
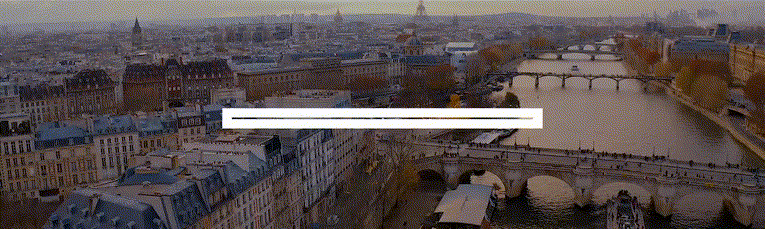
American Geosciences Institute
- Policy & Critical Issues
- Advisory Committees
- Coalitions & Working Groups
Explore the Geosciences
- Mineral Resources
Frequently Asked Questions
- Geoscience In Your State
- Policy News & Monthly Review
- Policy Annual Review
- Federal Geoscience Funding
Policy Positions
- Codes of Ethics
- Critical Needs
- Letters and Statements
- Policy Recommendations
- Position Statements
- Case Studies
- Geoscience in Your State
- GOLI Online Courses
Maps & Visualizations
- Petroleum and the Environment
- Policy Publications
- Research Database
- Congressional Briefings
- Congressional Visits Days
Get Involved
- Communicating with Congress
- Fellowships
- Internships
External Resources
- U.S. Congress
- Federal Agencies
- State & Local Agencies
Landslide Basics

Landslides are masses of earth, rock, or debris that move down slopes. Landslides are triggered by one event, but many causes can weaken slopes over time and make them more likely to fail when there is a triggering event. These causes can be both natural and artificial. Landslides often occur in areas with oversteepened slopes, weak soils/bedrock, or de-vegetated slopes (whether by human deforestation or natural events such as wildfires). [1] Some of the most damaging landslides are triggered by water, typically from intense short-term rainfall or long-term saturation of the slope. Both natural and human activities (such as irrigation or seepage ) can saturate hillsides. Earthquakes and volcanic eruptions also cause damaging landslides. [1]
Why do landslides matter?
Landslides affect all 50 states and U.S. territories, though mountainous regions such as the Pacific Coast range, the Rockies, the Appalachians, Alaska, and Hawaii bear the most severe risk. The U.S. Geological Survey estimates that each year in the United States, landslides cause the deaths of 25 to 50 people and losses of at least $1 billion. [2]
How does geoscience help?
Geoscientists map areas that may move so homeowners and emergency managers can plan ahead. They map these areas by locating old landslide features, which indicate areas that have moved in the past. They evaluate the slopes’ vulnerability by studying their features such as soil, bedrock, slope steepness, vegetation cover, and water table height. They also study the triggers of both natural and artificial landslides.
1 Landslide Types and Processes, U.S. Geological Survey, https://pubs.usgs.gov/fs/2004/3072/fs-2004-3072.html
2 Landslides 101, U.S. Geological Survey, https://landslides.usgs.gov/learn/ls101.php
Introductory Resources
- Landslides 101 (Webpage), U.S. Geological Survey A definition of landslides, list of factors that can cause landslides, brief overview of where landslides occur, and discussion of why it is important to study landslides.
- Landslide Types and Processes (Fact sheet), U.S. Geological Survey Provides an overview of the classification of landslides and a thorough list of landslide causes. Discusses the causes of the most damaging landslides. Includes diagrams of different landslide types.
- The Landslides Handbook– A Guide to Understanding Landslides (Handbook), U.S. Geological Survey This comprehensive resource for a lay audience includes detailed information on types of landslides, where landslides occur, landslide causes, and landslide effects/consequences. Also includes extensive sections on evaluating landslide hazard, communicating landslide hazard, and techniques for mitigating landslides.
Resources for Educators
- Education Resources Network, AGI's Center for Geoscience & Society Search for landslide resources in: Curricula & Instruction , Teaching Media
- NGSS Performance Expectations, Next Generation Science Standards 3-ESS3-1 , 4-ESS3-2 , MS-ESS3-2 , HS-ESS3-1
- NGSS Disciplinary Core Ideas , Next Generation Science Standards ESS3.B
Do you have a question that's not listed here? Search all FAQs

Search all Maps & Visualizations >
- Board of Directors
- AGI Connections Newsletter
- Nominations
- News and Announcements
- Membership Benefits
- Strategic Plan
- Annual Report
- Member Societies
- Member Society Council
- International Associates
- Trade Associates
- Academic Associates
- Regional Associates
- Liaison Organizations
- Community Documents
- Geoscience Calendar
- Center for Geoscience & Society
- Education & Outreach
- Diversity Activities
- Scholarly Information
- Geoscience Profession
- Earth Science Week
- Publication Store
- Glossary of Geology
- Earth Science Week Toolkit
- I'm a Geoscientist
- Free Geoscience Resources
- AGI Connections
- Be a Visiting Geoscientist
- Use Our Workspace
Does this explain why some landslides travel much further than others?
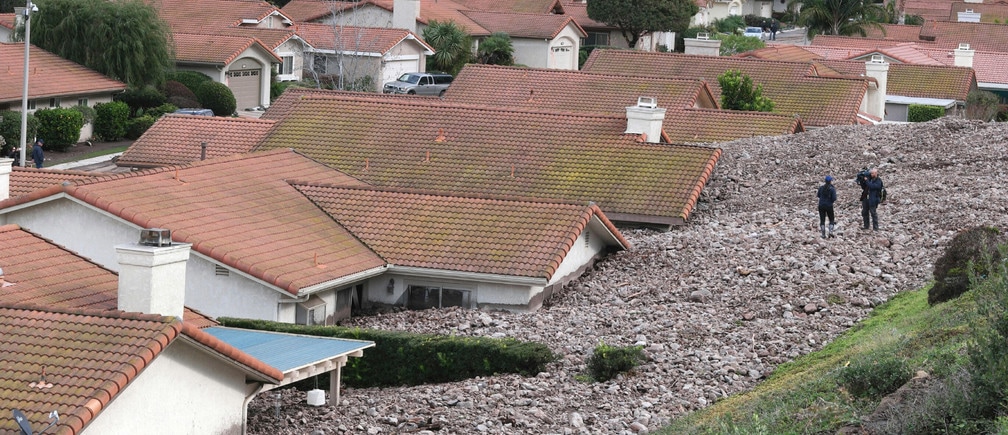
Large vibrations from landslide could allow rocks to behave like fluids, data suggests. Image: REUTERS/Jonathan Alcorn
.chakra .wef-1c7l3mo{-webkit-transition:all 0.15s ease-out;transition:all 0.15s ease-out;cursor:pointer;-webkit-text-decoration:none;text-decoration:none;outline:none;color:inherit;}.chakra .wef-1c7l3mo:hover,.chakra .wef-1c7l3mo[data-hover]{-webkit-text-decoration:underline;text-decoration:underline;}.chakra .wef-1c7l3mo:focus,.chakra .wef-1c7l3mo[data-focus]{box-shadow:0 0 0 3px rgba(168,203,251,0.5);} Kevin Stacey
.chakra .wef-9dduvl{margin-top:16px;margin-bottom:16px;line-height:1.388;font-size:1.25rem;}@media screen and (min-width:56.5rem){.chakra .wef-9dduvl{font-size:1.125rem;}} Explore and monitor how .chakra .wef-15eoq1r{margin-top:16px;margin-bottom:16px;line-height:1.388;font-size:1.25rem;color:#F7DB5E;}@media screen and (min-width:56.5rem){.chakra .wef-15eoq1r{font-size:1.125rem;}} Global Risks is affecting economies, industries and global issues
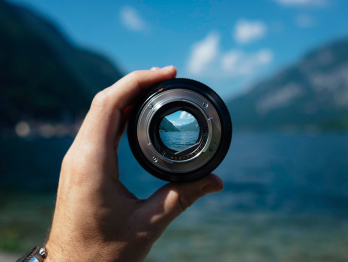
.chakra .wef-1nk5u5d{margin-top:16px;margin-bottom:16px;line-height:1.388;color:#2846F8;font-size:1.25rem;}@media screen and (min-width:56.5rem){.chakra .wef-1nk5u5d{font-size:1.125rem;}} Get involved with our crowdsourced digital platform to deliver impact at scale
Stay up to date:, global risks.
A computer model could explain why some landslides can travel much longer distances than others and cause damage far away from where they started.
The work suggests that vibrations generated by large slides can cause tons of rock to flow like a fluid, enabling the rocks to rumble across vast distances.
The “runout” distance of most landslides—the distance debris travels once it reaches flat land—tends to be about twice the vertical distance that the slide falls. So if a slide breaks loose a half-mile vertically up a slope, it can be expected to run out about a mile.
But “long-runout” landslides, also known as sturzstroms, can travel horizontal distances 10 to 20 times further than they fall, according to Brandon Johnson, an assistant professor of earth, environmental and planetary sciences at Brown University and lead author of the study published in the Journal of Geophysical Research: Earth Surface .
“There are a few examples where these slides have devastated towns, even when they were located at seemingly safe distances from a mountainside,” says Johnson, who started studying these slides as a student of Jay Melosh, a professor of earth, atmospheric, and planetary sciences and physics at Purdue University.
One such example was a slide in 1806 that slammed into the village of Goldau, Switzerland, and claimed nearly 500 lives.
“It has been known for more than a century that very large, dry landslides travel in a fluid-like manner, attaining speeds of more than 100 miles per hour, traveling tens to hundreds of kilometers from their sources and even climbing uphill as they overwhelm surprisingly large areas,” says Melosh, who was a part of the research team. “However, the mechanism by which these very dry piles of rock obtained their fluidity was a mystery.”
Not air or water, but vibrations
Scientists developed several initial hypotheses. Perhaps the slides were floating on a cushion of air, or perhaps they ran atop a layer of water or ice, which would lower the friction they encountered. But the fact that these types of landslides also occur on dry, airless bodies like the Moon cast doubt on those hypotheses.
In 1979, Melosh proposed a mechanism called “acoustic fluidization” to explain these long runouts. Slides of sufficient size, Melosh proposed, would generate vibrational waves that propagate through the rock debris.
[Past earthquakes can make landslides more likely]
Those vibrations reduce the effect of friction acting on the slide, enabling it to travel further than smaller slides, which don’t generate as much vibration. The mechanism is similar to the way a car is more likely to slide if it’s bouncing down a bumpy road as opposed to rolling along a smooth one.
In 1995, Charles Campbell from the University of Southern California created a computer model that was able to replicate the behavior of long-runout slides using only the dynamic interactions between rocks. No special circumstances like water or air cushions were required. However, due to the limitations of computers at the time, he was unable to determine what mechanism was responsible for the behavior.
“The model showed that there was something about rocks, when you get a lot of them together, that causes them to slide out further than you expect,” Johnson says. “But it didn’t tell us what was actually happening to give us this lower friction.”
‘We've now put to rest the debate’
For this new study, Johnson was able to resurrect that model, tweak it a bit, and run it on a modern workstation to capture the dynamics in finer detail. The new model showed that, indeed, vibrations do reduce the effective friction acting on the slide.
The amount of friction acting on a slide depends in part on gravity pulling it downward. The same gravitational force that accelerates the slide as it moves downslope tends to slow it down when it reaches flat land. But the model showed that vibrational waves counteract the gravitational force for brief moments.
The rocks tend to slide more when the vibration reduces the friction effect of the gravitational force. Because the vibrational waves affect different rocks in the slide at different times, the entire slide tends to move more like a fluid.
Those results of the new model are consistent with the acoustic fluidization idea that Melosh had proposed nearly 40 years ago, before computer power was adequate to confirm it.
“Campbell and I had a long-standing friendly rivalry and he did not believe my proposed acoustic fluidization mechanism could possibly explain his findings in the simulations,” Melosh says. “As a result of Brandon’s careful analysis of the interactions of the rock fragments in the simulations, we’ve now put to rest the debate, and it was a lot of fun for the three of us to work together.”
[Rock turns into soil at 'shockingly fast’ pace]
Ultimately, the researchers hope this work might be a step toward better predicting these types of potentially devastating landslides.
“I would suggest that understanding why these landslides run out so far is really is a first step to understanding when and where they might occur in the future,” Johnson says. “Our work suggests that all you need is enough volume to get these long runouts. This leads to the somewhat unsatisfying conclusion that these slides can happen nearly anywhere.”
The results may also help scientists understand other types of events. For example, acoustic fluidization might play a role in slippage along fault lines, which contributes to large earthquakes.
“This emergent phenomenon, arising from the simple interactions of individual particles, is likely at play whenever large movements of rock occur,” Johnson says.
Don't miss any update on this topic
Create a free account and access your personalized content collection with our latest publications and analyses.
License and Republishing
World Economic Forum articles may be republished in accordance with the Creative Commons Attribution-NonCommercial-NoDerivatives 4.0 International Public License, and in accordance with our Terms of Use.
The views expressed in this article are those of the author alone and not the World Economic Forum.
Related topics:
The agenda .chakra .wef-n7bacu{margin-top:16px;margin-bottom:16px;line-height:1.388;font-weight:400;} weekly.
A weekly update of the most important issues driving the global agenda
.chakra .wef-1dtnjt5{display:-webkit-box;display:-webkit-flex;display:-ms-flexbox;display:flex;-webkit-align-items:center;-webkit-box-align:center;-ms-flex-align:center;align-items:center;-webkit-flex-wrap:wrap;-ms-flex-wrap:wrap;flex-wrap:wrap;} More on Global Risks .chakra .wef-17xejub{-webkit-flex:1;-ms-flex:1;flex:1;justify-self:stretch;-webkit-align-self:stretch;-ms-flex-item-align:stretch;align-self:stretch;} .chakra .wef-nr1rr4{display:-webkit-inline-box;display:-webkit-inline-flex;display:-ms-inline-flexbox;display:inline-flex;white-space:normal;vertical-align:middle;text-transform:uppercase;font-size:0.75rem;border-radius:0.25rem;font-weight:700;-webkit-align-items:center;-webkit-box-align:center;-ms-flex-align:center;align-items:center;line-height:1.2;-webkit-letter-spacing:1.25px;-moz-letter-spacing:1.25px;-ms-letter-spacing:1.25px;letter-spacing:1.25px;background:none;padding:0px;color:#B3B3B3;-webkit-box-decoration-break:clone;box-decoration-break:clone;-webkit-box-decoration-break:clone;}@media screen and (min-width:37.5rem){.chakra .wef-nr1rr4{font-size:0.875rem;}}@media screen and (min-width:56.5rem){.chakra .wef-nr1rr4{font-size:1rem;}} See all
Global financial stability at risk due to cyber threats, IMF warns. Here's what to know
Spencer Feingold and Johnny Wood
May 15, 2024
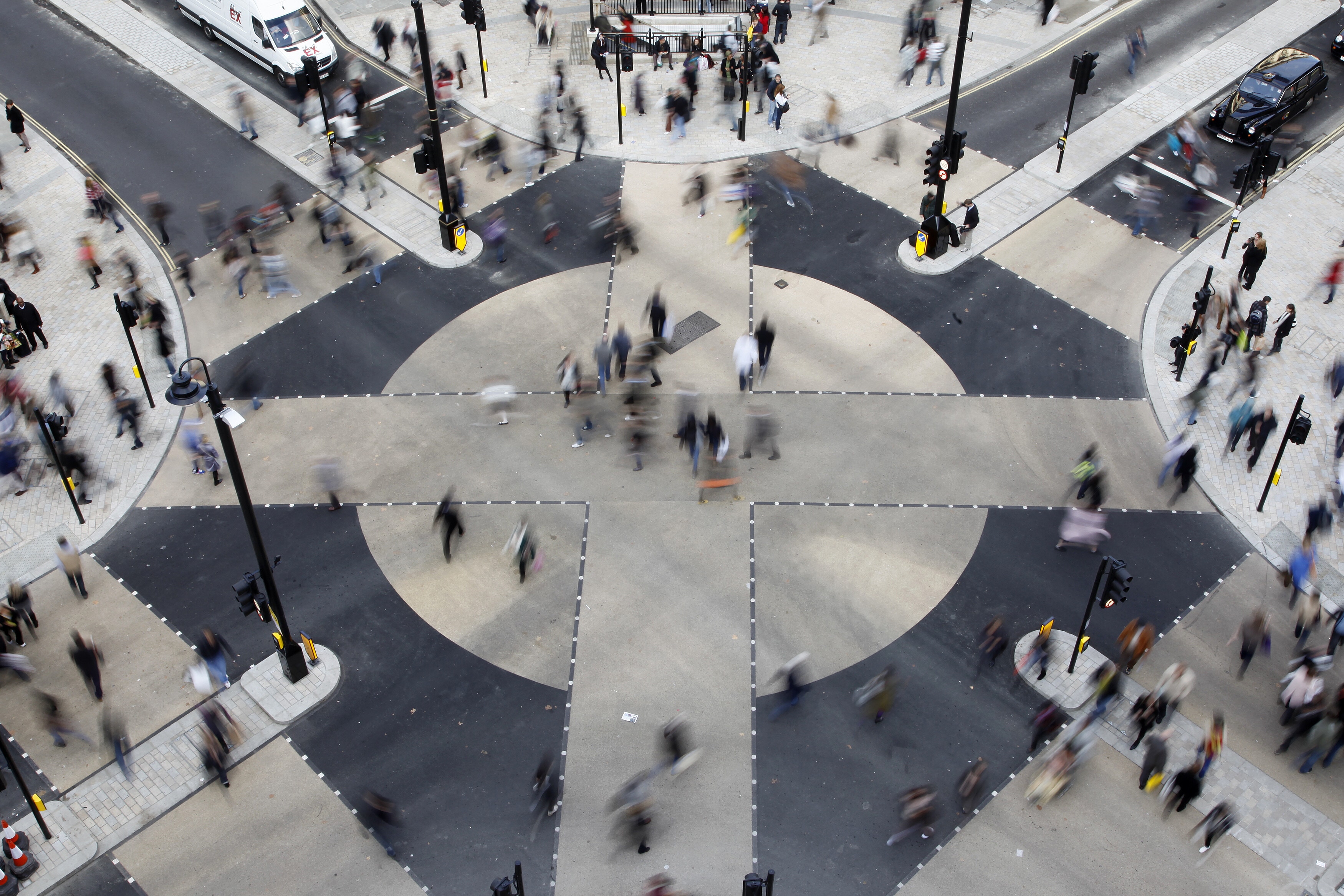
Why shifting from prediction to foresight can help us plan for future disruption
Roger Spitz
May 3, 2024
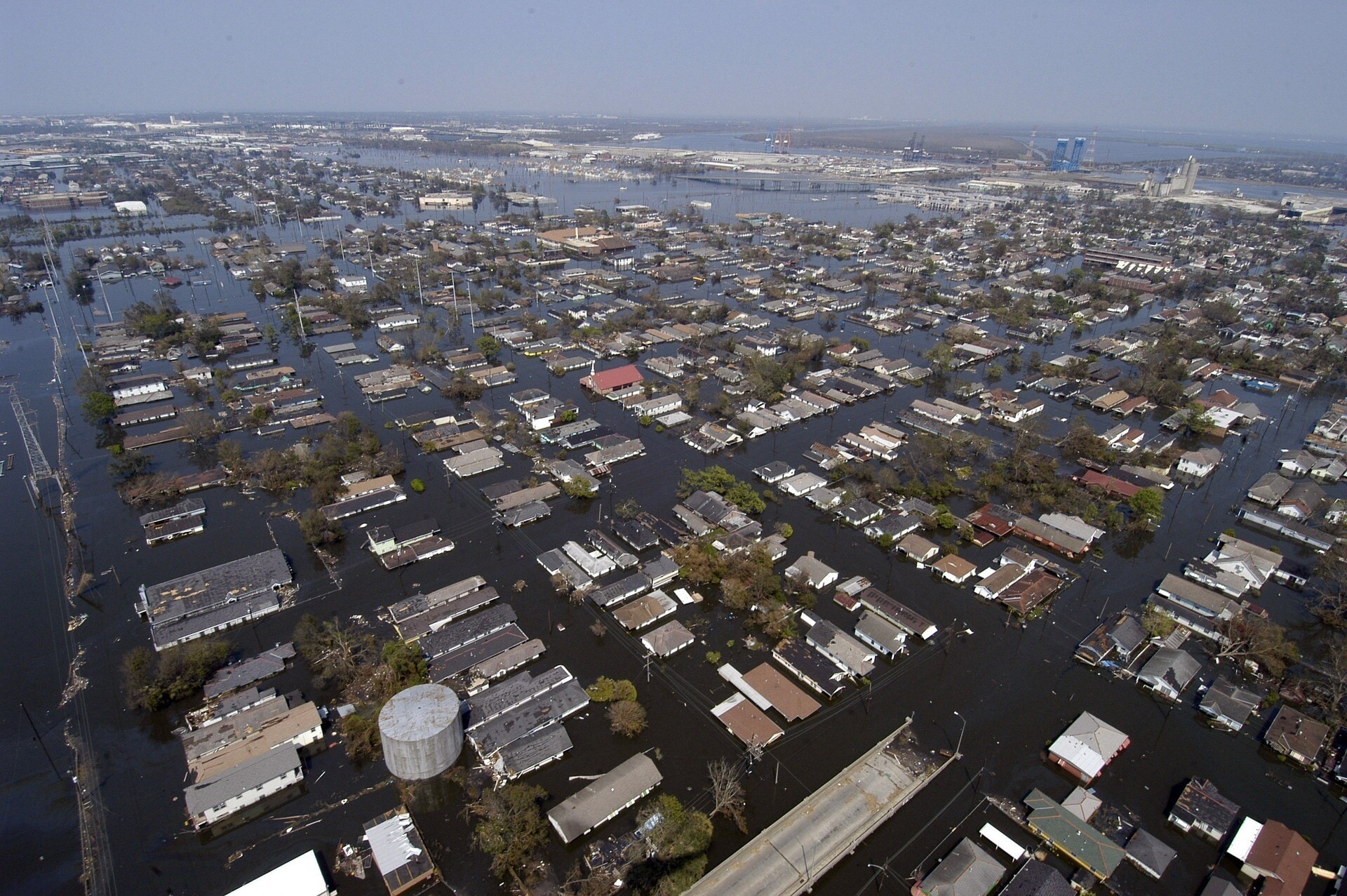
Disasters will be less devastating if we plan for them
Gareth Byatt and Ilan Kelman
March 11, 2024
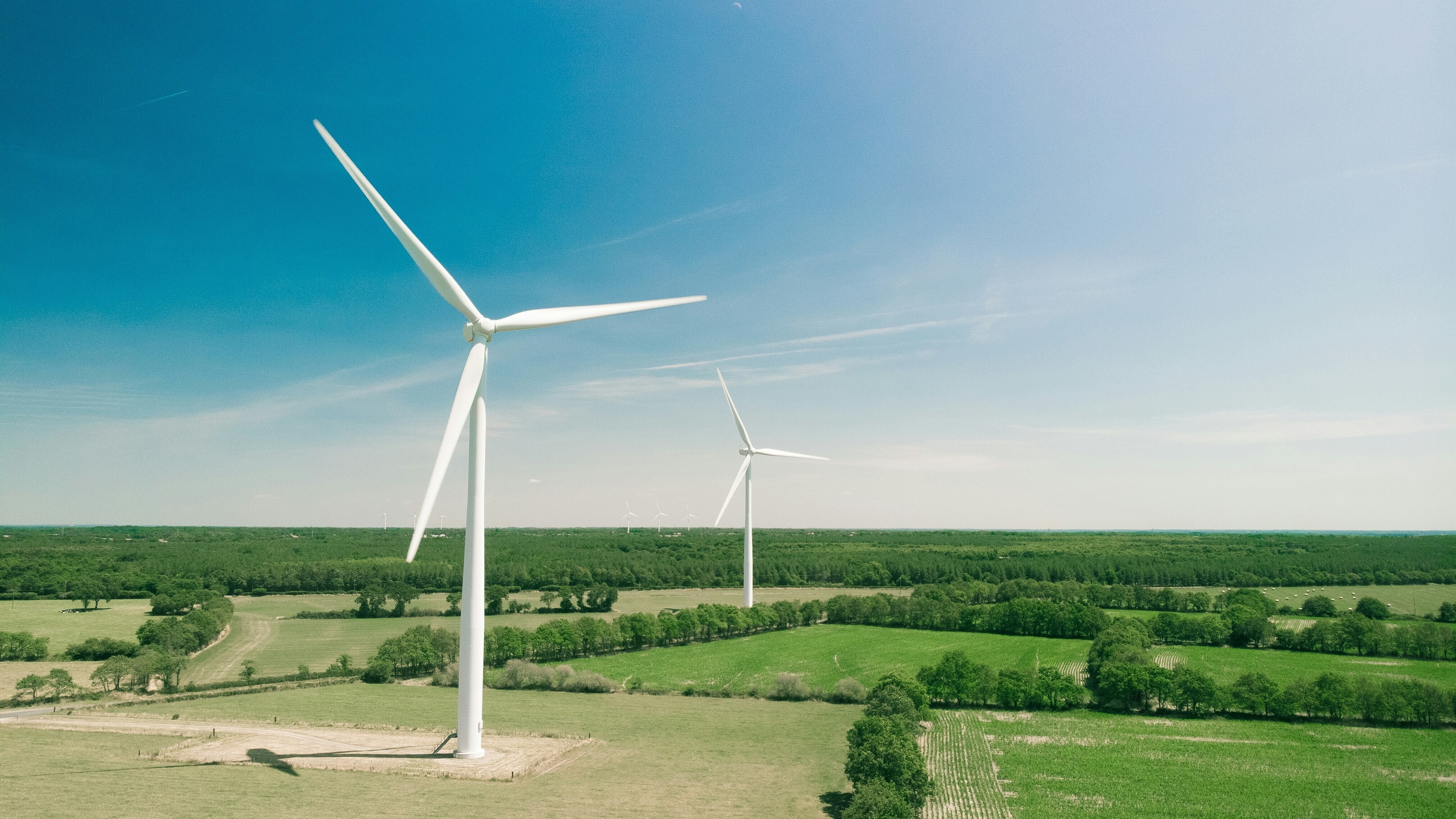
What are forever chemicals, and why does the EU want to ban them?
Andrea Willige
March 6, 2024
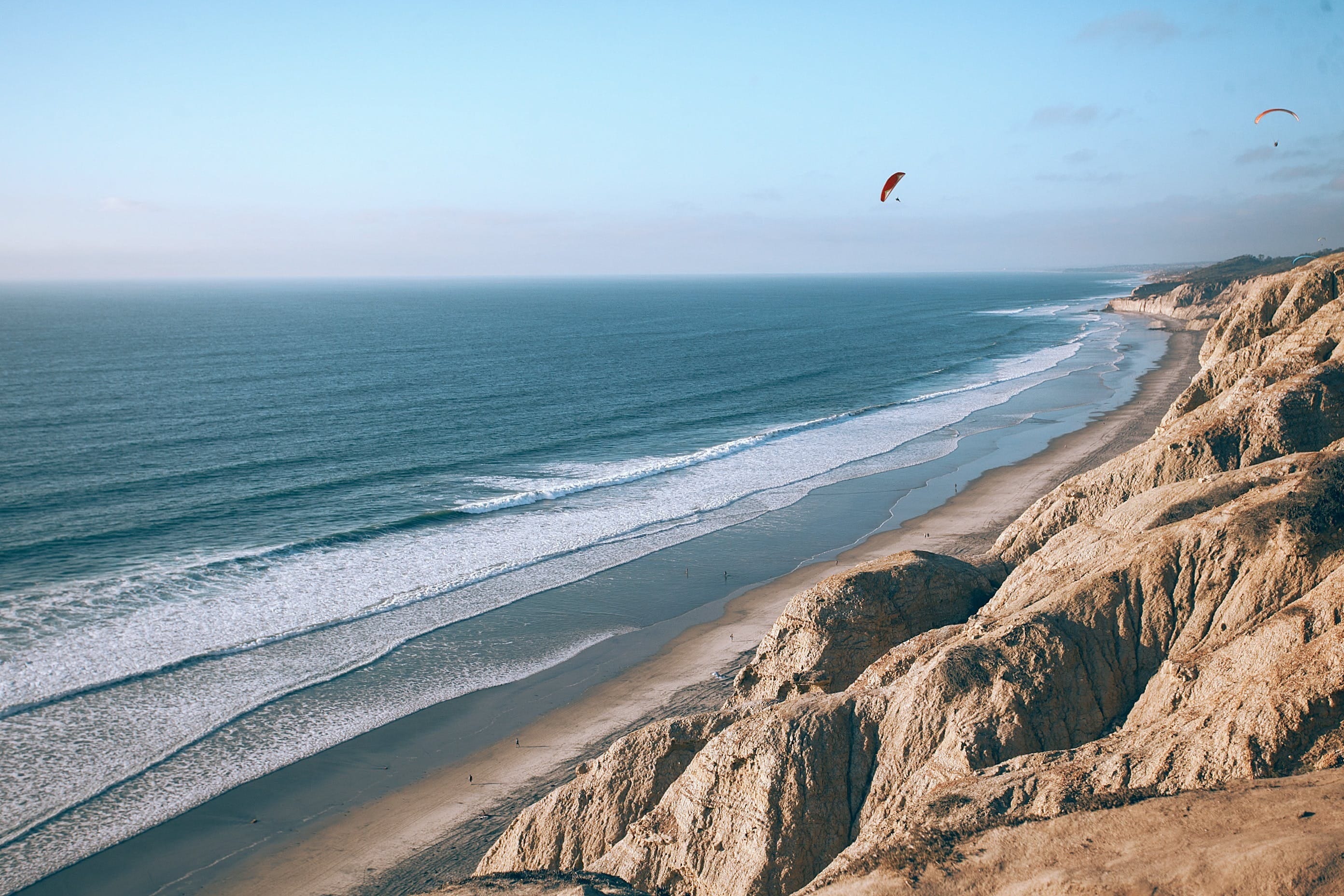
Global warming is shaking up the ocean, literally
Richard Aster
November 15, 2023
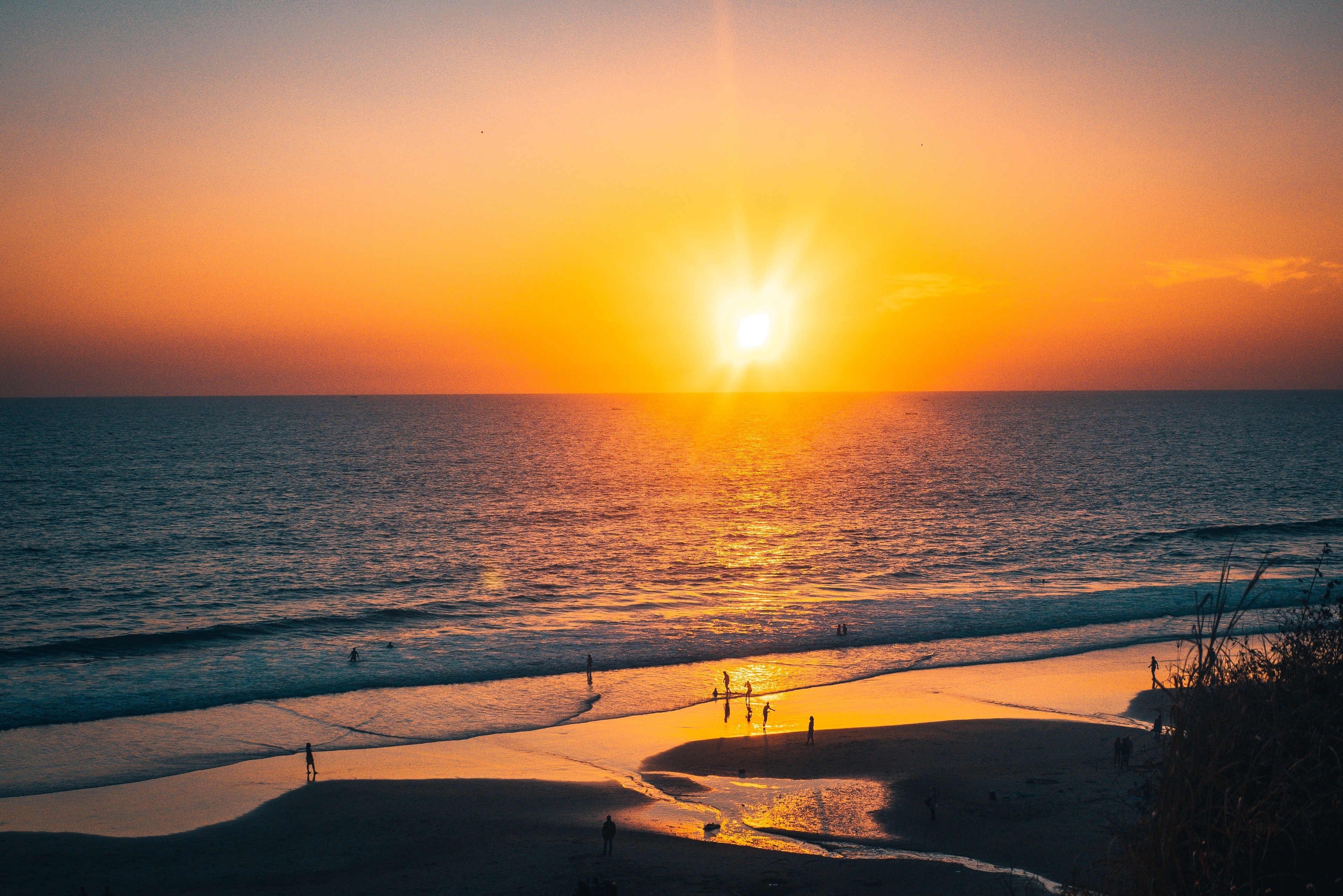
Is 2023 going to be the hottest year on record?
Ian Shine and Rebecca Geldard
November 14, 2023
ENCYCLOPEDIC ENTRY
A landslide is the movement of rock, earth, or debris down a sloped section of land.
Earth Science, Geology, Geography, Human Geography, Physical Geography
Loading ...

