provided important data for the study. All authors have reviewed the manuscript.
Corresponding author
Correspondence to Sanjay Singh .
Ethics declarations
Competing interests.
A. Saraf, R.G., S.K., A. Kulkarni, D.K., A. Singh, A.R., P.A., L.S. and S.S. are employees of Gennova Biopharmaceuticals Limited. J.K., A.Y. and E.B. are employees of JSS Medical Research, the CRO hired by Gennova Biopharmaceuticals Limited for conducting this study. R.K. and RV are employees of IRSHA whose services were utilized by Gennova Biopharmaceuticals Limited for PRNT analysis. The GEMCOVAC-OM Study Investigators H.P., P.S., R.B.S., V.V., A. Karmalkar, B.D., K.G., S.D., A.B., M.S.S., S.B., V.R.T., A.V.R., D.S., M.K.J., P.B., P.D.S., M.K.D., M.L. and V.B. were clinical trial site investigators who were funded by Gennova Biopharmaceuticals Limited for their contribution to this study.
Peer review
Peer review information.
Nature Medicine thanks the anonymous reviewer(s) for their contribution to the peer review of this work. Primary Handling Editor: Alison Farrell, in collaboration with the Nature Medicine team.
Additional information
Publisher’s note Springer Nature remains neutral with regard to jurisdictional claims in published maps and institutional affiliations.
Extended data
Extended data fig. 1 lymphocytes populations in chadox1 ncov-19 and gemcovac-om cohorts..
( A ) Total T-cells (CD3 + cells) population in % of lymphocyte gate applied, ( B ) total CD4 + T-cells population in % of total CD3 + T-cells, ( C ) total CD8 + T-cells in % of total CD3 + T-cells in ChAdOx1 nCoV-19 (n = 35) and GEMCOVAC-OM (n = 71) cohorts. ( D ) Total CD19 + CD20 + B-cells in % of CD3 − gated population in ChAdOx1 nCoV-19 (n = 34) and GEMCOVAC-OM (n = 67) cohorts. Box plots represent the median, 25 th and 75 th percentiles. Whiskers extend from the minimum to maximum values in the data set. Change in T-cells from baseline to day 29 and day 90 was assessed using a two-sided paired t-test or Wilcoxon sign ranked test based on normality. Expression at day 29 and day 90 in both the groups was compared using a two-sided t-test or Wilcoxon rank sum test based on normality.
Extended Data Fig. 2 Th2 response in ChAdOx1 nCoV-19 and GEMCOVAC-OM cohorts.
PBMCs were stimulated with Omicron Spike-specific PepTivator ® . ( A ) IL-4 expressing CD4 + T-cells, ( B ) IL-13 expressing CD4 + T-cells, ( C ) IL-4 expressing CD8 + T-cells and ( D ) IL-13 expressing CD8 + T-cells in ChAdOx1 nCoV-19 (n = 35) and GEMCOVAC-OM (n = 71) cohorts. Data is presented as median with interquartile range (IQR). Change in cytokine expression from baseline to day 29 and day 90 was assessed using a two-sided paired t-test or Wilcoxon sign ranked test based on normality. Expression at day 29 and day 90 in both the groups was compared using a two-sided t-test or Wilcoxon rank sum test based on normality. ns = not significant.
Extended Data Fig. 3 Gating strategy used to measure Omicron B.1.1.529.
( A ) Spike-specific effector T-cell responses and ( B ) B-cell population.
Supplementary information
Supplementary information.
Additional phase 2 and phase 3 data; antibodies, reagents, cells and viruses used for analysis; samRNA platform; methods used for characterization of GEMCOVAC-OM.
Reporting Summary
Supplementary data 1.
Phase 2 and 3 clinical trial protocol.
Supplementary Data 2
Statistical analysis plan for the clinical study.
Rights and permissions
Open Access This article is licensed under a Creative Commons Attribution 4.0 International License, which permits use, sharing, adaptation, distribution and reproduction in any medium or format, as long as you give appropriate credit to the original author(s) and the source, provide a link to the Creative Commons licence, and indicate if changes were made. The images or other third party material in this article are included in the article’s Creative Commons licence, unless indicated otherwise in a credit line to the material. If material is not included in the article’s Creative Commons licence and your intended use is not permitted by statutory regulation or exceeds the permitted use, you will need to obtain permission directly from the copyright holder. To view a copy of this licence, visit http://creativecommons.org/licenses/by/4.0/ .
Reprints and permissions
About this article
Cite this article.
Saraf, A., Gurjar, R., Kaviraj, S. et al. An Omicron-specific, self-amplifying mRNA booster vaccine for COVID-19: a phase 2/3 randomized trial. Nat Med (2024). https://doi.org/10.1038/s41591-024-02955-2
Download citation
Received : 25 September 2023
Accepted : 26 March 2024
Published : 18 April 2024
DOI : https://doi.org/10.1038/s41591-024-02955-2
Share this article
Anyone you share the following link with will be able to read this content:
Sorry, a shareable link is not currently available for this article.
Provided by the Springer Nature SharedIt content-sharing initiative
Quick links
- Explore articles by subject
- Guide to authors
- Editorial policies
Sign up for the Nature Briefing newsletter — what matters in science, free to your inbox daily.

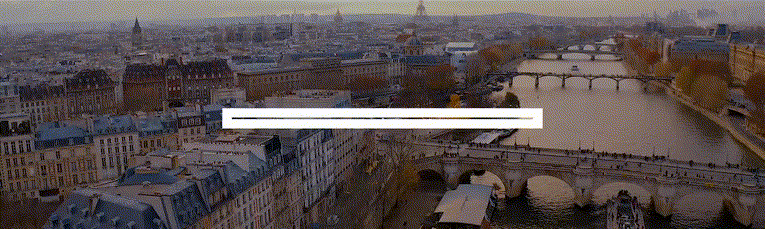
IMAGES
VIDEO
COMMENTS
Established patient E/M levels 1 through 5. 99211: 5 minutes. 99212: 10 minutes. 99213: 15 minutes. 99214: 25 minutes. 99215: 40 minutes. If the patient comes to the clinic only for counseling regarding immunizations required for foreign travel and preventive travel measures, then you might consider codes from the preventive medicine section of ...
Vaccines for Pregnant Travelers. Nasser and colleagues (2020) noted that pregnant travelers and their offspring are vulnerable to severe outcomes following a wide range of infections. Vaccine-preventable diseases can have a particularly severe course in pregnant women, but little is known about the safety of travel vaccines in pregnant women.
Find your vaccine or titer requested below and read the corresponding codes: Vaccines (read BOTH codes if two codes listed) Hepatitis A: 90632 & 90471. Hepatitis B: 90746 & 90471. Tetanus & Diphtheria: 90714 & 90471. Tetanus, Diphtheria & Acellular Pertussis: 90715 & 90471. Measles, Mumps & Rubella: 90707 & 90471.
All existing CPT codes that describe COVID-19 vaccine products and associated administration codes that end in "A" for products that are no longer covered under an existing Emergency Use Authorization (EUA) or Biologics License Application (BLA) from the US Food and Drug Administration (FDA) will be deleted effective Nov. 1, 2023.
to COVID-19 vaccine and vaccine administration, refer to the list of . COVID-19 Vaccine Codes. 90460 . Immunization administration through 18 years of age via any route of administration, with counseling by physician or other qualified health care professional; first or only component of each vaccine or toxoid administered N/A
The structure of the vaccines section of CPT was modified to accommodate COVID-19 vaccine product and immunization administration codes. • For immunization administration other than COVID-19, codes 90460-90474 are reported for the administration of the vaccine, along with the appropriate vaccine/toxoid code (90476- 90756) targeting the ...
Find information about CPT Category I Immunization Codes. ... Find travel, hotel, child care, and inauguration details for the 2024 Annual Meeting of the House of Delegates taking place June 7-12 in Chicago. ... For the purposes of CPT coding, "Immunization" includes vaccines/toxoids, immune globulins, serum or recombinant products. ...
A pretravel consultation enables updating of routine immunizations to protect against illness due to infections for which there is an increased risk of exposure during travel (such as diphtheria, measles, mumps, and varicella) [ 3 ]. Issues related to immunizations for travelers are reviewed here. Other travel-related medical issues and ...
Most vaccinations that are appropriate for international travelers are included in the routine domestic immunization schedule; only a few travel-specific vaccines must also be discussed.
Many yellow fever vaccine centers also provide other pre-travel health care services. Find an authorized US yellow fever vaccine center. Examples of Vaccines. Here is a list of possible vaccines that you may need to get for the first time or boosters before you travel. COVID-19; Chickenpox; Cholera ; Flu (Influenza) Hepatitis A ; Hepatitis B ...