A landslide is the movement of rock , earth , or debris down a sloped section of land. Landslides are caused by rain , earthquakes , volcanoes , or other factors that make the slope unstable . Geologists , scientists who study the physical formations of Earth, sometimes describe landslides as one type of mass wasting . A mass wasting is any downward movement in which Earth's surface is worn away. Other types of mass wasting include rockfalls and the flow of shore deposits called alluvium . Near populated areas, landslides present major hazards to people and property. Landslides cause an estimated 25 to 50 deaths and $3.5 billion in damage each year in the United States.
What Causes Landslides?
Landslides have three major causes: geology , morphology , and human activity.
Geology refers to characteristics of the material itself. The earth or rock might be weak or fractured , or different layers may have different strength and stiffness.
Morphology refers to the structure of the land. For example, slopes that lose their vegetation to fire or drought are more vulnerable to landslides. Vegetation holds soil in place, and without the root systems of trees , bushes , and other plants , the land is more likely to slide away.
A classic morphological cause of landslides is erosion , or weakening of earth due to water. In April 1983, the U.S. town of Thistle, Utah, experienced a devastating landslide brought on by heavy rains and rapidly melting snow . A mass of earth eventually totaling 305 meters (1,000 feet) wide, 61 meters (200 feet) thick, and 1.6 kilometers (one mile) long slid across the nearby Spanish Fork River, damming it and severing railroad and highway lines. The landslide was the costliest in U.S. history, causing over $400 million in damage and destroying Thistle, which remains an evacuated ghost town today.
Human activity, such as agriculture and construction , can increase the risk of a landslide. Irrigation , deforestation , excavation , and water leakage are some of the common activities that can help destabilize, or weaken, a slope.
Types of Landslides
There are many ways to describe a landslide. The nature of a landslide's movement and the type of material involved are two of the most common.
Landslide Movement
There are several ways of describing how a landslide moves. These include falls , topples , translational slides , lateral spreads , and flows. In falls and topples, heavy blocks of material fall after separating from a very steep slope or cliff. Boulders tumbling down a slope would be a fall or topple. In translational slides, surface material is separated from the more stable underlying layer of a slope. An earthquake may shake the loosen top layer of soil from the harder earth beneath in this type of landslide. A lateral spread or flow is the movement of material sideways, or laterally. This happens when a powerful force, such as an earthquake, makes the ground move quickly, like a liquid.
Landslide Material
A landslide can involve rock, soil, vegetation, water, or some combination of all these. A landslide caused by a volcano can also contain hot volcanic ash and lava from the eruption . A landslide high in the mountains may have snow and snowmelt . Volcanic landslides, also called lahars , are among the most devastating type of landslides. The largest landslide in recorded history took place after the 1980 eruption of Mount St. Helens in the U.S. state of Washington. The resulting flow of ash, rock, soil, vegetation and water, with a volume of about 2.9 cubic kilometers (0.7 cubic miles), covered an area of 62 square kilometers (24 square miles).
Other Factors
Another factor that might be important for describing landslides is the speed of the movement. Some landslides move at many meters per second, while others creep along at an centimeter or two a year. The amount of water, ice , or air in the earth should also be considered. Some landslides include toxic gases from deep in Earth expelled by volcanoes. Some landslides, called mudslides , contain a high amount of water and move very quickly. Complex landslides consist of a combination of different material or movement types.
Martian Landslide In December 2008, scientists announced that they had found evidence of the largest landslide ever. Because of a giant asteroid impact billions of years ago, the smooth northern hemisphere of Mars is sharply separated from the irregular southern highlands. Arabia Terra, a previously unexplained plateau between the two regions, is thought to have been formed by an enormous landslide immediately after the impact. The land mass that slid north to form Arabia Terra was the size of the entire United States!
Media Credits
The audio, illustrations, photos, and videos are credited beneath the media asset, except for promotional images, which generally link to another page that contains the media credit. The Rights Holder for media is the person or group credited.
Illustrators
Educator reviewer, last updated.
April 26, 2024
User Permissions
For information on user permissions, please read our Terms of Service. If you have questions about how to cite anything on our website in your project or classroom presentation, please contact your teacher. They will best know the preferred format. When you reach out to them, you will need the page title, URL, and the date you accessed the resource.
If a media asset is downloadable, a download button appears in the corner of the media viewer. If no button appears, you cannot download or save the media.
Text on this page is printable and can be used according to our Terms of Service .
Interactives
Any interactives on this page can only be played while you are visiting our website. You cannot download interactives.
Related Resources
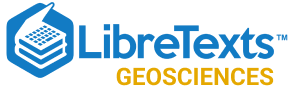
- school Campus Bookshelves
- menu_book Bookshelves
- perm_media Learning Objects
- login Login
- how_to_reg Request Instructor Account
- hub Instructor Commons
Margin Size
- Download Page (PDF)
- Download Full Book (PDF)
- Periodic Table
- Physics Constants
- Scientific Calculator
- Reference & Cite
- Tools expand_more
- Readability
selected template will load here
This action is not available.
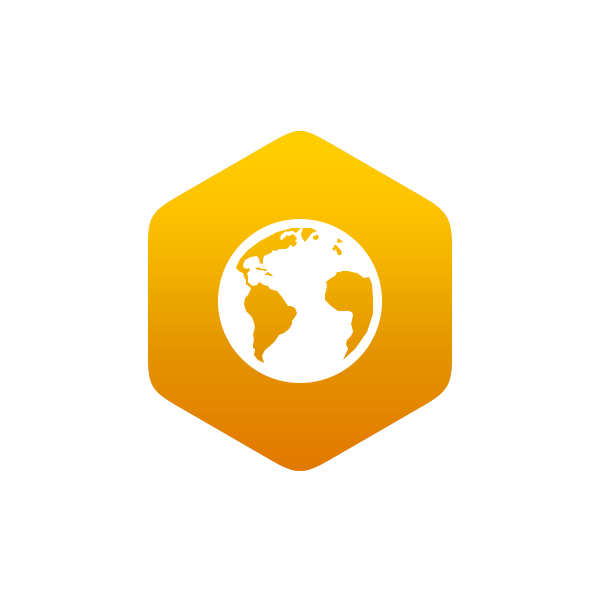
10.3: Landslide Classification and Identification
- Last updated
- Save as PDF
- Page ID 6904
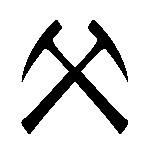
- Chris Johnson, Matthew D. Affolter, Paul Inkenbrandt, & Cam Mosher
- Salt Lake Community College via OpenGeology
\( \newcommand{\vecs}[1]{\overset { \scriptstyle \rightharpoonup} {\mathbf{#1}} } \)
\( \newcommand{\vecd}[1]{\overset{-\!-\!\rightharpoonup}{\vphantom{a}\smash {#1}}} \)
\( \newcommand{\id}{\mathrm{id}}\) \( \newcommand{\Span}{\mathrm{span}}\)
( \newcommand{\kernel}{\mathrm{null}\,}\) \( \newcommand{\range}{\mathrm{range}\,}\)
\( \newcommand{\RealPart}{\mathrm{Re}}\) \( \newcommand{\ImaginaryPart}{\mathrm{Im}}\)
\( \newcommand{\Argument}{\mathrm{Arg}}\) \( \newcommand{\norm}[1]{\| #1 \|}\)
\( \newcommand{\inner}[2]{\langle #1, #2 \rangle}\)
\( \newcommand{\Span}{\mathrm{span}}\)
\( \newcommand{\id}{\mathrm{id}}\)
\( \newcommand{\kernel}{\mathrm{null}\,}\)
\( \newcommand{\range}{\mathrm{range}\,}\)
\( \newcommand{\RealPart}{\mathrm{Re}}\)
\( \newcommand{\ImaginaryPart}{\mathrm{Im}}\)
\( \newcommand{\Argument}{\mathrm{Arg}}\)
\( \newcommand{\norm}[1]{\| #1 \|}\)
\( \newcommand{\Span}{\mathrm{span}}\) \( \newcommand{\AA}{\unicode[.8,0]{x212B}}\)
\( \newcommand{\vectorA}[1]{\vec{#1}} % arrow\)
\( \newcommand{\vectorAt}[1]{\vec{\text{#1}}} % arrow\)
\( \newcommand{\vectorB}[1]{\overset { \scriptstyle \rightharpoonup} {\mathbf{#1}} } \)
\( \newcommand{\vectorC}[1]{\textbf{#1}} \)
\( \newcommand{\vectorD}[1]{\overrightarrow{#1}} \)
\( \newcommand{\vectorDt}[1]{\overrightarrow{\text{#1}}} \)
\( \newcommand{\vectE}[1]{\overset{-\!-\!\rightharpoonup}{\vphantom{a}\smash{\mathbf {#1}}}} \)
Mass-wasting events are classified by type of movement and type of material, and there are several ways to classify these events. The figure and table show the terms used. In addition, mass-wasting types often share common morphological features observed on the surface, such as the head scarp—commonly seen as crescent shapes on a cliff face; hummocky or uneven surfaces; accumulations of talus—loose rocky material falling from above; and toe of the slope, which covers existing surface material.
Types of Mass Wasting
The most common mass-wasting types are falls, rotational and translational slides, flows, and creep [ 2 ]. Falls are abrupt rock movements that detach from steep slopes or cliffs. Rocks separate along existing natural breaks such as fractures or bedding planes. Movement occurs as free-falling, bouncing, and rolling. Falls are strongly influenced by gravity, mechanical weathering, and water. Rotational slides commonly show slow movement along a curved rupture surface. Translational slides often are rapid movements along a plane of distinct weakness between the overlying slide material and the more stable underlying material. Slides can be further subdivided into rock slides, debris slides, or earth slides depending on the type of the material involved (see table).
Table of Mass Wasting Types. Mass wasting movement type and primary earth material. Modified from [ 2 ; 3 ].
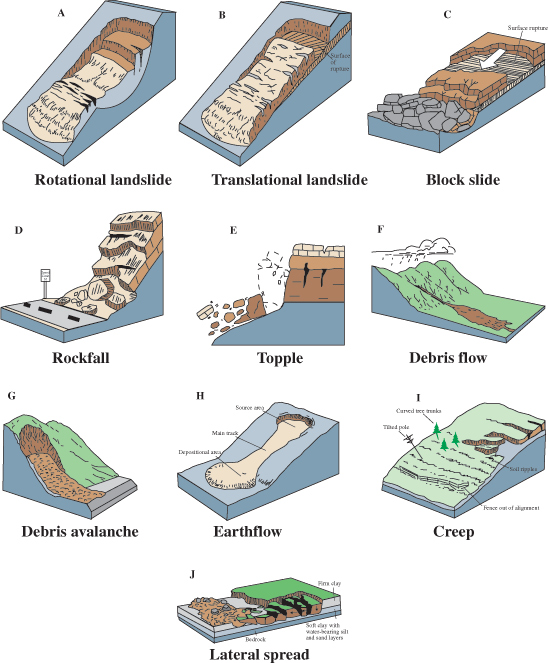
Examples of some of the types of landslides.
Flows are rapidly moving mass-wasting events in which the loose material is typically mixed with abundant water, creating long runouts at the slope base. Flows are commonly separated into debris flow (coarse material) and earthflow (fine material) depending on the type of material involved and the amount of water. Some of the largest and fastest flows on land are called sturzstroms , or long-runout landslides. They are still poorly understood but are known to travel for long distances, even in places without significant atmospheres like the Moon.
Creep is the imperceptibly slow downward movement of material caused by shear stress sufficient to produce permanent deformation in unconsolidated material [ 2 ]. Creep is indicated by curved tree trunks, bent fences or retaining walls, tilted poles or fences, and small soil ripples or ridges. A special type of soil creep is solifluction, which is the slow movement of soil lobes on low-angle slopes due to soil repeatedly freezing and thawing in high-latitude, typically sub-Arctic, Arctic, and Antarctic locations.
Landslide Hazards, David Applegate
Parts of a Landslide
Landslides have several identifying features that can be common across the different types of mass wasting. Note that there are many exceptions, and a landslide does not have to have these features. Displacement of material by landslides causes the absence of material uphill and the deposition of new material downhill, and careful observation can identify the evidence of that displacement. Other signs of landslides include tilted or offset structures or natural features that would normally be vertical or in place. Many landslides have escarpments or scarps. Landslide scarps, like fault scarps, are steep terrain created when the movement of the adjacent land exposes a part of the subsurface. The most prominent scarp is the main scarp, which marks the uphill extent of the landslide. As the disturbed material moves out of place, a step slope forms and develops a new hillside escarpment for the undisturbed material. Main scarps are formed by the movement of the displaced material away from the undisturbed ground and are the visible part of the slide rupture surface.
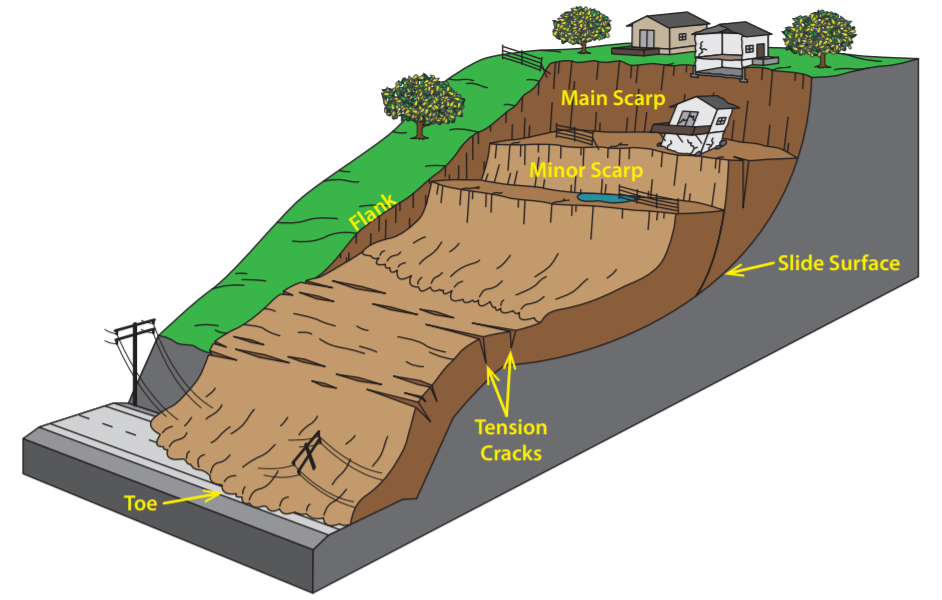
Diagram of a rotational landslide
For more information, visit http://opengeology.org/textbook/10-mass-wasting/#1032_Parts_of_a_Landslide
The slide rupture surface is the boundary of the body of the movement of the landslide. The geologic material below the slide surface does not move, and is marked on the sides by the flanks of the landslide and at the end by the toe of the landslide.
The toe of the landslide marks the end of the moving material. The toe marks the runout, or maximum distance traveled, of the landslide. In rotational landslides, the toe is often a large, disturbed mound of geologic material, forming as the landslide moves past its original rupture surface.
Rotational and translational landslides often have extensional cracks, sag ponds, hummocky terrain, and pressure ridges. Extensional cracks form when a landslide’s toe moves forward faster than the rest of landslide, resulting in tensional forces. Sag ponds are small bodies of water filling depressions formed where landslide movement has impounded drainage. Hummocky terrain is undulating and uneven topography that results from the ground being disturbed. Pressure ridges develop on the margins of the landslide where the material is forced upward into a ridge structure [ 4 ].
2. Highland, L. Landslide types and processes . (pubs.er.usgs.gov, 2004).
3. Hungr, O., Leroueil, S. & Picarelli, L. The Varnes classification of landslide types, an update. Landslides 11 , 167–194 (2013).
4. Highland, L. M. & Bobrowsky, P. The Landslide Handbook - A Guide to Understanding Landslides . 147 (U.S. Geological Survey, 2008).
Thank you for visiting nature.com. You are using a browser version with limited support for CSS. To obtain the best experience, we recommend you use a more up to date browser (or turn off compatibility mode in Internet Explorer). In the meantime, to ensure continued support, we are displaying the site without styles and JavaScript.
- View all journals
- Explore content
- About the journal
- Publish with us
- Sign up for alerts
- Review Article
- Published: 21 July 2020
Life and death of slow-moving landslides
- Pascal Lacroix ORCID: orcid.org/0000-0003-1282-9572 1 ,
- Alexander L. Handwerger ORCID: orcid.org/0000-0001-9235-3871 2 , 3 &
- Grégory Bièvre 1
Nature Reviews Earth & Environment volume 1 , pages 404–419 ( 2020 ) Cite this article
1986 Accesses
146 Citations
13 Altmetric
Metrics details
- Geomorphology
- Natural hazards
In the most destructive and catastrophic landslide events, rocks, soil and fluids can travel at speeds approaching several tens of metres per second. However, many landslides, commonly referred to as slow-moving landslides, creep at rates ranging from millimetres to several metres per year and can persist for years to decades. Although slow-moving landslides rarely claim lives, they can cause major damage to infrastructure and sometimes fail catastrophically, transitioning into fast-moving landslides that can result in thousands of casualties. In addition, slow-moving landslides are highly erosive features that control the landscape morphology in many mountainous regions (such as the California Coast Ranges or the Apennines). The persistent and long-term motion of slow-moving landslides provides an exceptional opportunity to investigate landslide processes and mechanisms. In this Review, we examine the environmental conditions (such as geology, climate and tectonics) of slow-moving-landslide-prone regions, analyse the forcings (for example, precipitation and groundwater, earthquakes, river erosion, anthropogenic forcings and external material supply) that drive their motion and investigate the subsequent implications of the different forcings on landslide dynamics. We then discuss circumstances in which slow-moving landslides can accelerate rapidly, move large distances or even fail catastrophically. Finally, we provide new perspectives and challenges for future landslide research.
Slow-moving landslides occur all around the world in mechanically weak rock and soil.
The persistent and long-term motion of slow-moving landslides provides an exceptional opportunity to investigate landslide processes and mechanisms.
The landslide velocity is modulated by external forcings (such as precipitation, earthquakes, material supply and anthropogenic activity).
Slow-moving landslides can sometimes accelerate rapidly and fail catastrophically.
This is a preview of subscription content, access via your institution
Access options
Access Nature and 54 other Nature Portfolio journals
Get Nature+, our best-value online-access subscription
24,99 € / 30 days
cancel any time
Subscribe to this journal
Receive 12 digital issues and online access to articles
92,52 € per year
only 7,71 € per issue
Buy this article
- Purchase on Springer Link
- Instant access to full article PDF
Prices may be subject to local taxes which are calculated during checkout
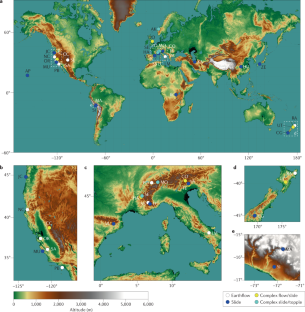
Similar content being viewed by others
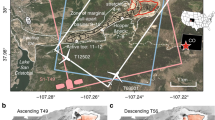
Four-dimensional surface motions of the Slumgullion landslide and quantification of hydrometeorological forcing
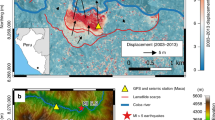
Rain and small earthquakes maintain a slow-moving landslide in a persistent critical state
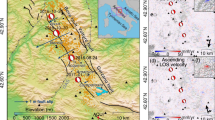
Triggering and recovery of earthquake accelerated landslides in Central Italy revealed by satellite radar observations
Froude, M. J. & Petley, D. Global fatal landslide occurrence from 2004 to 2016. Nat. Hazards Earth Syst. Sci. 18 , 2161–2181 (2018).
Google Scholar
Temple, P. H. & Rapp, A. Landslides in the Mgeta area, Western Uluguru mountains, Tanzania: Geomorphological effects of sudden heavy rainfall. Geogr. Ann. Ser. A Phys. Geogr. 54 , 157–193 (1972).
Keefer, D. K. Landslides caused by earthquakes. Geol. Soc. Am. Bull. 95 , 406–421 (1984).
Palmer, J. Creeping earth could hold secret to deadly landslides. Nature 548 , 384–386 (2017).
Mansour, M. F., Morgenstern, N. R. & Martin, C. D. Expected damage from displacement of slow-moving slides. Landslides 8 , 117–131 (2011).
Reid, M. E. et al. in Debris-Flow Hazards Mitigation: Mechanics, Prediction, and Assessment Vol. 1 (eds Rickenmann, D. & Chen, C.-L.) 155–166 (Millpress, 2003).
Booth, A. M. et al. Transient reactivation of a deep-seated landslide by undrained loading captured with repeat airborne and terrestrial lidar. Geophys. Res. Lett. 45 , 4841–4850 (2018).
Hendron, A. J. Jr & Patton, F. D. The Vaiont Slide, a geotechnical analysis based on how geologic observations of the failure surface. Technical Report GL-85-5 (U.S. Army Corps of Engineers, 1985).
Intrieri, E. et al. The Maoxian landslide as seen from space: detecting precursors of failure with Sentinel-1 data. Landslides 15 , 123–133 (2018).
Handwerger, A. L., Huang, M.-H., Fielding, E. J., Booth, A. M. & Bürgmann, R. A shift from drought to extreme rainfall drives a stable landslide to catastrophic failure. Sci. Rep. 9 , 1569 (2019).
Federico, A. et al. Prediction of time to slope failure: a general framework. Environ. Earth Sci. 66 , 245–256 (2012).
Carlà, T. et al. Perspectives on the prediction of catastrophic slope failures from satellite InSAR. Sci. Rep. 9 , 14137 (2019).
Lacroix, P., Bièvre, G., Pathier, E., Kniess, U. & Jongmans, D. Use of Sentinel-2 images for the detection of precursory motions before landslide failures. Remote. Sens. Environ. 215 , 507–516 (2018).
Desrues, M., Lacroix, P. & Brenguier, O. Satellite pre-failure detection and in situ monitoring of the landslide of the Tunnel du Chambon, French Alps. Geosciences 9 , 313 (2019).
Wang, F. et al. Movement of the Shuping landslide in the first four years after the initial impoundment of the Three Gorges Dam Reservoir, China. Landslides 5 , 321–329 (2008).
Dille, A. et al. Causes and triggers of deep-seated hillslope instability in the tropics–Insights from a 60-year record of Ikoma landslide (DR Congo). Geomorphology 345 , 106835 (2019).
Nappo, N., Peduto, D., Mavrouli, O., van Westen, C. J. & Gullà, G. Slow-moving landslides interacting with the road network: analysis of damage using ancillary data, in situ surveys and multi-source monitoring data. Eng. Geol. 260 , 105244 (2019).
Lacroix, P., Dehecq, A. & Taipe, E. Irrigation-triggered landslides in a Peruvian desert caused by modern intensive farming. Nat. Geosci. 13 , 56–60 (2020).
Wu, S. et al. Zonation of the landslide hazards in the forereservoir region of the Three Gorges Project on the Yangtze River. Eng. Geol. 59 , 51–58 (2001).
Mackey, B. H. & Roering, J. J. Sediment yield, spatial characteristics, and the long-term evolution of active earthflows determined from airborne lidar and historical aerial photographs, Eel River, California. Bulletin 123 , 1560–1576 (2011).
Simoni, A., Ponza, A., Picotti, V., Berti, M. & Dinelli, E. Earthflow sediment production and Holocene sediment record in a large Apennine catchment. Geomorphology 188 , 42–53 (2013).
Coe, J. A., McKenna, J. P., Godt, J. W. & Baum, R. L. Basal-topographic control of stationary ponds on a continuously moving landslide. Earth Surf. Process. Landf. 34 , 264–279 (2009).
Bontemps, N., Lacroix, P., Larose, E., Jara, J. & Taipe, E. Rain and small earthquakes maintain a slow-moving landslide in a persistent critical state. Nat. Commun. 11 , 780 (2020).
Nereson, A. L. & Finnegan, N. J. Drivers of earthflow motion revealed by an 80 yr record of displacement from Oak Ridge earthflow, Diablo Range, California, USA. Bulletin 131 , 389–402 (2018).
Iverson, R. M. & Major, J. J. Rainfall, ground-water flow, and seasonal movement at Minor Creek landslide, northwestern California: physical interpretation of empirical relations. Geol. Soc. Am. Bull. 99 , 579–594 (1987).
Krzeminska, D., Bogaard, T., Malet, J.-P. & Van Beek, L. A model of hydrological and mechanical feedbacks of preferential fissure flow in a slow-moving landslide. Hydrol. Earth Syst. Sci. 17 , 947–959 (2013).
Hungr, O., Leroueil, S. & Picarelli, L. The Varnes classification of landslide types, an update. Landslides 11 , 167–194 (2013).
Galloway, W. The landslide in the Rhymney valley. Nature 73 , 425–426 (1906).
Miller, W. J. The landslide at Point Firmin, California. Sci. Monthly 32 , 464–469 (1931).
Benson, W. N. Landslides and their relation to engineering in the Dunedin district, New Zealand. Econ. Geol. 41 , 328–347 (1946).
Crandell, D. R. Movement of the Slumgullion earthflow near Lake City, Colorado. Short Papers in the Geologic and Hydrologic Sciences B136–B139 (1961).
Schulz, W. H., McKenna, J. P., Kibler, J. D. & Biavati, G. Relations between hydrology and velocity of a continuously moving landslide — evidence of pore-pressure feedback regulating landslide motion? Landslides 6 , 181–190 (2009).
Schulz, W. H., Kean, J. W. & Wang, G. Landslide movement in southwest Colorado triggered by atmospheric tides. Nat. Geosci. 2 , 863–866 (2009).
Schulz, W. H. et al. Landslide kinematics and their potential controls from hourly to decadal timescales: Insights from integrating ground-based InSAR measurements with structural maps and long-term monitoring data. Geomorphology 285 , 121–136 (2017).
Schulz, W. H., Smith, J. B., Wang, G., Jiang, Y. & Roering, J. J. Clayey landslide initiation and acceleration strongly modulated by soil swelling. Geophys. Res. Lett. 45 , 1888–1896 (2018).
Helmstetter, A. & Garambois, S. Seismic monitoring of Séchilienne rockslide (French Alps): Analysis of seismic signals and their correlation with rainfalls. J. Geophys. Res. Earth Surf. 115 , F03016 (2010).
Mainsant, G. et al. Ambient seismic noise monitoring of a clay landslide: Toward failure prediction. J. Geophys. Res. Earth Surf. 117 , F01030 (2012).
Travelletti, J., Sailhac, P., Malet, J.-P., Grandjean, G. & Ponton, J. Hydrological response of weathered clay-shale slopes: Water infiltration monitoring with time-lapse electrical resistivity tomography. Hydrol. Process. 26 , 2106–2119 (2012).
Provost, F., Hibert, C. & Malet, J.-P. Automatic classification of endogenous landslide seismicity using the Random Forest supervised classifier. Geophys. Res. Lett. 44 , 113–120 (2017).
Delacourt, C., Allemand, P., Casson, B. & Vadon, H. Velocity field of the “La Clapière” landslide measured by the correlation of aerial and QuickBird satellite images. Geophys. Res. Lett. 31 , L15619 (2004).
Hilley, G. E., Bürgmann, R., Ferretti, A., Novali, F. & Rocca, F. Dynamics of slow-moving landslides from permanent scatterer analysis. Science 304 , 1952–1955 (2004).
Booth, A. M., Lamb, M. P., Avouac, J.-P. & Delacourt, C. Landslide velocity, thickness, and rheology from remote sensing: La Clapière landslide, France. Geophys. Res. Lett. 40 , 4299–4304 (2013).
Lacroix, P., Berthier, E. & Maquerhua, E. T. Earthquake-driven acceleration of slow-moving landslides in the Colca valley, Peru, detected from Pléiades images. Remote. Sens. Environ. 165 , 148–158 (2015).
Lacroix, P., Araujo, G., Hollingsworth, J. & Taipe, E. Self entrainment motion of a slow-moving landslide inferred from Landsat-8 time-series. J. Geophys. Res. Earth Surf. 124 , 1201–1216 (2019).
Bennett, G. L. et al. Historic drought puts the brakes on earthflows in Northern California. Geophys. Res. Lett. 43 , 5725–5731 (2016).
Stumpf, A., Malet, J.-P. & Delacourt, C. Correlation of satellite image time-series for the detection and monitoring of slow-moving landslides. Remote. Sens. Environ. 189 , 40–55 (2017).
Handwerger, A. L. et al. Widespread initiation, reactivation, and acceleration of landslides in the northern California Coast Ranges due to extreme rainfall. J. Geophys. Res. Earth Surf. 124 , 1782–1797 (2019).
Hutchinson, J. & Bhandari, R. Undrained loading, a fundamental mechanism of mudflows and other mass movements. Geotechnique 21 , 353–358 (1971).
Van Asch, T. W. J., Malet, J.-P. & Bogaard, T. A. The effect of groundwater fluctuations on the velocity pattern of slow-moving landslides. Nat. Hazards Earth Syst. Sci. 9 , 739–749 (2009).
Lacroix, P., Perfettini, H., Taipe, E. & Guillier, B. Coseismic and postseismic motion of a landslide: Observations, modeling, and analogy with tectonic faults. Geophys. Res. Lett. 41 , 6676–6680 (2014).
Handwerger, A. L., Rempel, A. W., Skarbek, R. M., Roering, J. J. & Hilley, G. E. Rate-weakening friction characterizes both slow sliding and catastrophic failure of landslides. Proc. Natl Acad. Sci. USA 113 , 10281–10286 (2016).
RESIF/OMIV. French multidisciplinary observatory of versant instabilities. RESIF - Réseau Sismologique et géodésique Français (RESIF/OMIV, 2006).
Colombo, A., Lanteri, L., Ramasco, M. & Troisi, C. Systematic GIS-based landslide inventory as the first step for effective landslide-hazard management. Landslides 2 , 291–301 (2005).
Jaboyedoff, M. et al. in Landslides Processes — from Geomorphologic Mapping to Dynamic Modeling (eds Malet, J. P., Remaître, A. & Bogaard, T.) 131–137 (CERG Editions, 2009).
Malet, J.-P., Maquaire, O., Locat, J. & Remaître, A. Assessing debris flow hazards associated with slow moving landslides: methodology and numerical analyses. Landslides 1 , 83–90 (2004).
Corsini, A., Pasuto, A., Soldati, M. & Zannoni, A. Field monitoring of the Corvara landslide (Dolomites, Italy) and its relevance for hazard assessment. Geomorphology 66 , 149–165 (2005).
Bièvre, G. et al. Paleotopographic control of landslides in lacustrine deposits (Trièves plateau, French western Alps). Geomorphology 125 , 214–224 (2011).
Lebourg, T., Binet, S., Tric, E., Jomard, H. & El Bedoui, S. Geophysical survey to estimate the 3D sliding surface and the 4D evolution of the water pressure on part of a deep seated landslide. Terra Nova 17 , 399–406 (2005).
Strozzi, T. et al. Combined observations of rock mass movements using satellite SAR interferometry, differential GPS, airborne digital photogrammetry, and airborne photography interpretation. J. Geophys. Res. Earth Surf. 115 , F01014 (2010).
Meric, O. et al. Application of geophysical methods for the investigation of the large gravitational mass movement of Séchilienne, France. Can. Geotech. J. 42 , 1105–1115 (2005).
Corominas, J., Moya, J., Ledesma, A., Lloret, A. & Gili, J. A. Prediction of ground displacements and velocities from groundwater level changes at the Vallcebre landslide (Eastern Pyrenees, Spain). Landslides 2 , 83–96 (2005).
Mantovani, F., Pasuto, A., Silvano, S. & Zannoni, A. Collecting data to define future hazard scenarios of the Tessina landslide. Int. J. Appl. Earth Obs. Geoinf. 2 , 33–40 (2000).
Merriam, R. Portuguese bend landslide, Palos Verdes Hills, California. J. Geol. 68 , 140–153 (1960).
Scheingross, J. S. et al. Fault-zone controls on the spatial distribution of slow-moving landslides. Bulletin 125 , 473–489 (2013).
Roering, J. J. et al. Beyond the angle of repose: A review and synthesis of landslide processes in response to rapid uplift, Eel River, Northern California. Geomorphology 236 , 109–131 (2015).
Nereson, A. L., Davila Olivera, S. & Finnegan, N. J. Field and remote-sensing evidence for hydro-mechanical isolation of a long-lived earthflow in central California. Geophys. Res. Lett. 45 , 9672–9680 (2018).
Schulz, W. H. & Wang, G. Residual shear strength variability as a primary control on movement of landslides reactivated by earthquake-induced ground motion: Implications for coastal Oregon, U.S. J. Geophys. Res. Earth Surf. 119 , 1617–1635 (2014).
Coe, J. A. et al. Seasonal movement of the Slumgullion landslide determined from Global Positioning System surveys and field instrumentation, July 1998–March 2002. Eng. Geol. 68 , 67–101 (2003).
Zhang, X., Phillips, C. & Pearce, A. Surface movement in an earthflow complex, Raukumara Peninsula, New Zealand. Geomorphology 4 , 261–272 (1991).
Massey, C., Petley, D., McSaveney, M. & Archibald, G. Basal sliding and plastic deformation of a slow, reactivated landslide in New Zealand. Eng. Geol. 208 , 11–28 (2016).
Macfarlane, D. F. Observations and predictions of the behaviour of large, slow-moving landslides in schist, Clyde Dam reservoir, New Zealand. Eng. Geol. 109 , 5–15 (2009).
Furuya, G., Sassa, K., Hiura, H. & Fukuoka, H. Mechanism of creep movement caused by landslide activity and underground erosion in crystalline schist, Shikoku Island, southwestern Japan. Eng. Geol. 53 , 311–325 (1999).
Peyret, M. et al. Monitoring of the large slow Kahrod landslide in Alborz mountain range (Iran) by GPS and SAR interferometry. Eng. Geol. 100 , 131–141 (2008).
Iverson, R. M. A constitutive equation for mass-movement behavior. J. Geol. 93 , 143–160 (1985).
Malet, J.-P. & Maquaire, O. in Proceedings of 1st International Conference on Fast Slope Movements (eds Malet, J.-P., Maquaire, O. & Picarelli, L.) 333–340 (Patron Editore, 2003).
Agliardi, F., Scuderi, M. M., Fusi, N. & Collettini, C. Slow-to-fast transition of giant creeping rockslides modulated by undrained loading in basal shear zones. Nat. Commun. 11 , 1352 (2020).
Glueer, F., Loew, S., Manconi, A. & Aaron, J. From toppling to sliding: progressive evolution of the Moosfluh Landslide, Switzerland. J. Geophys. Res. Earth Surf. 124 , 2899–2919 (2020).
El Bedoui, S., Guglielmi, Y., Lebourg, T. & Pérez, J.-L. Deep-seated failure propagation in a fractured rock slope over 10,000 years: the La Clapiere slope, the south-eastern French Alps. Geomorphology 105 , 232–238 (2009).
Le Roux, O. et al. CRE dating on the head scarp of a major landslide (Séchilienne, French Alps), age constraints on Holocene kinematics. Earth Planet. Sci. Lett. 280 , 236–245 (2009).
Guerriero, L. et al. Kinematic segmentation and velocity in earth flows: a consequence of complex basal-slip surfaces. Procedia Earth Planet. Sci. 16 , 146–155 (2016).
Zerathe, S. et al. Morphology, structure and kinematics of a rainfall controlled slow-moving Andean landslide, Peru. Earth Surf. Process. Landf. 41 , 1477–1493 (2016).
Bontemps, N., Lacroix, P. & Doin, M. Inversion of deformation fields time-series from optical images, and application to the long term kinematics of slow-moving landslides in Peru. Remote. Sens. Environ. 210 , 144–158 (2018).
Van Genuchten, P. M. & De Rijke, H. On pore water pressure variations causing slide velocities and accelerations observed in a seasonally active landslide. Earth Surf. Process. Landf. 14 , 577–586 (1989).
Handwerger, A. L., Roering, J. J. & Schmidt, D. A. Controls on the seasonal deformation of slow-moving landslides. Earth Planet. Sci. Lett. 377 , 239–247 (2013).
Malet, J.-P., Maquaire, O. & Calais, E. The use of Global Positioning System techniques for the continuous monitoring of landslides: application to the Super-Sauze earthflow (Alpes-de-Haute-Provence, France). Geomorphology 43 , 33–54 (2002).
Li, X., Zhao, C., Hölter, R., Datcheva, M. & Alimardani Lavasan, A. Modelling of a large landslide problem under water level fluctuation — model calibration and verification. Geosciences 9 , 89 (2019).
Iverson, R. M. Landslide triggering by rain infiltration. Water Resour. Res. 36 , 1897–1910 (2000).
Aleotti, P. A warning system for rainfall-induced shallow failures. Eng. Geol. 73 , 247–265 (2004).
Matsuura, S., Asano, S. & Okamoto, T. Relationship between rain and/or meltwater, pore-water pressure and displacement of a reactivated landslide. Eng. Geol. 101 , 49–59 (2008).
Bayer, B., Simoni, A., Mulas, M., Corsini, A. & Schmidt, D. Deformation responses of slow moving landslides to seasonal rainfall in the Northern Apennines, measured by InSAR. Geomorphology 308 , 293–306 (2018).
Osawa, H. et al. Seasonal transition of hydrological processes in a slow-moving landslide in a snowy region. Hydrol. Process. 32 , 2695–2707 (2018).
Zhou, C., Yin, K., Cao, Y. & Ahmed, B. Application of time series analysis and PSO–SVM model in predicting the Bazimen landslide in the Three Gorges Reservoir, china. Eng. Geol. 204 , 108–120 (2016).
Zhang, X., Phillips, C. & Marden, M. A comparison of earthflow movement mechanisms on forested and grassed slopes, Raukumara Peninsula, North Island, New Zealand. Geomorphology 6 , 175–187 (1993).
Terzaghi, K. Theoretical Soil Mechanics (Wiley, 1943).
Keefer, D. K. & Johnson, A. M. Earth flows; morphology, mobilization, and movement. Professional Paper 1264 (U.S. Government Publishing Office, 1983).
Picarelli, L., Urciuoli, G., Ramondini, M. & Comegna, L. Main features of mudslides in tectonised highly fissured clay shales. Landslides 2 , 15–30 (2005).
Fiolleau, S. et al. Seismic characterization of a clay-block rupture in Harmalière landslide, French Western Alps. Geophys. J. Int. 221 , 1777–1788 (2020).
Lu, N. & Godt, J. Infinite slope stability under steady unsaturated seepage conditions. Water Resour. Res. 44 , W11404 (2008).
Lu, N. & Godt, J. W. Hillslope Hydrology and Stability (Cambridge Univ. Press, 2013).
Iverson, R. M. et al. Acute sensitivity of landslide rates to initial soil porosity. Science 290 , 513–516 (2000).
Van Asch, T. W. J., Hendriks, M. R., Hessel, R. & Rappange, F. E. Hydrological triggering conditions of landslides in varved clays in the French Alps. Eng. Geol. 42 , 239–251 (1996).
Van Asch, T. J. W., Buma, J. & Van Beek, L. P. H. A view on some hydrological triggering systems in landslides. Geomorphology 30 , 25–32 (1999).
Hu, X. et al. Mobility, thickness, and hydraulic diffusivity of the slow-moving Monroe landslide in California revealed by L-band satellite radar interferometry. J. Geophys. Res. Solid. Earth 124 , 7504–7518 (2019).
Bièvre, G., Jongmans, D., Winiarski, T. & Zumbo, V. Application of geophysical measurements for assessing the role of fissures in water infiltration within a clay landslide (Trièves area, French Alps). Hydrol. Process. 26 , 2128–2142 (2012).
Krzeminska, D. M., Bogaard, T. A., van Asch, T. W. J. & van Beek, L. P. H. A conceptual model of the hydrological influence of fissures on landslide activity. Hydrol. Earth Syst. Sci. 16 , 1561–1576 (2012).
Bièvre, G. et al. Influence of environmental parameters on the seismic velocity changes in a clayey mudflow (Pont-Bourquin Landslide, Switzerland). Eng. Geol. 245 , 248–257 (2018).
Helmstetter, A. et al. Slider block friction model for landslides: Application to Vaiont and La Clapiere landslides. J. Geophys. Res. Solid Earth 109 , B02409 (2004).
Du, J., Yin, K. & Lacasse, S. Displacement prediction in colluvial landslides, Three Gorges Reservoir, China. Landslides 10 , 203–218 (2013).
Miao, H., Wang, G., Yin, K., Kamai, T. & Li, Y. Mechanism of the slow-moving landslides in Jurassic red-strata in the Three Gorges Reservoir, China. Eng. Geol. 171 , 59–69 (2014).
Tofani, V., Dapporto, S., Vannocci, P. & Casagli, N. Infiltration, seepage and slope instability mechanisms during the 20–21 November 2000 rainstorm in Tuscany, central Italy. Nat. Hazards Earth Syst. Sci. 6 , 1025–1033 (2006).
Keqiang, H., Xiangran, L., Xueqing, Y. & Dong, G. The landslides in the Three Gorges Reservoir Region, China and the effects of water storage and rain on their stability. Environ. Geol. 55 , 55–63 (2008).
Hu, X., Lu, Z., Pierson, T. C., Kramer, R. & George, D. L. Combining InSAR and GPS to determine transient movement and thickness of a seasonally active low-gradient translational landslide. Geophys. Res. Lett. 45 , 1453–1462 (2018).
Bièvre, G., Joseph, A. & Bertrand, C. Preferential water infiltration path in a slow-moving clayey earthslide evidenced by cross-correlation of hydrometeorological time series (Charlaix landslide, French Western Alps). Geofluids 2018 , 9593267 (2018). Special issue “The Role and Impact of Geofluids in Geohazards” .
Keefer, D. K. et al. Real-time landslide warning during heavy rainfall. Science 238 , 921–925 (1987).
Krøgli, I. K. et al. The Norwegian forecasting and warning service for rainfall- and snowmelt-induced landslides. Nat. Hazards Earth Syst. Sci. 18 , 1427–1450 (2018).
Berti, M. & Simoni, A. Observation and analysis of near-surface pore-pressure measurements in clay-shales slopes. Hydrol. Process. 26 , 2187–2205 (2012).
Preuth, T., Glade, T. & Demoulin, A. Stability analysis of a human-influenced landslide in eastern Belgium. Geomorphology 120 , 38–47 (2010).
Calabro, M. D., Schmidt, D. A. & Roering, J. J. An examination of seasonal deformation at the Portuguese Bend landslide, southern California, using radar interferometry. J. Geophys. Res. Earth Surf. 115 , F02020 (2010).
Helle, T. E., Nordal, S., Aagaard, P. & Lied, O. K. Long-term effect of potassium chloride treatment on improving the soil behavior of highly sensitive clay — Ulvensplitten, Norway. Can. Geotech. J. 53 , 410–422 (2016).
Helle, T. E., Aagaard, P. & Nordal, S. In situ improvement of highly sensitive clays by potassium chloride migration. J. Geotech. Geoenviron. Eng. 143 , 04017074 (2018).
Bardou, E., Bowen, P., Boivin, P. & Banfill, P. Impact of small amounts of swelling clays on the physical properties of debris-flow-like granular materials. Implications for the study of alpine debris flow. Earth Surf. Process. Landf. 32 , 698–710 (2007).
Torrance, J. K. in Landslides: Types, Mechanisms and Modeling Ch. 8 (eds Clague, J. J. & Stead, D.) 83–94 (Cambridge Univ. Press, 2012).
Tika, T. E., Vaughan, P. & Lemos, L. Fast shearing of pre-existing shear zones in soil. Geotechnique 46 , 197–233 (1996).
Scaringi, G., Hu, W., Xu, Q. & Huang, R. Shear-rate-dependent behavior of clayey bimaterial interfaces at landslide stress levels. Geophys. Res. Lett. 45 , 766–777 (2018).
Molinari, A. & Perfettini, H. Fundamental aspects of a new micromechanical model of rate and state friction. J. Mech. Phys. Solids 124 , 63–82 (2019).
Brodsky, E. E., Roeloffs, E., Woodcock, D., Gall, I. & Manga, M. A mechanism for sustained groundwater pressure changes induced by distant earthquakes. J. Geophys. Res. Solid Earth 108 , 2390 (2003).
Wang, C.-y & Chia, Y. Mechanism of water level changes during earthquakes: Near field versus intermediate field. Geophys. Res. Lett. 35 , L12402 (2008).
Sassa, K., Fukuoka, H., Scarascia-Mugnozza, G. & Evans, S. Earthquake-induced-landslides: distribution, motion and mechanisms. Soils Found. 36 , 53–64 (1996).
Wang, F., Sassa, K. & Wang, G. Mechanism of a long-runout landslide triggered by the August 1998 heavy rainfall in Fukushima Prefecture, Japan. Eng. Geol. 63 , 169–185 (2002).
Glueer, F., Loew, S. & Manconi, A. Paraglacial history and structure of the Moosfluh Landslide (1850–2016), Switzerland. Geomorphology 355 , 106677 (2020).
Carrière, S. et al. Rheological properties of clayey soils originating from flow-like landslides. Landslides 15 , 1615–1630 (2018).
Newmark, N. M. Effects of earthquakes on dams and embankments. Geotechnique 15 , 139–159 (1965).
Marc, O., Hovius, N., Meunier, P., Uchida, T. & Hayashi, S. Transient changes of landslide rates after earthquakes. Geology 43 , 883–886 (2015).
Durand, V. et al. On the link between external forcings and slope instabilities in the Piton de la Fournaise Summit Crater, Reunion Island. J. Geophys. Res. Earth Surf. 123 , 2422–2442 (2018).
Bovis, M. J. & Jones, P. Holocene history of earthflow mass movements in south-central British Columbia: the influence of hydroclimatic changes. Can. J. Earth Sci. 29 , 1746–1755 (1992).
Rutter, E. & Green, S. Quantifying creep behaviour of clay-bearing rocks below the critical stress state for rapid failure: Mam Tor landslide, Derbyshire, England. J. Geol. Soc. 168 , 359–372 (2011).
Riva, F., Agliardi, F., Amitrano, D. & Crosta, G. B. Damage-based time-dependent modeling of paraglacial to postglacial progressive failure of large rock slopes. J. Geophys. Res. Earth Surf. 123 , 124–141 (2018).
Moore, P. L. & Iverson, N. R. Slow episodic shear of granular materials regulated by dilatant strengthening. Geology 30 , 843–846 (2002).
Gabet, E. J. & Mudd, S. M. The mobilization of debris flows from shallow landslides. Geomorphology 74 , 207–218 (2006).
Iverson, R. M. Regulation of landslide motion by dilatancy and pore pressure feedback. J. Geophys. Res. Earth Surf. 110 , F02015 (2005).
Iverson, R. M. & George, D. L. Modelling landslide liquefaction, mobility bifurcation and the dynamics of the 2014 Oso disaster. Géotechnique 66 , 175–187 (2016).
Khaldoun, A. et al. Quick clay and landslides of clayey soils. Phys. Rev. Lett. 103 , 188301 (2009).
Voight, B. A method for prediction of volcanic eruptions. Nature 332 , 125–130 (1988).
Lacroix, P. & Amitrano, D. Long-term dynamics of rockslides and damage propagation inferred from mechanical modeling. J. Geophys. Res. Earth Surf. 118 , 2292–2307 (2013).
Viesca, R. C. & Rice, J. R. Nucleation of slip-weakening rupture instability in landslides by localized increase of pore pressure. J. Geophys. Res. Solid. Earth 117 , B03104 (2012).
Schaeffer, D. G. & Iverson, R. M. Steady and intermittent slipping in a model of landslide motion regulated by pore-pressure feedback. SIAM J. Appl. Math. 69 , 769–786 (2008).
Collins, B. D. & Reid, M. E. Enhanced landslide mobility by basal liquefaction: The 2014 State Route 530 (Oso), Washington, landslide. GSA Bulletin 132 , 451–476 (2020).
Chau, K. T. Onset of natural terrain landslides modelled by linear stability analysis of creeping slopes with a two-state variable friction law. Int. J. Numer. Anal. Methods Geomech. 23 , 1835–1855 (1999).
Dieterich, J. H. Modeling of rock friction: 1. Experimental results and constitutive equations. J. Geophys. Res. Solid Earth 84 , 2161–2168 (1979).
Rice, J. R. & Ruina, A. L. Stability of steady frictional slipping. J. Appl. Mech. 50 , 343–349 (1983).
Wang, G., Suemine, A. & Schulz, W. H. Shear-rate-dependent strength control on the dynamics of rainfall-triggered landslides, Tokushima Prefecture, Japan. Earth Surf. Process. Landf. 35 , 407–416 (2010).
Segall, P. & Rice, J. R. Dilatancy, compaction, and slip instability of a fluid-infiltrated fault. J. Geophys. Res. Solid Earth 100 , 22155–22171 (1995).
Samuelson, J., Elsworth, D. & Marone, C. Shear-induced dilatancy of fluid-saturated faults: experiment and theory. J. Geophys. Res. Solid Earth 114 , B12404 (2009).
Segall, P., Rubin, A. M., Bradley, A. M. & Rice, J. R. Dilatant strengthening as a mechanism for slow slip events. J. Geophys. Res. Solid. Earth 115 , B12305 (2010).
Fukuzono, T. A method to predict the time of slope failure caused by rainfall using the inverse number of velocity of surface displacement. Landslides 22 , 8–13_1 (1985).
Amitrano, D., Grasso, J. R. & Senfaute, G. Seismic precursory patterns before a cliff collapse and critical point phenomena. Geophys. Res. Lett. 32 , L08314 (2005).
Kilburn, C. R. & Petley, D. N. Forecasting giant, catastrophic slope collapse: lessons from Vajont, Northern Italy. Geomorphology 54 , 21–32 (2003).
Crosta, G. & Agliardi, F. Failure forecast for large rock slides by surface displacement measurements. Can. Geotech. J. 40 , 176–191 (2003).
Rose, N. D. & Hungr, O. Forecasting potential slope failure in open pit mines–contingency planning and remediation. Int. J. Rock. Mech. Min. Sci. 44 , 308–320 (2007).
Segalini, A., Valletta, A. & Carri, A. Landslide time-of-failure forecast and alert threshold assessment: a generalized criterion. Eng. Geol. 245 , 72–80 (2018).
Poli, P. Creep and slip: Seismic precursors to the Nuugaatsiaq landslide (Greenland). Geophys. Res. Lett. 44 , 8832–8836 (2017).
Bell, A. F. Predictability of landslide timing from quasi-periodic precursory earthquakes. Geophys. Res. Lett. 45 , 1860–1869 (2018).
Fan, X. et al. Failure mechanism and kinematics of the deadly June 24th 2017 Xinmo landslide, Maoxian, Sichuan, China. Landslides 14 , 2129–2146 (2017).
Skarbek, R. M., Rempel, A. W. & Schmidt, D. A. Geologic heterogeneity can produce aseismic slip transients. Geophys. Res. Lett. 39 , L21306 (2012).
Bell, A. F., Naylor, M., Heap, M. J. & Main, I. G. Forecasting volcanic eruptions and other material failure phenomena: an evaluation of the failure forecast method. Geophys. Res. Lett. 38 , L15304 (2011).
Lipovsky, B. P. & Dunham, E. M. Slow-slip events on the Whillans Ice Plain, Antarctica, described using rate-and-state friction as an ice stream sliding law. J. Geophys. Res. Earth Surf. 122 , 973–1003 (2017).
Minchew, B. M. & Meyer, C. R. Dilation of subglacial sediment governs incipient surge motion in glaciers with deformable beds. Proc. R. Soc. A Math. Phys. Eng. Sci. 476 , 20200033 (2020).
Gomberg, J., Bodin, P., Savage, W. & Jackson, M. E. Landslide faults and tectonic faults, analogs?: The Slumgullion earthflow, Colorado. Geology 23 , 41–44 (1995).
Dmitrieva, K., Hotovec-Ellis, A. J., Prejean, S. & Dunham, E. M. Frictional-faulting model for harmonic tremor before redoubt volcano eruptions. Nat. Geosci. 6 , 652–656 (2013).
Le Breton, M. et al. Passive radio-frequency identification ranging, a dense and weather-robust technique for landslide displacement monitoring. Eng. Geol. 250 , 1–10 (2019).
Madson, A., Fielding, E., Sheng, Y. & Cavanaugh, K. High-resolution spaceborne, airborne and in situ landslide kinematic measurements of the Slumgullion landslide in Southwest Colorado. Remote. Sens. 11 , 265 (2019).
Thomas, A. M., Spica, Z., Bodmer, M., Schulz, W. H. & Roering, J. J. Using a dense seismic array to determine structure and site effects of the two towers earthflow in northern California. Seismol. Res. Lett. 91 , 913–920 (2020).
Truffert, C. et al. Large 3D resistivity and IP measurement of the Séchilienne landslide using the FullWaver system. Proc. EAGE-HAGI 1st Asia Pacfic Meeting on Near Surface Geoscience and Engineering 2018 , 1–4 (2018).
Loke, M., Chambers, J., Rucker, D., Kuras, O. & Wilkinson, P. Recent developments in the direct-current geoelectrical imaging method. J. Appl. Geophys. 95 , 135–156 (2013).
Perrone, A., Lapenna, V. & Piscitelli, S. Electrical resistivity tomography technique for landslide investigation: A review. Earth Sci. Rev. 135 , 65–82 (2014).
Uhlemann, S. et al. Four-dimensional imaging of moisture dynamics during landslide reactivation. J. Geophys. Res. Earth Surf. 122 , 398–418 (2017).
Uhlemann, S. et al. Assessment of ground-based monitoring techniques applied to landslide investigations. Geomorphology 253 , 438–451 (2016).
Whiteley, J. S., Chambers, J. E., Uhlemann, S., Wilkinson, P. B. & Kendall, J. M. Geophysical monitoring of moisture-induced landslides: a review. Rev. Geophys. 57 , 106–145 (2019).
Gariano, S. L. & Guzzetti, F. Landslides in a changing climate. Earth Sci. Rev. 162 , 227–252 (2016).
Carlà, T., Farina, P., Intrieri, E., Ketizmen, H. & Casagli, N. Integration of ground-based radar and satellite InSAR data for the analysis of an unexpected slope failure in an open-pit mine. Eng. Geol. 235 , 39–52 (2018).
Dong, J. et al. Measuring precursory movements of the recent Xinmo landslide in Mao County, China with Sentinel-1 and ALOS-2 PALSAR-2 datasets. Landslides 15 , 135–144 (2018).
Roberts, N. J. et al. Changes in ground deformation prior to and following a large urban landslide in La Paz, Bolivia, revealed by advanced InSAR. Nat. Hazards Earth Syst. Sci. 19 , 679–696 (2019).
Walter, T. R. et al. Complex hazard cascade culminating in the Anak Krakatau sector collapse. Nat. Commun. 10 , 4339 (2019).
Crosta, G. B. & Agliardi, F. How to obtain alert velocity thresholds for large rockslides. Phys. Chem. Earth Parts A/B/C 27 , 1557–1565 (2002).
Bennett, G. L., Miller, S. R., Roering, J. J. & Schmidt, D. A. Landslides, threshold slopes, and the survival of relict terrain in the wake of the Mendocino Triple junction. Geology 44 , 363–366 (2016).
Handwerger, A. L., Roering, J. J., Schmidt, D. A. & Rempel, A. W. Kinematics of earthflows in the Northern California Coast Ranges using satellite interferometry. Geomorphology 246 , 321–333 (2015).
Dini, B., Manconi, A. & Loew, S. Investigation of slope instabilities in NW Bhutan as derived from systematic DInSAR analyses. Eng. Geol. 259 , 105111 (2019).
Strozzi, T. et al. Satellite SAR interferometry for the improved assessment of the state of activity of landslides: A case study from the Cordilleras of Peru. Remote. Sens. Environ. 217 , 111–125 (2018).
Pham, M. Q., Lacroix, P. & Doin, M. P. Sparsity optimization method for slow-moving landslides detection in satellite image time-series. IEEE Trans. Geosci. Remote Sens. 57 , 2133–2144 (2018).
Roering, J. J., Stimely, L. L., Mackey, B. H. & Schmidt, D. A. Using DInSAR, airborne LiDAR, and archival air photos to quantify landsliding and sediment transport. Geophys. Res. Lett. 36 , L19402 (2009).
Kelsey, H. M. Earthflows in Franciscan melange, Van Duzen River basin, California. Geology 6 , 361–364 (1978).
Finnegan, N. J. et al. River channel width controls blocking by slow-moving landslides in California’s Franciscan mélange. Earth Surf. Dyn. 7 , 879–894 (2019).
Delbridge, B. G., Bürgmann, R., Fielding, E., Hensley, S. & Schulz, W. H. Three-dimensional surface deformation derived from airborne interferometric UAVSAR: application to the Slumgullion Landslide. J. Geophys. Res. Solid Earth 121 , 3951–3977 (2016).
Stumpf, A., Malet, J. P., Allemand, P. & Ulrich, P. Surface reconstruction and landslide displacement measurements with Pléiades satellite images. ISPRS J. Photogramm. Remote Sens. 95 , 1–12 (2014).
Schlögel, R. et al. Multi-temporal X-band radar interferometry using corner reflectors: Application and validation at the Corvara Landslide (Dolomites, Italy). Remote Sens. 9 , 739 (2017).
Chambers, J. et al. Three-dimensional geophysical anatomy of an active landslide in Lias Group mudrocks, Cleveland Basin, UK. Geomorphology 125 , 472–484 (2011).
Petley, D., Mantovani, F., Bulmer, M. & Zannoni, A. The use of surface monitoring data for the interpretation of landslide movement patterns. Geomorphology 66 , 133–147 (2005).
Massey, C. I., Petley, D. N. & McSaveney, M. Patterns of movement in reactivated landslides. Eng. Geol. 159 , 1–19 (2013).
Baum, R. L., Messerich, J. & Fleming, R. W. Surface deformation as a guide to kinematics and three-dimensional shape of slow-moving, clay-rich landslides, Honolulu, Hawaii. Environ. Eng. Geosci. 4 , 283–306 (1998).
O’Brien, G. A., Cox, S. C. & Townend, J. Spatially and temporally systematic hydrologic changes within large geoengineered landslides, Cromwell Gorge, New Zealand, induced by multiple regional earthquakes. J. Geophys. Res. Solid Earth 121 , 8750–8773 (2016).
Casson, B., Delacourt, C., Baratoux, D. & Allemand, P. Seventeen years of the “La Clapiere” landslide evolution analysed from ortho-rectified aerial photographs. Eng. Geol. 68 , 123–139 (2003).
Warrick, J. A., Ritchie, A. C., Schmidt, K. M., Reid, M. E. & Logan, J. Characterizing the catastrophic 2017 Mud Creek landslide, California, using repeat structure-from-motion (SfM) photogrammetry. Landslides 16 , 1201–1219 (2019).
Sassa, K. The movement and the mechanism of a crystalline schist landslide “Zentoku” in Japan. Proc. Interpraevent 1980 1 , 85–106 (1980).
Oppikofer, T., Jaboyedoff, M., Blikra, L., Derron, M.-H. & Metzger, R. Characterization and monitoring of the Åknes rockslide using terrestrial laser scanning. Nat. Hazards Earth Syst. Sci. 9 , 1003–1019 (2009).
Grøneng, G., Christiansen, H. H., Nilsen, B. & Blikra, L. H. Meteorological effects on seasonal displacements of the Åknes rockslide, western Norway. Landslides 8 , 1–15 (2011).
Ganerød, G. V. et al. Geological model of the Åknes rockslide, western Norway. Eng. Geol. 102 , 1–18 (2008).
Kos, A. et al. Contemporary glacier retreat triggers a rapid landslide response, Great Aletsch Glacier, Switzerland. Geophys. Res. Lett. 43 , 12,466–12,474 (2016).
Cappa, F., Guglielmi, Y., Viseur, S. & Garambois, S. Deep fluids can facilitate rupture of slow-moving giant landslides as a result of stress transfer and frictional weakening. Geophys. Res. Lett. 41 , 61–66 (2014).
Lacroix, P. & Helmstetter, A. Location of seismic signals associated with microearthquakes and rockfalls on the Séchilienne landslide, French Alps. Bull. Seismol. Soc. Am. 101 , 341–353 (2011).
Marone, C. Laboratory-derived friction laws and their application to seismic faulting. Annu. Rev. Earth Planet Sci. 26 , 643–696 (1998).
Download references
Acknowledgements
Part of this research was carried out at the Jet Propulsion Laboratory, California Institute of Technology, under a contract with the National Aeronautics and Space Administration (NASA). P.L. and G.B. are part of LabEx OSUG@2020 (ANR10 LABX56).
Author information
Authors and affiliations.
Université Grenoble Alpes, Université Savoie Mont Blanc, CNRS, IRD, IFSTTAR, ISTerre, Grenoble, France
Pascal Lacroix & Grégory Bièvre
Joint Institute for Regional Earth System Science and Engineering, University of California, Los Angeles, Los Angeles, CA, USA
Alexander L. Handwerger
Jet Propulsion Laboratory, California Institute of Technology, Pasadena, CA, USA
You can also search for this author in PubMed Google Scholar
Contributions
All authors contributed to all aspects of the article.
Corresponding author
Correspondence to Pascal Lacroix .
Ethics declarations
Competing interests.
The authors declare no competing interests.
Additional information
Peer review information.
Nature Reviews Earth & Environment thanks E. Intrieri, X. Hu and F. Zhang for their contribution to the peer review of this work.
Publisher’s note
Springer Nature remains neutral with regard to jurisdictional claims in published maps and institutional affiliations.
Related links
USGS-USA: https://www.usgs.gov/natural-hazards/landslide-hazards/monitoring
Rights and permissions
Reprints and permissions
About this article
Cite this article.
Lacroix, P., Handwerger, A.L. & Bièvre, G. Life and death of slow-moving landslides. Nat Rev Earth Environ 1 , 404–419 (2020). https://doi.org/10.1038/s43017-020-0072-8
Download citation
Accepted : 11 June 2020
Published : 21 July 2020
Issue Date : August 2020
DOI : https://doi.org/10.1038/s43017-020-0072-8
Share this article
Anyone you share the following link with will be able to read this content:
Sorry, a shareable link is not currently available for this article.
Provided by the Springer Nature SharedIt content-sharing initiative
This article is cited by
An integrated approach for mapping slow-moving hillslopes and characterizing their activity using insar, slope units and a novel 2-d deformation scheme.
- Nitheshnirmal Sadhasivam
- Hakan Tanyaş
Natural Hazards (2024)
InSAR-derived predisaster spatio-temporal evolution of a reactivated landslide
- Ruichen Zhou
Bulletin of Engineering Geology and the Environment (2024)
The 2022 Chaos Canyon landslide in Colorado: Insights revealed by seismic analysis, field investigations, and remote sensing
- Kate E. Allstadt
- Jeffrey A. Coe
- Lance R. Brady
Landslides (2024)
Model test study on natural frequency evolution and early warning of reservoir landslides under water level fluctuations
- Zhi-xiang Wu
- Xue-liang Zhang
Critical assessment of landslide failure forecasting methods with case histories: a comparative study of INV, MINV, SLO, and VOA
- Sohrab Sharifi
- Renato Macciotta
- Michael T. Hendry
Quick links
- Explore articles by subject
- Guide to authors
- Editorial policies
Sign up for the Nature Briefing newsletter — what matters in science, free to your inbox daily.