Protect your child and family when traveling in the United States or abroad by: Getting the shots required for all countries you and your family plan to visit during your trip. Making sure you and your family are up-to-date on all routine U.S. vaccines. Staying informed about travel notices and alerts and how they can affect your family's ...
• Contact your insurance carrier prior to your appointment to see if travel vaccines ar e covered o Typhoid (CPT Code: 90691) o Yellow Fever (CPT Code: 90717) o Japanese Encephalitis (CPT Code: 90738); this is a two -shot series 28 days apart o Rabies Pre-Exposure (CPT Code: 90675); this is a three -shot series 28 days interval
home health or hospice care supervision | advance care planning via telehealth | ambulatory blood pressure monitoring | counseling patients about foreign travel | catching up on children's vaccines
The CPT®/RUC Workgroup on E/M is committed to changing the current coding and documentation requirements for office E/M visits to . simplify. the work of the health care provider and . improve the health . of the patient. Guiding Principles: 1. To decrease administrative burden of documentation and coding 2. To decrease the need for audits 3.
Instructions for Use. This Medical Benefit Drug Policy provides assistance in interpreting UnitedHealthcare standard benefit plans. When deciding coverage, the member specific benefit plan document must be referenced as the terms of the member specific benefit plan may differ from the standard plan. In the event of a conflict, the member ...
The Travel Medicine Clinic provides individualized preventive care and education to promote the health and safety of travelers with international destinations. ... Note that the estimator automatically includes a flat physician fee for a new patient visit and a hospital fee when a vaccine CPT code is entered. However, the provider charge ...
Code 90461 is an add-on code reported for each additional vaccine component administered. Report codes 90471-90474 for immunization administration of any vaccine that is not accompanied by face-to ...
Treat vs. Prevent. For example, if a patient gets a tetanus vaccination because of an accidental puncture wound, it's a Part B-covered vaccine. However, if the patient gets a tetanus booster shot, unrelated to injury or illness, it's a Part D-covered vaccine. You can bill in- and out-of-network Part D vaccines and administration fees on 1 ...
AAP Pediatric Coding Newsletter (2020) 15 (5): 8. ICD-10-CM, You Code It! Code Z71.84 was added to International Classification of Diseases, 10th Revision, Clinical Modification ( ICD-10-CM) on October 1, 2019, for reporting travel counseling. When travel counseling is the only service provided at an encounter, a preventive medicine counseling ...
Please note: State funded vaccines will be used for patients age 18 years and younger, when appropriate. Administration . Office visit fee: Contingent on services provided according to St. Luke's coding and billing policies. Administration fee for 1st injection: $45.00 ; Administration fee for each additional injection: $28.00 ; Vaccinations
The file contains the SARS-CoV-2-related CPT codes released since the 2021 data file release on Aug. 31, 2020 and includes: CPT code descriptors (long, medium, and short) Published date; Effective date; Type of change. Visit the COVID-19 CPT vaccine and immunization codes page for more information and to download the files.
In considering this comment, we also identified two CPT codes (90653 and 90658, both flu vaccines) that were inadvertently left off of the list of codes to which the exception for preventive screening tests and vaccines at § 411.355 (h) should apply. Accordingly, we are adding these CPT codes to the list of codes to which the exception at ...
The streamlined structure brings greater alignment between CPT and the current COVID-19 vaccine reporting environment. "The new coding system will allow for new vaccines for new variants whenever they come up without having to do a new code," said Samuel "Le" Church, MD, MPH, a member of the CPT Editorial Panel and vice-chair of its ...
Effective for dates of service on or after April 1, 2024, vaccine services procedure code 90623 is available through the Texas Vaccines for Children (TVFC) program and will now process as informational only for clients who are 10 years through 18 years of age. Affected claims that are submitted with dates of service from April 1, 2024, through ...
G0311 - Immunization counseling by a physician or other qualified health care professional when the vaccine (s) is not administered on the same date of service, 16-30 minutes. (This code is used ...
Here we conducted a multicenter open-label, randomized phase 2 and 3 study to assess the safety and immunogenicity of a severe acute respiratory syndrome coronavirus 2 (SARS-CoV-2) Omicron ...
WHO authorized the vaccine, made by EuBiologics, which also makes the formulation now used, last week. The new version, called Euvichol-S, is a simplified formula that uses fewer ingredients, is ...