A .gov website belongs to an official government organization in the United States.
A lock ( ) or https:// means you've safely connected to the .gov website. Share sensitive information only on official, secure websites.
- Natural Disasters and Severe Weather
- Health Topics A-Z
Landslides and Mudslides and Your Safety
- Some areas are more prone to landslides and mudslides than others.
Know what to do to protect yourself if you live in an area prone to landslides or mudslides.
What are landslides and debris flows?
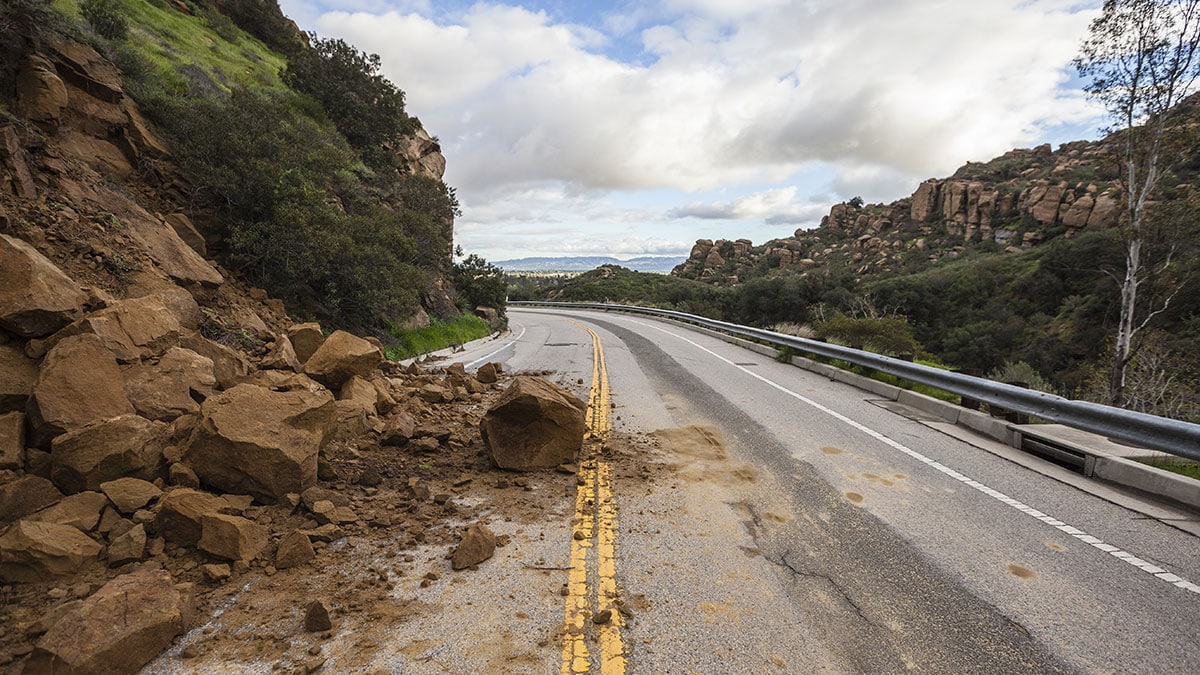
Landslides occur when masses of rock, earth, or debris move down a slope.
Mudslides or debris flows
Debris flows , also known as mudslides , are a common type of fast-moving landslide that tends to flow in channels.
What causes them
Landslides are caused by disturbances in the natural stability of a slope. They can accompany heavy rains or follow droughts, earthquakes, or volcanic eruptions.
Mudslides develop when water rapidly accumulates in the ground and results in a surge of water-saturated rock, earth, and debris. Mudslides usually start on steep slopes and can be activated by natural disasters. Areas where wildfires or human modification of the land have destroyed vegetation on slopes are particularly vulnerable to landslides during and after heavy rains.
Health impacts
In the United States, landslides and debris flows result in 25 to 50 deaths each year. The health hazards associated with landslides and mudflows include:
- Rapidly moving water and debris that can lead to trauma;
- Broken electrical, water, gas, and sewage lines that can result in injury or illness; and
- Disrupted roadways and railways that can endanger motorists and disrupt transport and access to health care.
Some areas are more likely to experience landslides or mudslides, including:
- Areas where wildfires or human modification of the land have destroyed vegetation;
- Areas where landslides have occurred before;
- Steep slopes and areas at the bottom of slopes or canyons;
- Slopes that have been altered for construction of buildings and roads;
- Channels along a stream or river; and
- Areas where surface runoff is directed.
Protect yourself before a landslide or mudslide
- Assume that steep slopes and areas burned by wildfires are vulnerable to landslides and debris flows.
- Contact local authorities about emergency and evacuation plans.
- Develop emergency and evacuation plans for your family and business.
- Develop an emergency communication plan in case family members are separated.
- If you live in an area vulnerable to landslides, consider leaving it.
Learn about your area's risk for landslides
During intense storms and rainfall, stay alert during intense storms or rainfall..
- Listen to the radio or watch TV for warnings about intense rainfall or for information and instructions from local officials.
- Be aware of any sudden increase or decrease in water level on a stream or creek that might indicate debris flow upstream. A trickle of flowing mud may precede a larger flow.
- Look for tilted trees, telephone poles, fences, or walls, and for new holes or bare spots on hillsides.
- Listen for rumbling sounds that might indicate an approaching landslide or mudflow.
- Be alert when driving. Roads may become blocked or closed due to collapsed pavement or debris.
Quickly move away from the path of a landslide or debris flow.
- Getting out of the path of a debris flow is your best protection. Move to the nearest high ground in a direction away from the path.
- If rocks and debris are approaching, run for the nearest shelter and take cover (if possible, under a desk, table, or other piece of sturdy furniture).
Stay safe after a landslide or mudslide
- Stay away from the site. Flooding or additional slides may occur after a landslide or mudflow.
- Check for injured or trapped people near the affected area, if it is possible to do so without entering the path of the landslide or mudflow.
- Listen to the radio or TV for emergency information.
- Report broken utility lines to the appropriate authorities.
- Consult a geotechnical expert (a registered professional engineer with soils engineering expertise) for advice on reducing additional landslide problems and risks. Local authorities should be able to tell you how to contact a geotechnical expert.
- The U.S. Geological Survey (USGS) has a fact sheet containing information on landslides and mudflows in the United States, as well as recommendations on how to prepare and behave during and after a landslide.
- The U.S. Geological Survey (USGS) provides information on landslide hazards and characteristics, and up-to-date information on recent and past landslide events.
- The American Red Cross (ARC) has a Web site containing information on landslides and mudslides.
- University of Colorado – Natural Hazards Center provides a resources page of landslide-related websites.
- For information about reducing losses from landslides at the national, state, and local levels, in both public and private sectors, review the following document: Spiker EC, Gori PL. National landslide hazards mitigation strategy — a framework for loss reduction . U.S. Geological Survey Circular 1244 – Online Version 1.0, 2003.
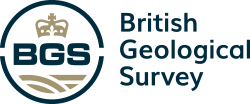
Quick links
- Climate change
- Multiple hazards
- Decarbonisation
Understanding landslides
Discovering Geology — Earth hazards
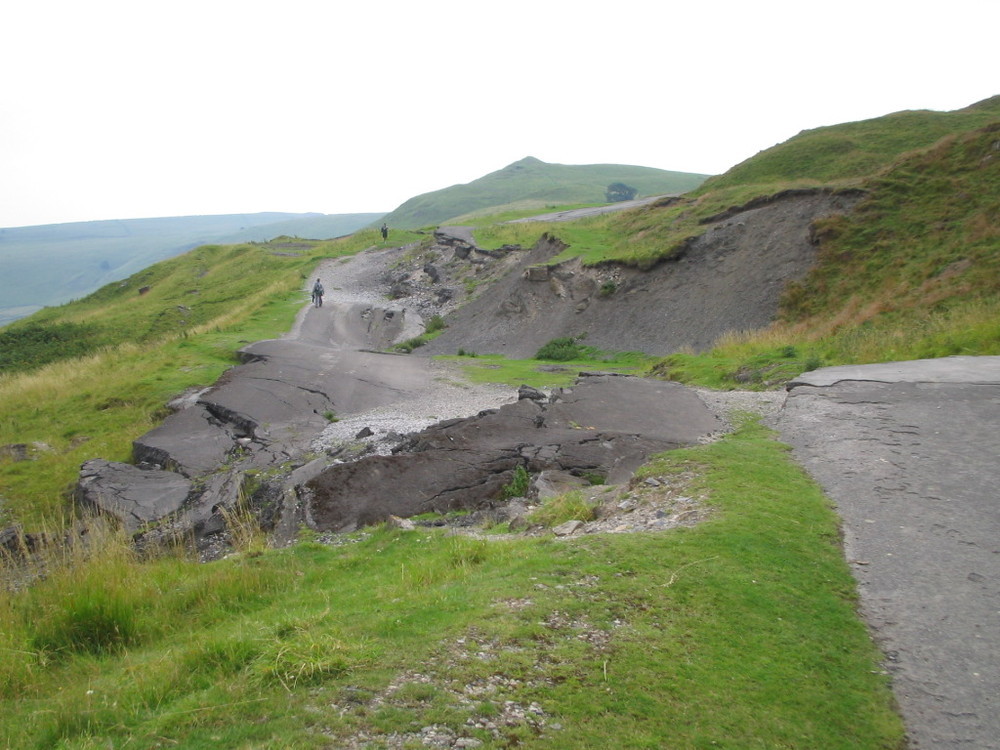
What is a landslide?
A landslide is a mass movement of material, such as rock, earth or debris, down a slope. They can happen suddenly or more slowly over long periods of time.
When the force of gravity acting on a slope exceeds the resisting forces of a slope, the slope will fail and a landslide occurs. External factors can lead to landslides happening, including:
- heavy rainfall leading to saturation of the ground
- erosion of the base of a slope
- changes to the material’s strength through weathering
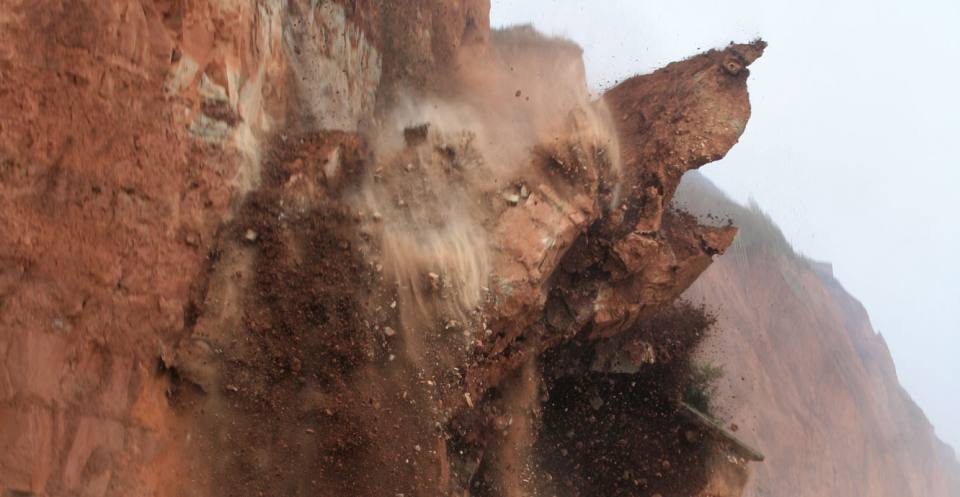
Rock fall landslide at Pennington Point, near Sidmouth on the south-east Devon coast, UK. © Eve Mathews, used with kind permission. All rights reserved.
Landslides are classified by their type of movement. The four main types of movement are:
- slides (rotational and translational)
Landslides can be classified as just one of these movements or, more commonly, can be a mixture of several.
At BGS we usually study the landslides that happen in the UK , but here we also include some examples of large landslides that have occurred elsewhere around the world and also in the sea .
Why do landslides happen?
A landslide may occur because the strength of the material is weakened. This reduces the power of the ‘glue’ that cements the rock or soil grains together. Located on a slope, the rock is then no longer strong enough to resist the forces of gravity acting upon it.
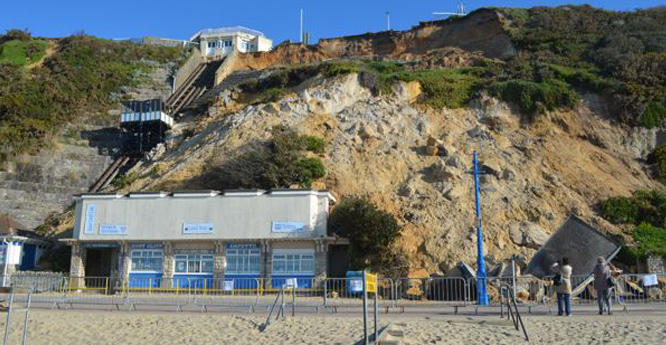
On 24 April 2016, a landslide at East Cliff, Bournemouth caused damage to a funicular railway, a toilet block and an array of fences and benches that were at the top of the cliff. BGS © UKRI.
What can increase the chance of a landslide?
Several factors can increase a slope’s susceptibility to a landslide event.
- Water: adding water to the material on a slope makes a landslide more likely to happen. This is because water adds weight, lowers the strength of the material and reduces friction, making it easier for material to move downslope.
- Erosion processes: if the bottom of a slope is continually eroded, for example by the sea or a river, the slope will eventually become too steep to hold itself up.
- Slope angle (steepness of slope): the slope angle is a key factor as far as landslides are concerned. Any change to this that makes it steeper (such as coastal erosion) increases the likelihood of a landslide.
- Rock type: the type of rocks in the slope, and their combination, can increase the chance of a landslide.
- Grain shape: the shape of the grains that make up a rock can affect the risk of a landslide.
- Jointing and orientation of bedding planes.
- Arrangement of the rock layers.
- Weathering processes: for example freeze-thaw reduces the cohesion (‘stickiness’) between the rock grains.
- Vegetation: vegetation helps bind material together; removing vegetation increases the chance of a landslide.
- Volcanoes and earthquake activity nearby.
- Human activity: mining, traffic vibrations or urbanisation change surface water drainage patterns.
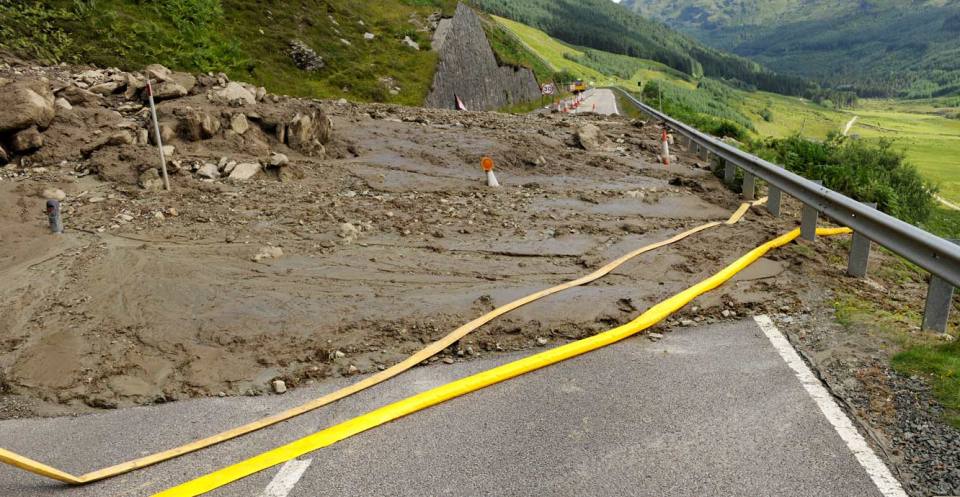
Following a period of heavy rain, a debris flow landslide occurred along the A83 Rest and Be Thankful pass (Argyll and Bute, Scotland) on 1 August 2012. It was reported that between 50 and 100 tonnes of material blocked the road, which was subsequently closed in both directions resulting in a long diversion. BGS © UKRI.
Further information on landslides
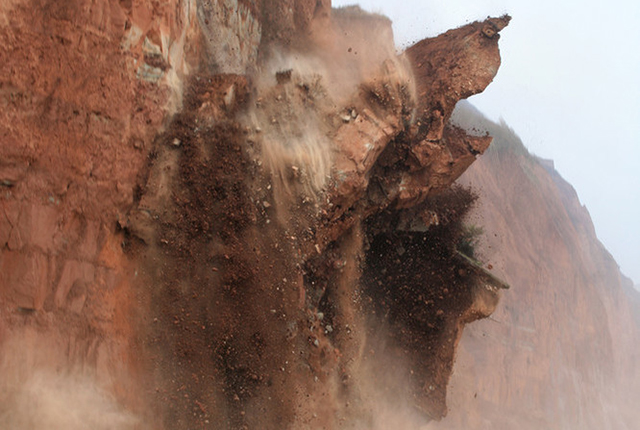
Landslide case studies
The landslides team at the BGS has studied numerous landslides. This work informs our geological maps, memoirs and sheet explanations and provides data for our National Landslide Database, which underpins much of our research.
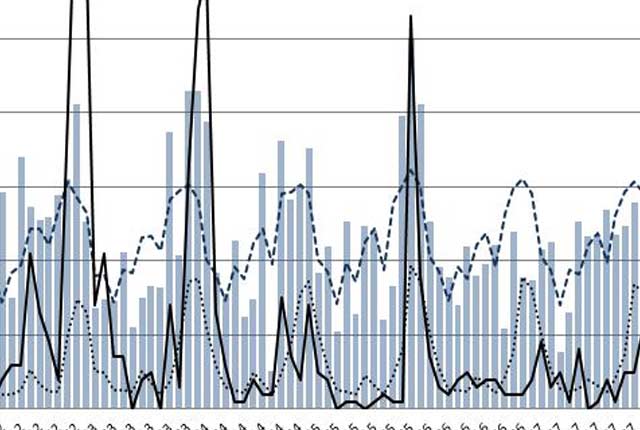
Landslides and rainfall
We combine rainfall statistics and landslide data to produce graphs demonstrating the relationship between landslides and rainfall.
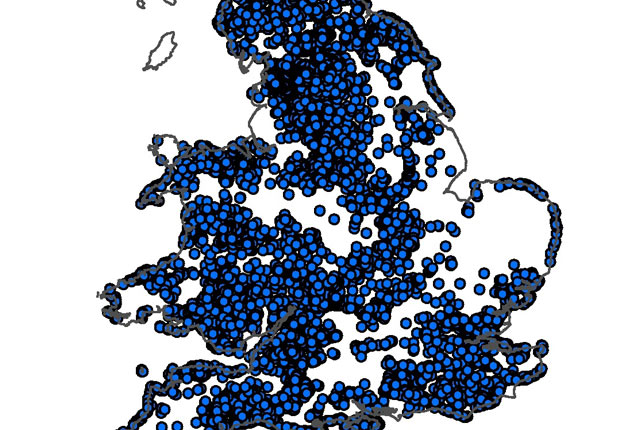
National Landslide Database
The BGS National Landslide Database (NLD) is the most extensive source of information on landslides in Great Britain.
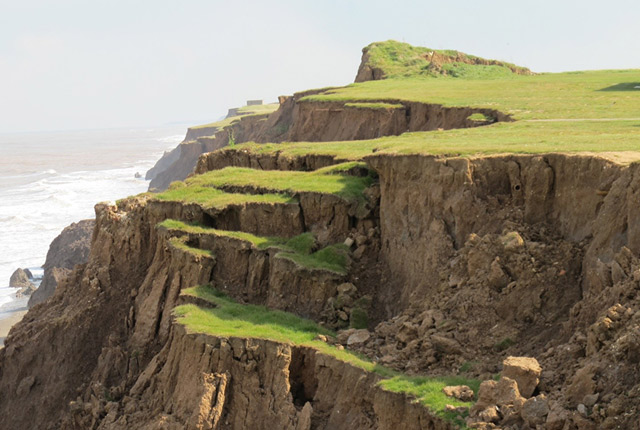
Landslide observatories
BGS have long-term monitoring observatories where data have been collected, in some instances, since 2001.
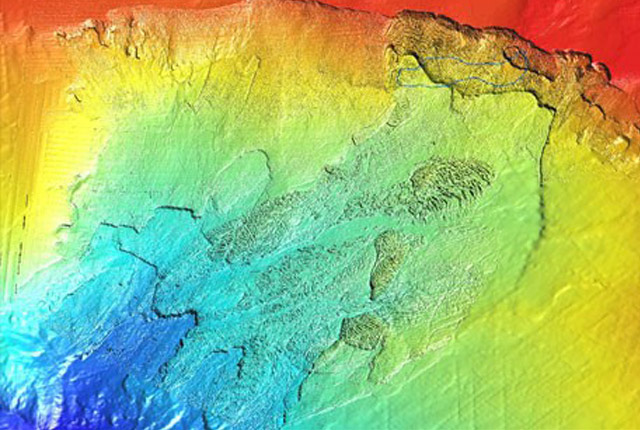
Landslides in the UK and around the world
Landslides in the UK, around the world and under the sea.
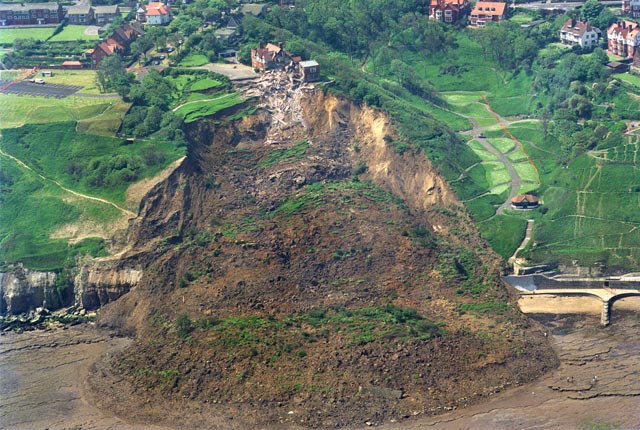
How to classify a landslide
Landslides are classified by their type of movement. The four main types of movement are falls, topples, slides and flows.
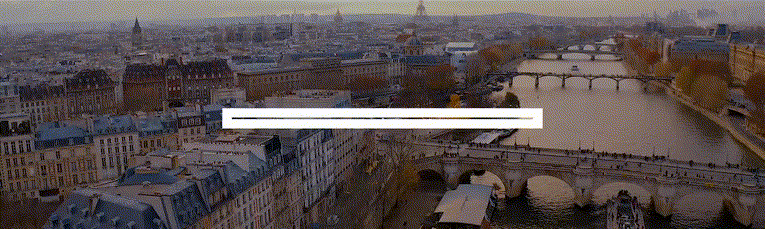
You may also be interested in
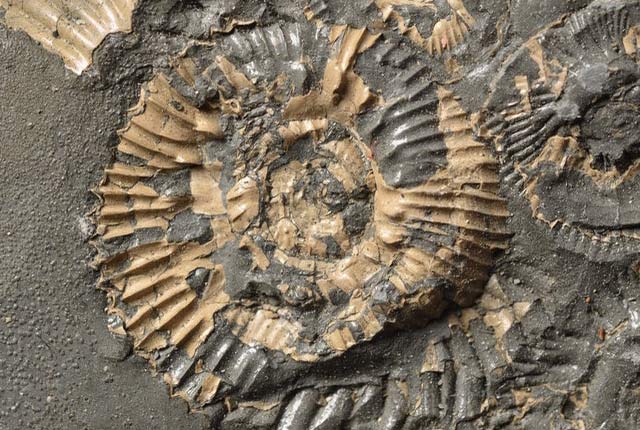
Discovering Geology
Discovering Geology introduces a range of geoscience topics to school-age students and learners of all ages.
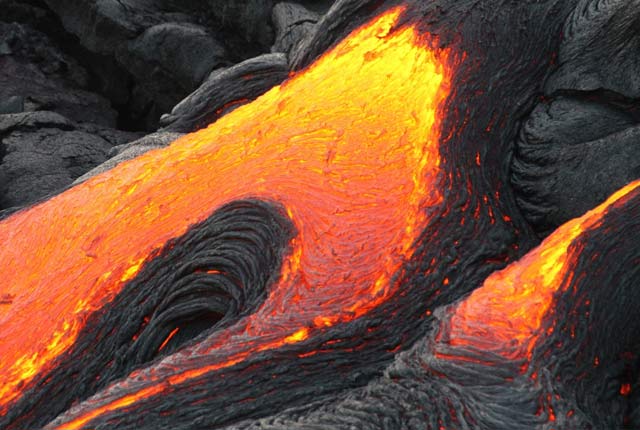
Earth hazards
The Earth beneath our feet is constantly shifting and moving, and violently with catastrophic and immediate results. Find out more about earth hazards.
Was this page helpful?
How can we make this section better?*
- How can we make this section better? *
Please select a reason*
- Please select a reason * Select reason Didn't answer my question Too confusing Links are broken/Doesn't work
Landslide and Debris Flow (Mudslide)
What are landslides and debris flows, and what causes them.
- Some landslides move slowly and cause damage gradually, whereas others move so rapidly that they can destroy property and take lives suddenly and unexpectedly. Gravity is the force driving landslide movement. Factors that allow the force of gravity to overcome the resistance of earth material to landslide movement include: saturation by water, steepening of slopes by erosion or construction, alternate freezing or thawing, earthquake shaking, and volcanic eruptions.
- Landslides are typically associated with periods of heavy rainfall or rapid snow melt and tend to worsen the effects of flooding that often accompanies these events. In areas burned by forest and brush fires, a lower threshold of precipitation may initiate landslides.
- Debris flows, sometimes referred to as mudslides, mudflows, lahars, or debris avalanches, are common types of fast-moving landslides. These flows generally occur during periods of intense rainfall or rapid snow melt. They usually start on steep hillsides as shallow landslides that liquefy and accelerate to speeds that are typically about 10 miles per hour, but can exceed 35 miles per hour. The consistency of debris flows ranges from watery mud to thick, rocky mud that can carry large items such as boulders, trees, and cars. Debris flows from many different sources can combine in channels, and their destructive power may be greatly increased. They continue flowing down hills and through channels, growing in volume with the addition of water, sand, mud, boulders, trees, and other materials. When the flows reach flatter ground, the debris spreads over a broad area, sometimes accumulating in thick deposits that can wreak havoc in developed areas.
- Among the most destructive types of debris flows are those that accompany volcanic eruptions. A spectacular example in the United States was a massive debris flow resulting from the 1980 eruptions of Mount St. Helens, Washington. Areas near the bases of many volcanoes in the Cascade Mountain Range of California, Oregon, and Washington are at risk from the same types of flows during future volcanic eruptions.
- Wildfires can also lead to destructive debris-flow activity. In July 1994, a severe wildfire swept Storm King Mountain, west of Glenwood Springs, Colorado, denuding the slopes of vegetation. Heavy rains on the mountain in September resulted in numerous debris flows, one of which blocked Interstate 70 and threatened to dam the Colorado River.
- Learn whether landslides or debris flows have occurred in your area by contacting local officials, state geological surveys or departments of natural resources, and university departments of geology.
Awareness Information
- Areas that are generally prone to landslide hazards include existing old landslides; the bases of steep slopes; the bases of drainage channels; and developed hillsides where leach-field septic systems are used.
- Areas that are typically considered safe from landslides include areas that have not moved in the past; relatively flat-lying areas away from sudden changes in slope; and areas at the top or along ridges, set back from the tops of slopes.
- Learn what to watch for prior to major landsliding. Look for patterns of storm-water drainage on slopes near your home, noting especially the places where runoff water converges, increasing flow over soil-covered slopes. Check hillsides around your home for any signs of land movement, such as small landslides or debris flows or progressively tilting trees.
Plan for a Landslide
If you are at risk from landslides:.
- Talk to your insurance agent. Debris flow may be covered by flood insurance policies from the National Flood Insurance Program (NFIP) .
- Develop an evacuation plan. (See "Evacuation" in the "Family Disaster Plan" section.) You should know where to go if you have to leave. Trying to make plans at the last minute can be upsetting and create confusion.
- Discuss landslides and debris flow with your family. Everyone should know what to do in case all family members are not together. Discussing disaster ahead of time helps reduce fear and lets everyone know how to respond during a landslide or debris flow.
- Disaster Suplies Kit basics.
- Evacuation Supplies Kit.
How to Protect Your Property
- If your property is in a landslide-prone area, contract with a private consulting company specializing in earth movement for opinions and advice on landslide problems and on corrective measures you can take. Such companies would likely be those specializing in geotechnical engineering, structural engineering, or civil engineering. Local officials could possibly advise you as to the best kind of professional to contact in your area. Taking steps without consulting a professional could make your situation worse.
- Install flexible pipe fittings to avoid gas or water leaks. Flexible fittings will be less likely to break.
Media and Community Education Ideas
- In an area prone to landslides, publish a special newspaper section with emergency information on landslides and debris flows. Localize the information by including the phone numbers of local emergency services offices, the American Red Cross chapter, and hospitals.
- Report on what city and county governments are doing to reduce the possibility of landslides. Interview local officials about local land- use zoning regulations.
- Interview local officials and major insurers regarding the National Flood Insurance Program . Find out if debris flow is covered by flood insurance policies from the National Flood Insurance Program and contact your local emergency management office to learn more about the program.
- Work with local emergency services and American Red Cross officials to prepare special reports for people with mobility impairments on what to do if evacuation is ordered.
- Support your local government in efforts to develop and enforce land-use and building ordinances that regulate construction in areas susceptible to landslides and debris flows. Buildings should be located away from steep slopes, streams and rivers, intermittent-stream channels, and the mouths of mountain channels.
What to Do Before Intense Storms
- Become familiar with the land around you. Learn whether landslides and debris flows have occurred in your area by contacting local officials, state geological surveys or departments of natural resources, and university departments of geology. Knowing the land can help you assess your risk for danger.
- Watch the patterns of storm-water drainage on slopes near your home, and especially the places where runoff water converges, increasing flow over soil-covered slopes. Watch the hillsides around your home for any signs of land movement, such as small landslides or debris flows, or progressively tilting trees. Watching small changes could alert you to the potential of a greater landslide threat.
What to Do During Intense Storms
- Stay alert and awake. Many debris-flow fatalities occur when people are sleeping. Listen to a NOAA Weather Radio or portable, battery-powered radio or television for warnings of intense rainfall. Be aware that intense, short bursts of rain may be particularly dangerous, especially after longer periods of heavy rainfall and damp weather.
- If you are in areas susceptible to landslides and debris flows, consider leaving if it is safe to do so. Remember that driving during an intense storm can be hazardous. If you remain at home, move to a second story if possible. Staying out of the path of a landslide or debris flow saves lives.
- Listen for any unusual sounds that might indicate moving debris, such as trees cracking or boulders knocking together. A trickle of flowing or falling mud or debris may precede larger landslides. Moving debris can flow quickly and sometimes without warning.
- If you are near a stream or channel, be alert for any sudden increase or decrease in water flow and for a change from clear to muddy water. Such changes may indicate landslide activity upstream, so be prepared to move quickly. Don't delay! Save yourself, not your belongings.
- Be especially alert when driving. Embankments along roadsides are particularly susceptible to landslides. Watch the road for collapsed pavement, mud, fallen rocks, and other indications of possible debris flows.
What to Do if You Suspect Imminent Landslide Danger
- Contact your local fire, police, or public works department. Local officials are the best persons able to assess potential danger.
- Inform affected neighbors. Your neighbors may not be aware of potential hazards. Advising them of a potential threat may help save lives. Help neighbors who may need assistance to evacuate.
- Evacuate. Getting out of the path of a landslide or debris flow is your best protection.
What to Do During a Lanslide
- Quickly move out of the path of the landslide or debris flow. Moving away from the path of the flow to a stable area will reduce your risk.
- If escape is not possible, curl into a tight ball and protect your head. A tight ball will provide the best protection for your body.
What to Do After a Landslide
- Stay away from the slide area. There may be danger of additional slides.
- Check for injured and trapped persons near the slide, without entering the direct slide area. Direct rescuers to their locations.
- Help a neighbor who may require special assistance - infants, elderly people, and people with disabilities. Elderly people and people with disabilities may require additional assistance. People who care for them or who have large families may need additional assistance in emergency situations.
- Listen to local radio or television stations for the latest emergency information.
- Watch for flooding, which may occur after a landslide or debris flow. Floods sometimes follow landslides and debris flows because they may both be started by the same event.
- Look for and report broken utility lines to appropriate authorities. Reporting potential hazards will get the utilities turned off as quickly as possible, preventing further hazard and injury.
- Check the building foundation, chimney, and surrounding land for damage. Damage to foundations, chimneys, or surrounding land may help you assess the safety of the area.
- Replant damaged ground as soon as possible since erosion caused by loss of ground cover can lead to flash flooding.
- Seek the advice of a geotechnical expert for evaluating landslide hazards or designing corrective techniques to reduce landslide risk. A professional will be able to advise you of the best ways to prevent or reduce landslide risk, without creating further hazard.
Produced by the National Disaster Education Coalition: American Red Cross, FEMA , IAEM , IBHS , NFPA , NWS , USDA/CSREES , and USGS . HTML formating By the Disaster Center
From: Talking About Disaster: Guide for Standard Messages. Produced by the National Disaster Education Coalition, Washington, D.C., 1999.
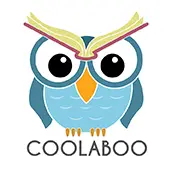
Landslide Facts for Kids
Landslides involve soil, rock, and debris sliding down slopes, triggered by rain, earthquakes, or human actions. They vary in speed and size, posing risks to property and lives. Teaching kids about landslides highlights slope stability, soil management, and safety measures.
- Landslides are rocks & soil moving downhill.
- Heavy rain often triggers landslides.
- Earthquakes can also cause landslides.
- Loose soil makes landslides more likely.
- Steep slopes increase landslide risk.
- Landslides can be slow or fast.
- They can cause damage to property.
- Plants help stabilize soil on slopes.
- Humans can cause landslides too.
- Early warning systems save lives.
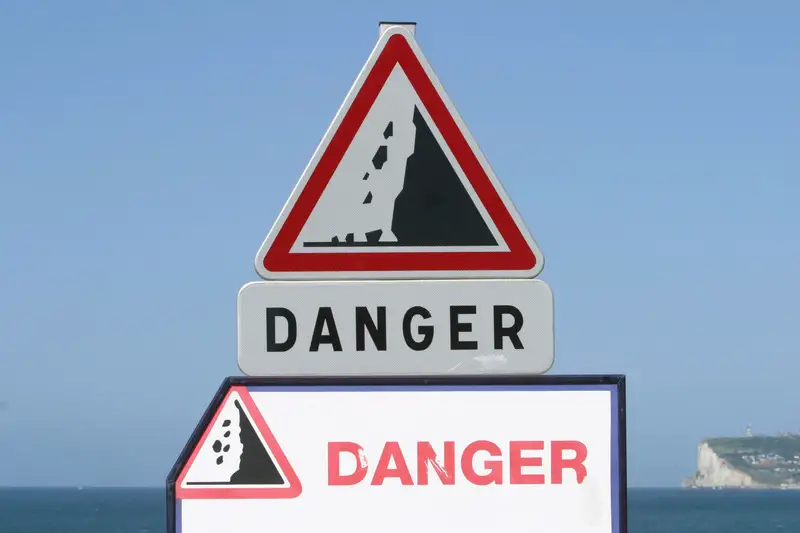
Are Landslides Dangerous?
Landslides can cause a lot of damage when they happen.
This damage can cause paths to be swept away, it can cause trees, homes, and even things in the way to be covered or damaged.
When a landslide happens, rivers and lakes can be filled in or covered and in a terrible situation, even towns can be destroyed by landslides.
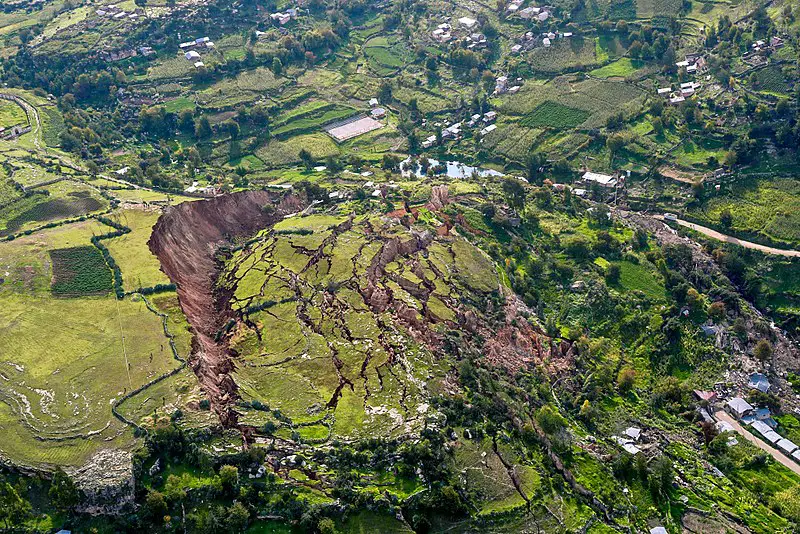
How Does a Landslide Start?
In order for a landslide to happen, there must first be a slope, and more than likely, it will be a steep slope.
When there are waves and heavy rains or some type of erosion, the surface of the slope can start to get worn away. When something gets worn away, this is called erosion.
When erosion happens, it can cause the slope to become even steeper and the slope becomes weak and is not able to support all of the soil and rocks that are on it.
This can cause the material on the slope to be loose and when the gravity comes, it can cause all of the rocks and soil to come tumbling down the slope.
What Besides Erosion Can Cause Landslides?
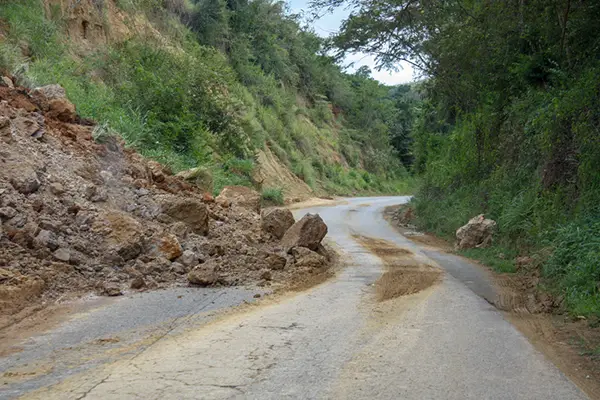
Even though erosion is a big reason that there are landslides, other things such as when big snows melt and when there are heavy rainstorms, the material on the slope can still be loosened and can cause landslides to happen.
Natural disasters such as earthquakes or volcanoes can also cause landslides.
When areas experience explosions or shaking, this can cause the material of the slope to be loosened and can cause a landslide to happen.
What is a Submarine Landslide?
When there is an earthquake, the land under the water can begin to quake and slide.
When this happens, this is called a submarine landslide.
Where Do Landslides Happen?
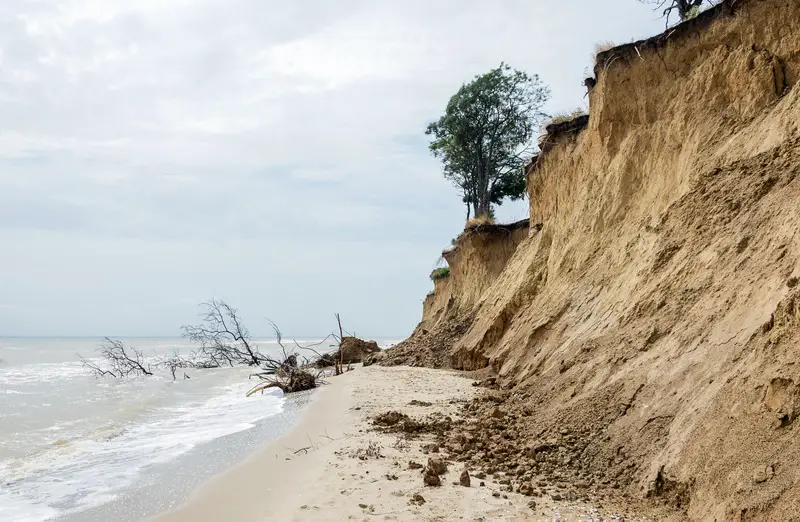
Landslides can happen in:
- All regions
- All 50 states
- Coastal Areas
- Mountainous Areas
- Hilly Areas
How Fast Do Landslides Travel?
Landslides can travel at a slow rate or a fast rate. Some landslides can travel at:
- 200 miles per hour.
- 30-50 miles per hour.
There are different things that depend on how fast the landslide will travel such as:
- How steep the slope is.
- What type of Earth is on the slope.
- The angle of the slope.
- How much water hits the slope.
- What type of debris is on the slope.
Why is it Important to Understand Landslides?
It is important for people to study landslides because they cause a lot of damage and they sometimes even cause people to die.
- The United States had over $1 billion dollars of damage from landslides.
- There are around 25-50 people that die from landslides each year.
What Areas Are More at Risk from Landslide?
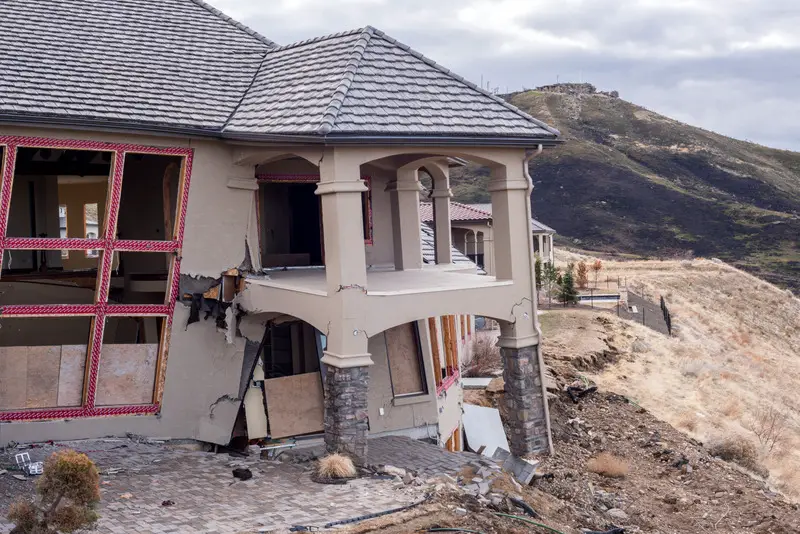
People that live in areas that are hilly or mountainous have a chance to experience a landslide.
When there is new construction, it is important for them to understand about landslides so that they can build things that will help to reduce the landslides from happening.
What Are Some Major Landslides That Happened?
- On May 18, 1980, Mount St. Helen had a major landslide.
- On March 22, 2014, Oso, Washington had a major landslide.
- On April 9, 2000, Tibet had one of the largest recorded landslides.
- On August 8, 2010, Zhougu, China had a major landslide.
Do People Cause Landslides?
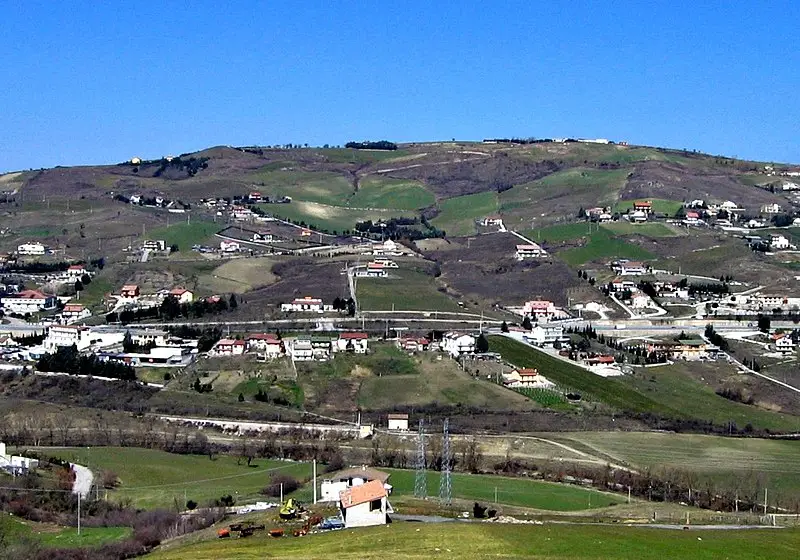
Sometimes the things that people do can cause landslides.
When people build roads, buildings, and other things, they sometimes forget about the drainage and how their buildings can increase landslides or cause older ones to happen again.
What Can Scientists Use to Keep an Eye Out for Landslides?
- ASTER-a satellite map that shows slopes and hills.
- Global Precipitation Measurement or GPM-this is a satellite to map how much rain has fallen.
What Other Things Are Like Landslides?
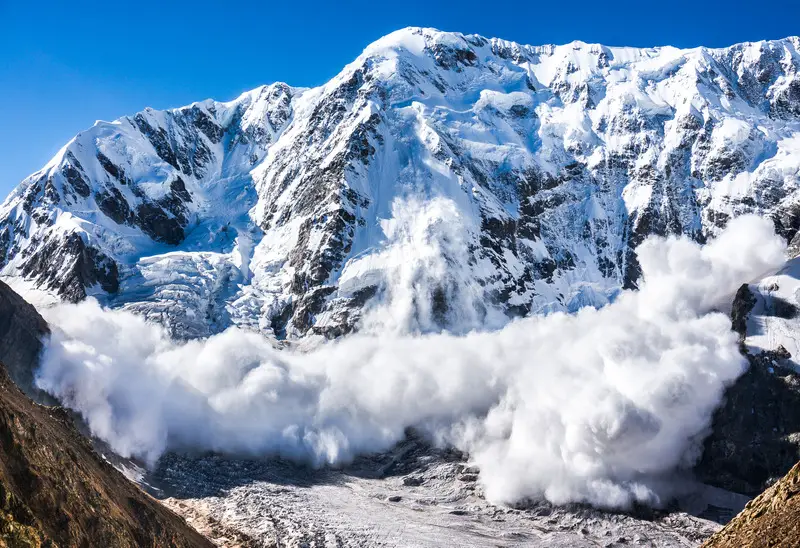
Other things that are similar to landslides are:
These things do the same thing as a landslide except the material is different.
Mudflow – happens when mud falls down a slope quickly.
Rockfall – happens when there are large rocks that are weighted down, and they are being loosened. These rocks can fall down and cause huge problems.
Avalanches – happen when snowfalls are on a steep slope and cause the avalanche to fall to the bottom of the slope.
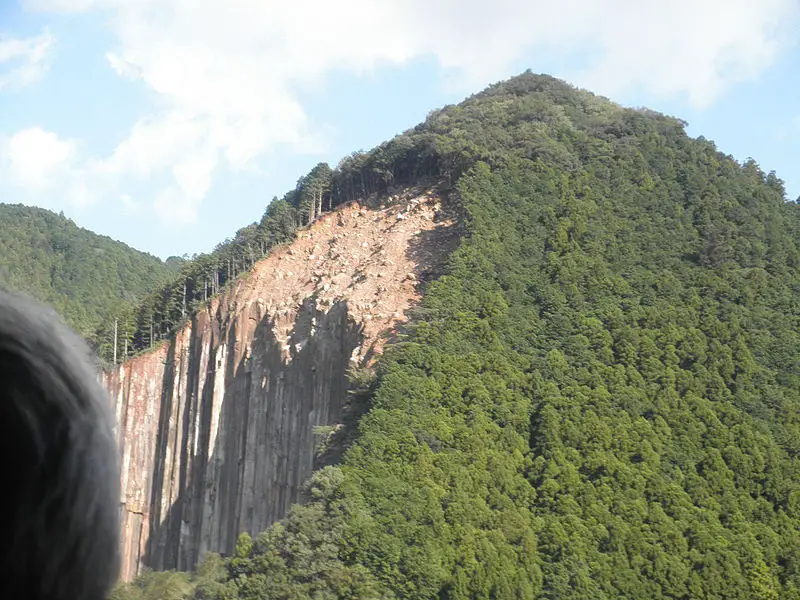
What Did You Learn?
- What is a landslide? A landslide is when rock and debris fall from a steep slope.
- Are landslides dangerous? Landslides can be very dangerous. They can cause damage to homes, property, lakes, rivers and they can even destroy entire towns.
- What causes landslides? Landslides can be caused by erosion, rainfall, melting snow, and more.
- What is a landslide that happens under a body of water called? When a landslide happens under the water like in an ocean or a lake, it is called a submarine landslide.
- Can landslides cause other damage? When a landslide happens, it can cause there to be earthquakes and even tsunamis.
- Back to – Earth Science
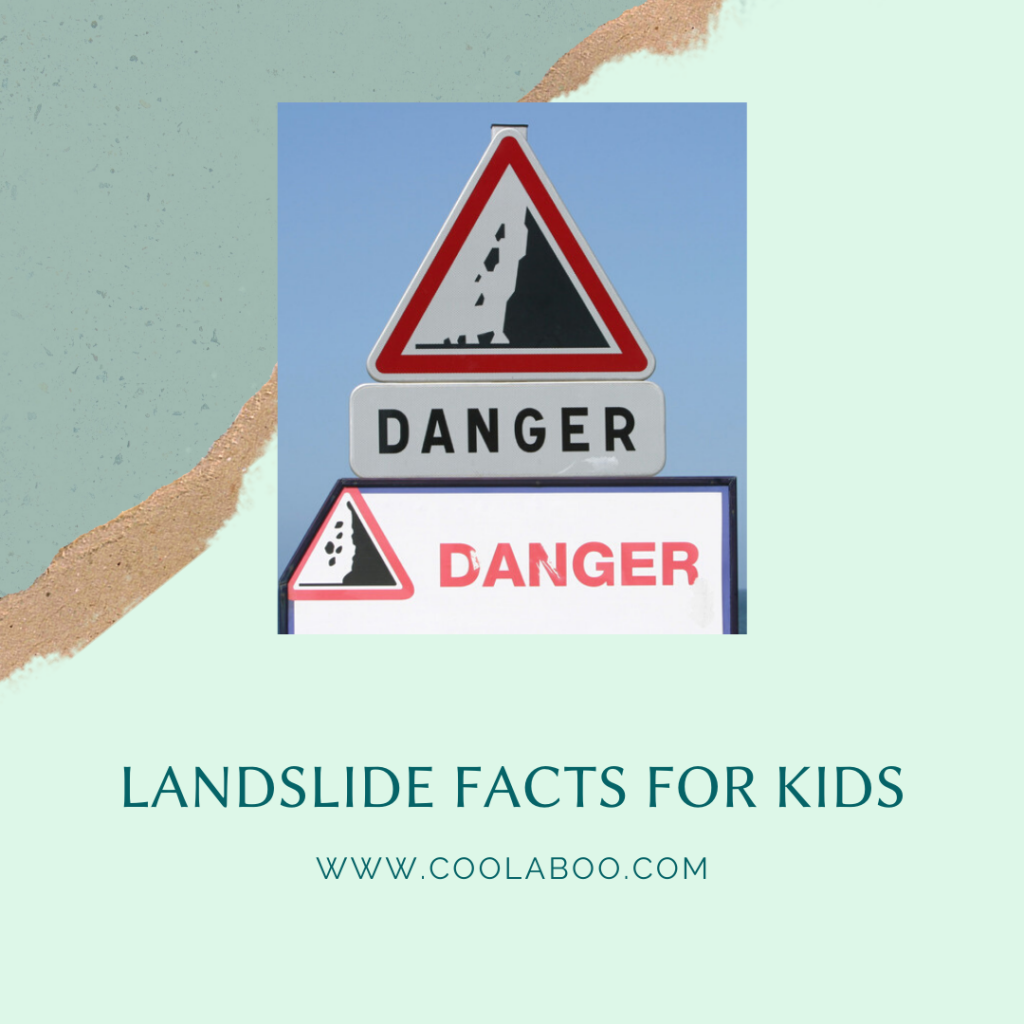
Sage-Answer
Just clear tips and lifehacks for every day
Do all landslides travel fast?
Table of Contents
- 1 Do all landslides travel fast?
- 2 Is a landslide rapid or slow?
- 3 How far can landslides go?
- 4 What signified length of a landslide?
- 5 Can landslides be predicted?
- 6 What country has most landslides?
- 7 When do landslides and debris flow take place?
- 8 How are landslides different from other types of landslides?
How fast do landslide travel? Landslides can move slowly, (millimeters per year) or can move quickly and disastrously, as is the case with debris flows.
Is a landslide rapid or slow?
Soil that moves downhill slowly is said to “creep”, and its movement is often slow and shallow enough to be anticipated and managed with a modest effort. A “translational” landslide can happen rapidly and result in all of the soil on a hillside being stripped off and filling the area at the bottom of the slope.
What is the speed of landslides in KM?
Mudslides like this one are the fastest-moving type of landslide, or “mass wasting.” Mudslides can move at speeds of 80 kilometers (50 miles) per hour.
How far can landslides go?
But large slides (such as the 30-million-cubic-meter flow that occurred in Mesa County, Colorado, and ran more than 4.5 kilometers in 2014, shown) can sometimes travel more than 20 times farther than they fall—and sometimes even, like a fluid, slosh up and over hills. Now, scientists may have figured out why.
What signified length of a landslide?
Measurement of landslide dimensions (L = length, W = width, D = depth) and slope inclination a (°) at the release zone. Shallow landslides triggered off by heavy rainfall are recurrent phenomena on steep slopes. It is widely recognised that vegetation, particularly forest, can stabilize steep slopes.
What was the worst landslide?
Deadliest Landslides In Recorded History
- Huaraz Debris Flows, Ancash, Peru, December 1941 (5,000 deaths)
- 62 Nevado Huascaran Debris Fall, Ranrahirca, Peru, January 1962 (4,500 deaths)
- Khait Landslide, Tajikstan, July 1949 (4,000 deaths))
- Diexi Slides, Sichuan, China, August 1933 (3,000+ deaths)
Can landslides be predicted?
To be able to predict landslides, scientists have developed slope stability models to analyze the risk locally. More recently, NASA has created a preliminary algorithm to map landslide hazards globally using satellite measurements of rainfall, land cover and other surface variables.
What country has most landslides?
Countries where there are frequent landslides include China, the western United States, Italy, Switzerland, Japan, the Philippines and Indonesia. Countries that straddle the Himalayas such as Nepal, Pakistan and India are also areas where there are a large number of landslides.
How many meters per second do landslides move?
When do landslides and debris flow take place?
How are landslides different from other types of landslides.
When do most landslides occur in Washington State?
Privacy Overview
Battered Wayfarers Chapel must be completely dismantled to be saved
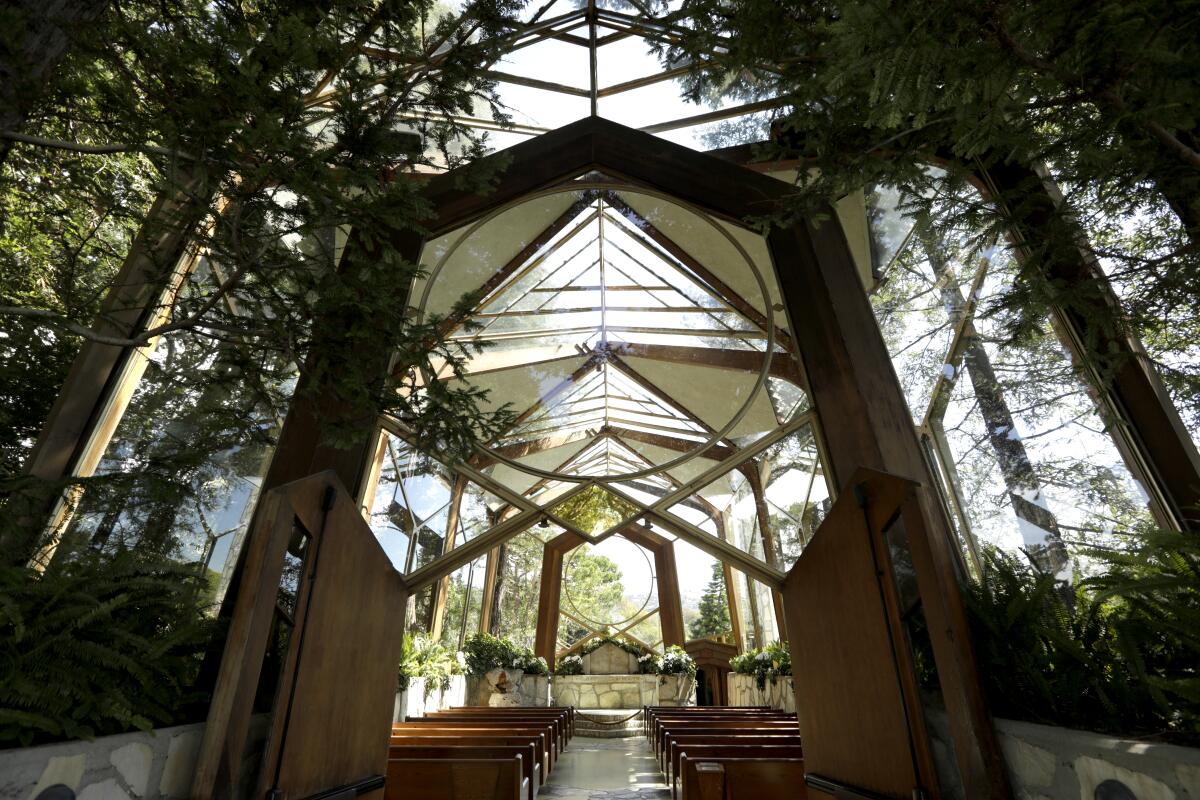
- Show more sharing options
- Copy Link URL Copied!
Each day, landslide damage at the historic Wayfarers Chapel in Rancho Palos Verdes worsens.
More windows in the famous glass chapel shatter. Metal framing along its walls and ceiling further torque. New fissures open across the parking lot.
The landslide beneath the chapel — mostly manageable for decades — has accelerated to unprecedented rates and is likely to upend the possibility of a future for the chapel at its idyllic seaside site.
Chapel leaders announced on Monday their plans to begin taking the structure apart. The hope, they said, is to preserve what they can of the national historic landmark , spiritual sanctuary and well-known wedding venue.
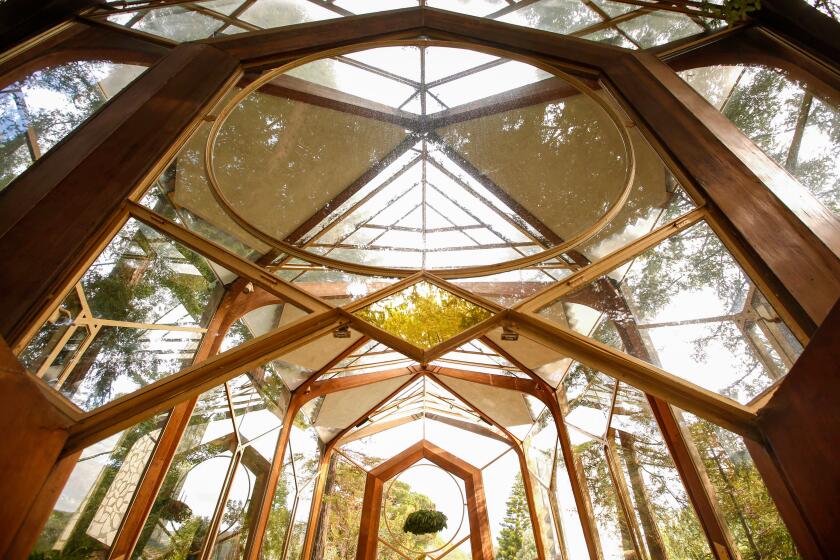
Wayfarers Chapel looks to relocate due to threatening landslide
Wayfarers Chapel officials are working to find a new location in Rancho Palos Verdes after an intensifying landslide has upended any future at its current one.
April 4, 2024
“We are taking immediate action to carefully disassemble the chapel’s historic materials as a necessary step in the preservation of the chapel for generations to come,” Dan Burchett, the executive director of Wayfarers Chapel, said in a statement. “Wayfarers is committed to preserving our iconic chapel exactly as it has always been, either on the current site or a similar site close by in Rancho Palos Verdes.”
Burchett and his team have been searching for a nearby location — on more stable ground — where the chapel could be rebuilt in as close to its original form as possible. He said they would also continue to monitor the landslide to see whether the chapel could be reassembled on-site — but that continues to look less feasible by the day as the land movement has intensified.
In February, Wayfarers closed its doors, worried about safety due to the landslide. Last month, city officials red-tagged the administration building that sits not far from Wayfarers Chapel, and as of Monday, all the underground services for the site, including electricity, water, sewer and gas, were broken and unusable, officials said.
The 100-seat glass-and-wood sanctuary was built in 1951, designed by architect Lloyd Wright, son of Frank Lloyd Wright.
Disassembly, carried out by preservation design firm Architectural Resources Group , will be a tedious process, Burchett said. This week, the team is preparing the property for the large-scale project, and Burchett expected work to begin next week.
“The chapel will not be able to withstand much more damage before it becomes impossible to preserve,” Wayfarers officials said in a news release. “It has been determined that the immediate deconstruction of the chapel is the safest and most viable preservation action to take at this time and will prevent further irreparable damage to the chapel’s structure and materials.”
Many of the chapel’s building materials are no longer available, Burchett said, so deconstruction allows the structure to keep its historical designation and paves the way for “a future careful and thoughtful rebuilding of the chapel.”
“With each passing day, more of this material is lost or irreparably damaged,” said Katie Horak, a principal at Architectural Resources Group. With deconstruction set to begin, “our team is working against the clock to document and move these building components to safety so that they can be put back together again.”
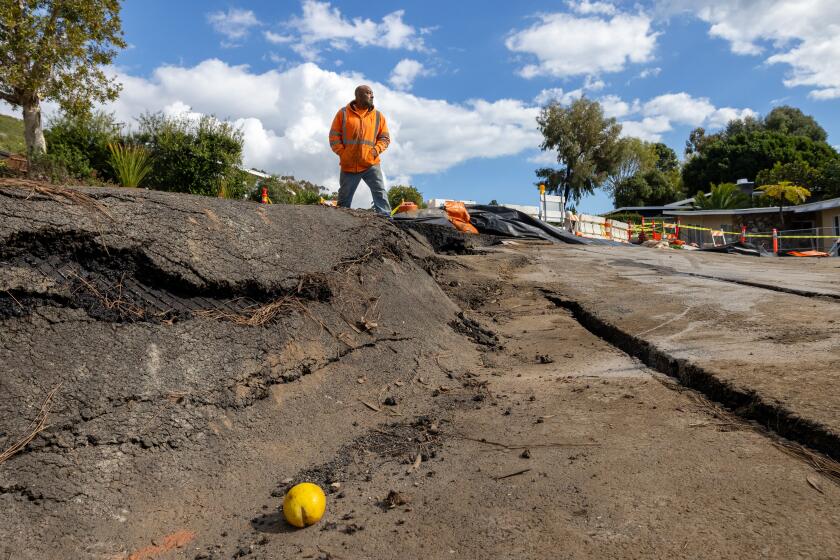
Winter rains fuel ‘unprecedented’ acceleration, expansion of landslides in Rancho Palos Verdes
More and faster land movement has wreaked havoc across Portuguese Bend on the Palos Verdes Peninsula, where a slow-moving landslide has lurked for decades.
Feb. 18, 2024
She said some of the irreplaceable parts include old-growth-redwood glulam ( laminated timber bonded with adhesive), blue roof tile and the elegant network of steel that holds the windows together.
The city’s latest report on the historical landslide complex, which affects about 700 acres on both sides of Palos Verdes Drive South in Rancho Palos Verdes, found that land movement in March and April had further accelerated, almost two times the movement that was recorded from January through March — when leaders were already sounding the alarms about the situation. In some of the fastest-moving areas, the hillside was shifting as much as 9 inches per week, the city’s geologist found.
“Wayfarers Chapel has been a treasured part of our community for generations,” Rancho Palos Verdes Mayor John Cruikshank said in a statement. “The city ... is committed to working with Wayfarers Chapel to ensure it can be quickly rebuilt on a geologically safe location somewhere within the city, if possible.”
Burchett said the deconstruction and closing of the campus is estimated to cost $300,000 to $500,000 — well beyond the almost $70,000 raised through an online fundraiser that was started after the chapel had to close and cease most of its operations.
The full rebuild is estimated to cost nearly $20 million, Burchett said.
The nonprofit has about $5 million in savings reserved for that effort, revenue primarily from weddings at the site. Couples would pay more than $5,000 to marry at the highly sought-after Instagram-famous chapel.
Burchett said Wayfarers would need additional community support, and is planning a fundraising drive for the rest.
More to Read
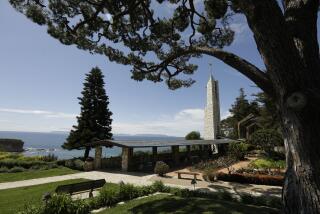
Patt Morrison: Palos Verdes Peninsula landslides can tell us a lot about L.A. history
May 3, 2024
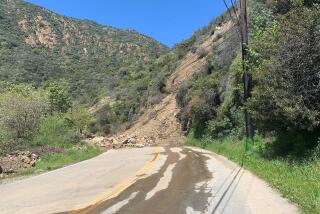
Topanga Canyon could remain closed into the fall after massive landslide
April 19, 2024
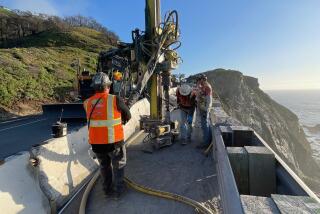
Highway 1 collapse in Big Sur was caused by weather, waves, gravity. That’s the good news
April 16, 2024
Start your day right
Sign up for Essential California for news, features and recommendations from the L.A. Times and beyond in your inbox six days a week.
You may occasionally receive promotional content from the Los Angeles Times.
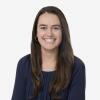
Grace Toohey is a reporter at the Los Angeles Times covering breaking news for the Fast Break Desk. Before joining the newsroom in 2022, she covered criminal justice issues at the Orlando Sentinel and the Advocate in Baton Rouge. Toohey is a Maryland native and proud Terp.
More From the Los Angeles Times
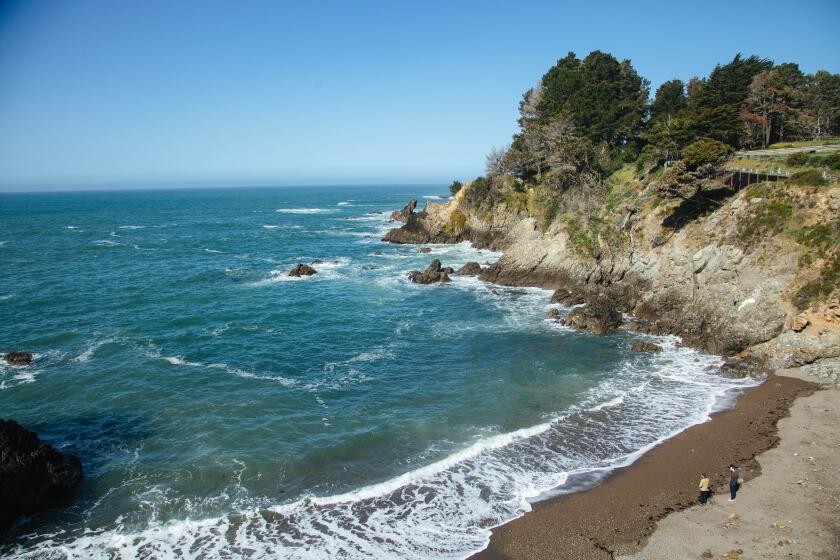
DNA helps identify woman whose body was found at bottom of Bay Area cliff nearly 60 years ago
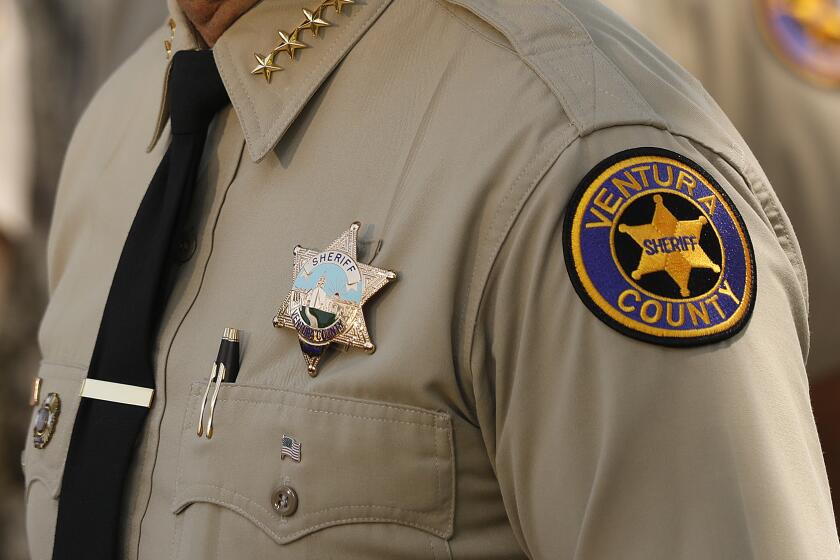
Ventura County high school student accused of falsely reporting gunman on campus
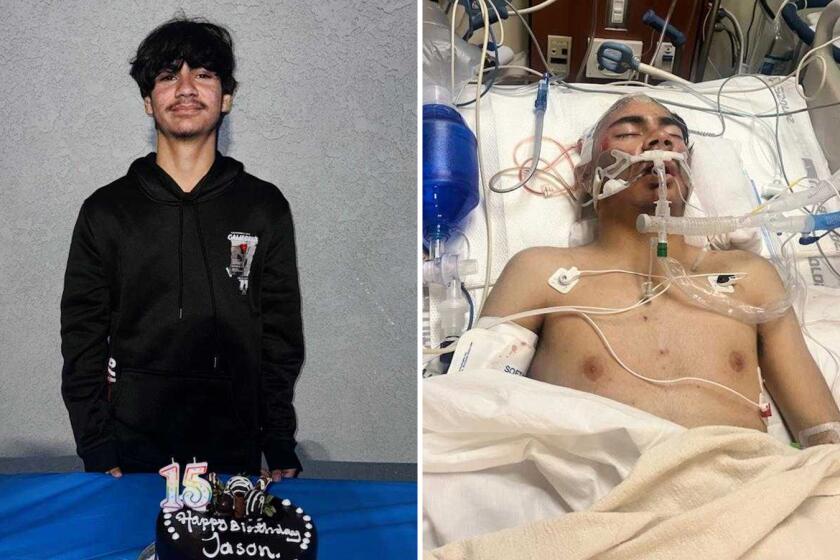
Watts teen attacked while eating a burger; teenage suspect is arrested
May 17, 2024
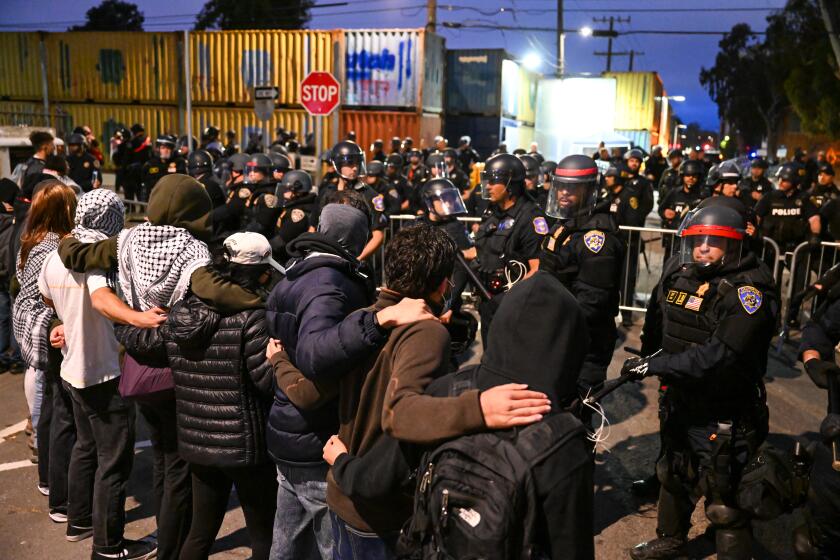
Pro-Palestinian group takes over UC Berkeley building; university calls it ‘crime scene’
NASA - National Aeronautics and Space Administration
Log in to glopac.
- Create new account
- Request new password
Search form
- Video about ESPO
- Deployment Sites
- Flight Request
- ASP Mission Tool Suite
- GloPac Home
- Mission Calendar
- Plan of the Day
- Forecasting
- Lab Layouts
- Science Overview
- Flight Information
- Archive Information
- Global Hawk
- Participants
- People Photos

Disclaimer: This material is being kept online for historical purposes. Though accurate at the time of publication, it is no longer being updated. The page may contain broken links or outdated information, and parts may not function in current web browsers. Visit https://espo.nasa.gov for information about our current projects.
Life and death of slow-moving landslides
In the most destructive and catastrophic landslide events, rocks, soil and fluids can travel at speeds approaching several tens of metres per second. However, many landslides, commonly referred to as slow-moving landslides, creep at rates ranging from millimetres to several metres per year and can persist for years to decades. Although slow-moving landslides rarely claim lives, they can cause major damage to infrastructure and sometimes fail catastrophically, transitioning into fast-moving landslides that can result in thousands of casualties. In addition, slow-moving landslides are highly erosive features that control the landscape morphology in many mountainous regions (such as the California Coast Ranges or the Apennines). The persistent and long-term motion of slow-moving landslides provides an exceptional opportunity to investigate landslide processes and mechanisms. In this Review, we examine the environmental conditions (such as geology, climate and tectonics) of slow-moving-landslide-prone regions, analyse the forcings (for example, precipitation and groundwater, earthquakes, river erosion, anthropogenic forcings and external material supply) that drive their motion and investigate the subsequent implications of the different forcings on landslide dynamics. We then discuss circumstances in which slowmoving landslides can accelerate rapidly, move large distances or even fail catastrophically. Finally, we provide new perspectives and challenges for future landslide research.
Page Last Updated: July 28, 2020
Page Editor: Alexander Handwerger
NASA Official: Marilyn Vasques
Website Issues: Contact Us
- Budgets, Strategic Plans and Accountability Reports
- Equal Employment Opportunity Data Posted Pursuant to the No Fear Act
- Information-Dissemination Policies and Inventories
- Freedom of Information Act
- Privacy Policy & Important Notices
- NASA Advisory Council
- Inspector General Hotline
- Office of the Inspector General
- NASA Communications Policy
- Contact NASA
- Open Government at NASA
- Accessibility
- Skip to main content
- Keyboard shortcuts for audio player
The huge solar storm is keeping power grid and satellite operators on edge

Geoff Brumfiel
Willem Marx

NASA's Solar Dynamics Observatory captured this image of solar flares early Saturday afternoon. The National Oceanic and Atmospheric Administration says there have been measurable effects and impacts from the geomagnetic storm. Solar Dynamics Observatory hide caption
NASA's Solar Dynamics Observatory captured this image of solar flares early Saturday afternoon. The National Oceanic and Atmospheric Administration says there have been measurable effects and impacts from the geomagnetic storm.
Planet Earth is getting rocked by the biggest solar storm in decades – and the potential effects have those people in charge of power grids, communications systems and satellites on edge.
The National Oceanic and Atmospheric Administration says there have been measurable effects and impacts from the geomagnetic storm that has been visible as aurora across vast swathes of the Northern Hemisphere. So far though, NOAA has seen no reports of major damage.

The Picture Show
Photos: see the northern lights from rare, solar storm.
There has been some degradation and loss to communication systems that rely on high-frequency radio waves, NOAA told NPR, as well as some preliminary indications of irregularities in power systems.
"Simply put, the power grid operators have been busy since yesterday working to keep proper, regulated current flowing without disruption," said Shawn Dahl, service coordinator for the Boulder, Co.-based Space Weather Prediction Center at NOAA.
NOAA Issues First Severe Geomagnetic Storm Watch Since 2005

- LISTEN & FOLLOW
- Apple Podcasts
- Google Podcasts
- Amazon Music
- Amazon Alexa
Your support helps make our show possible and unlocks access to our sponsor-free feed.
"Satellite operators are also busy monitoring spacecraft health due to the S1-S2 storm taking place along with the severe-extreme geomagnetic storm that continues even now," Dahl added, saying some GPS systems have struggled to lock locations and offered incorrect positions.
NOAA's GOES-16 satellite captured a flare erupting occurred around 2 p.m. EDT on May 9, 2024.
As NOAA had warned late Friday, the Earth has been experiencing a G5, or "Extreme," geomagnetic storm . It's the first G5 storm to hit the planet since 2003, when a similar event temporarily knocked out power in part of Sweden and damaged electrical transformers in South Africa.
The NOAA center predicted that this current storm could induce auroras visible as far south as Northern California and Alabama.
Extreme (G5) geomagnetic conditions have been observed! pic.twitter.com/qLsC8GbWus — NOAA Space Weather Prediction Center (@NWSSWPC) May 10, 2024
Around the world on social media, posters put up photos of bright auroras visible in Russia , Scandinavia , the United Kingdom and continental Europe . Some reported seeing the aurora as far south as Mallorca, Spain .
The source of the solar storm is a cluster of sunspots on the sun's surface that is 17 times the diameter of the Earth. The spots are filled with tangled magnetic fields that can act as slingshots, throwing huge quantities of charged particles towards our planet. These events, known as coronal mass ejections, become more common during the peak of the Sun's 11-year solar cycle.
A powerful solar storm is bringing northern lights to unusual places
Usually, they miss the Earth, but this time, NOAA says several have headed directly toward our planet, and the agency predicted that several waves of flares will continue to slam into the Earth over the next few days.
While the storm has proven to be large, predicting the effects from such incidents can be difficult, Dahl said.
Shocking problems
The most disruptive solar storm ever recorded came in 1859. Known as the "Carrington Event," it generated shimmering auroras that were visible as far south as Mexico and Hawaii. It also fried telegraph systems throughout Europe and North America.

Stronger activity on the sun could bring more displays of the northern lights in 2024
While this geomagnetic storm will not be as strong, the world has grown more reliant on electronics and electrical systems. Depending on the orientation of the storm's magnetic field, it could induce unexpected electrical currents in long-distance power lines — those currents could cause safety systems to flip, triggering temporary power outages in some areas.
my cat just experienced the aurora borealis, one of the world's most radiant natural phenomena... and she doesn't care pic.twitter.com/Ee74FpWHFm — PJ (@kickthepj) May 10, 2024
The storm is also likely to disrupt the ionosphere, a section of Earth's atmosphere filled with charged particles. Some long-distance radio transmissions use the ionosphere to "bounce" signals around the globe, and those signals will likely be disrupted. The particles may also refract and otherwise scramble signals from the global positioning system, according to Rob Steenburgh, a space scientist with NOAA. Those effects can linger for a few days after the storm.
Like Dahl, Steenburgh said it's unclear just how bad the disruptions will be. While we are more dependent than ever on GPS, there are also more satellites in orbit. Moreover, the anomalies from the storm are constantly shifting through the ionosphere like ripples in a pool. "Outages, with any luck, should not be prolonged," Steenburgh said.

What Causes The Northern Lights? Scientists Finally Know For Sure
The radiation from the storm could have other undesirable effects. At high altitudes, it could damage satellites, while at low altitudes, it's likely to increase atmospheric drag, causing some satellites to sink toward the Earth.
The changes to orbits wreak havoc, warns Tuija Pulkkinen, chair of the department of climate and space sciences at the University of Michigan. Since the last solar maximum, companies such as SpaceX have launched thousands of satellites into low Earth orbit. Those satellites will now see their orbits unexpectedly changed.
"There's a lot of companies that haven't seen these kind of space weather effects before," she says.
The International Space Station lies within Earth's magnetosphere, so its astronauts should be mostly protected, Steenburgh says.
In a statement, NASA said that astronauts would not take additional measures to protect themselves. "NASA completed a thorough analysis of recent space weather activity and determined it posed no risk to the crew aboard the International Space Station and no additional precautionary measures are needed," the agency said late Friday.

People visit St Mary's lighthouse in Whitley Bay to see the aurora borealis on Friday in Whitley Bay, England. Ian Forsyth/Getty Images hide caption
People visit St Mary's lighthouse in Whitley Bay to see the aurora borealis on Friday in Whitley Bay, England.
While this storm will undoubtedly keep satellite operators and utilities busy over the next few days, individuals don't really need to do much to get ready.
"As far as what the general public should be doing, hopefully they're not having to do anything," Dahl said. "Weather permitting, they may be visible again tonight." He advised that the largest problem could be a brief blackout, so keeping some flashlights and a radio handy might prove helpful.
I took these photos near Ranfurly in Central Otago, New Zealand. Anyone can use them please spread far and wide. :-) https://t.co/NUWpLiqY2S — Dr Andrew Dickson reform/ACC (@AndrewDickson13) May 10, 2024
And don't forget to go outside and look up, adds Steenburgh. This event's aurora is visible much further south than usual.
A faint aurora can be detected by a modern cell phone camera, he adds, so even if you can't see it with your eyes, try taking a photo of the sky.
The aurora "is really the gift from space weather," he says.
- space weather
- solar flares
- solar storm
Follow Polygon online:
- Follow Polygon on Facebook
- Follow Polygon on Youtube
- Follow Polygon on Instagram
Site search
- Dragon’s Dogma 2
- Ghost of Tsushima
- Zelda: Tears of the Kingdom
- Baldur’s Gate 3
- GTA 5 cheats
- PlayStation
- Dungeons & Dragons
- Magic: The Gathering
- Board Games
- All Tabletop
- All Entertainment
- What to Watch
- What to Play
- Buyer’s Guides
- Really Bad Chess
- All Puzzles
Filed under:
What are eggs for in Animal Well and how to unlock fast travel
Eggs-plore more with fast travel
Share this story
- Share this on Facebook
- Share this on Reddit
- Share All sharing options
Share All sharing options for: What are eggs for in Animal Well and how to unlock fast travel
/cdn.vox-cdn.com/uploads/chorus_image/image/73336549/Animal_Well_eggs_cover.0.png)
You’ll start finding eggs pretty early in Animal Well , but it’ll take a while before you figure out what they’re for — namely, they’re like keys for a specific room in Animal Well ’s map, which allows you to access new areas.
Our Animal Well guide will explain how to use eggs and how they relate to fast travel.
How to use eggs in Animal Well
:no_upscale()/cdn.vox-cdn.com/uploads/chorus_asset/file/25437805/Animal_Well_eggs_fast_travel_5.jpg)
You’ll find your first egg almost immediately in Animal Well . When you wake up, head to the left. There’s a hidden passage in the wall there that will lead you to your first egg — the Clover Egg — in a chest.
It’ll drop into your inventory off to the side where you can’t interact with it. It’ll take a bit of exploring before you can understand how they’re used.
:no_upscale()/cdn.vox-cdn.com/uploads/chorus_asset/file/25437762/Animal_Well_early_map_copy.png)
After you get past the first ghost enemy , you’ll find the map and then climb up to (what we’re calling) the egg room . This is where you’ll get the stamps for your map. We’re not going to walk you through finding the first handful of eggs, but they are marked on the map above — specifically, eight of them are. And that’s because…
How to use eggs in the egg room
:no_upscale()/cdn.vox-cdn.com/uploads/chorus_asset/file/25437771/Animal_Well_egg_room.png)
When you enter the egg room, you’ll see a peacock standing over a set of shelves with all the eggs you’ve found arranged on them. More importantly, off to the left, you’ll see a set of four doors. The bottom one has eight dots on it, the second one up has 16, the third one has 32, and the topmost one has 64.
Those dots correspond to the number of eggs you’ve found. Once you’ve found that number of eggs, the door will open automatically. The first door — that opens when you find eight eggs — leads to a way to unlock fast travel (more on this below), but we don’t know what the others lead to.
How to unlock fast travel in Animal Well
:no_upscale()/cdn.vox-cdn.com/uploads/chorus_asset/file/25437796/Animal_Well_eggs_fast_travel_2.jpg)
Once you have eight eggs, head to the egg room and you’ll be able to go through the bottom-most door. A couple screens to the left, you’ll find a dreamy room with a few sleeping chinchillas.
Hop up the stairs on the left and use them to jump to the chest in the upper left. Inside, you’ll find the animal flute .
Play your new flute to wake up the chinchillas, and they’ll walk toward you (when you’re on the same vertical level as they are). Use your new chinchilla friends to climb up the stairs heading right. Alternately, you can just use the bubble wand if you have it.
:no_upscale()/cdn.vox-cdn.com/uploads/chorus_asset/file/25437797/Animal_Well_eggs_fast_travel_3.jpg)
In the next room, you’ll find an altar-thing. When you stand in front of it, the eyes on the crow head to the left with light up. Keep standing there, and play the animal flute. The bird’s beak (mouth?) will open.
Hop up to it and go inside.
:no_upscale()/cdn.vox-cdn.com/uploads/chorus_asset/file/25437798/Animal_Well_eggs_fast_travel_4.jpg)
You’ll come to a new room with several animal heads and another altar. Jump down to the altar and play your flute again. This will open any of the animal heads you’ve found so far, making this room a fast travel hub of sorts.

The next level of puzzles.
Take a break from your day by playing a puzzle or two! We’ve got SpellTower, Typeshift, crosswords, and more.
Sign up for the newsletter Patch Notes
A weekly roundup of the best things from Polygon
Just one more thing!
Please check your email to find a confirmation email, and follow the steps to confirm your humanity.
Oops. Something went wrong. Please enter a valid email and try again.
Loading comments...

Challengers, Godzilla x Kong: The New Empire, Madame Web on Netflix, and every new movie to watch at home this weekend

Twitch unbans shaking butts and twerking for animated emotes

Talisman, one of the OG dungeon crawlers, is back with a 5th edition this summer

How to breed Pals in Palworld

Why am I crying because of a damn video game commercial?

Fallout is coming to Fortnite
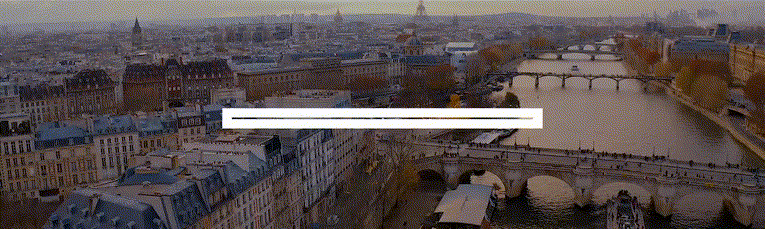
IMAGES
VIDEO
COMMENTS
Landslides are the downslope movement of earth materials (rock, debris, and soil) at rates that range from inches per year to tens of miles per hour. Some landslides can move faster than a person can run. Landslides can happen with no notice or can take place over a period of days, weeks, or longer. Sources/Usage: Public Domain.
Landslides occur in all 50 states and territories, and they affect lives, property, infrastructure, and the environment. Landslides are the downslope movement of earth materials (rock, debris, and soil) at rates that range from inches per year to tens of miles per hour. ... Some fast-moving landslides can travel thousands of feet, even across ...
Small landslides typically flow about twice the distance they've fallen downslope before they run out of energy. But large slides (such as the 30-million-cubic-meter flow that occurred in Mesa County, Colorado, and ran more than 4.5 kilometers in 2014, shown) can sometimes travel more than 20 times farther than they fall—and sometimes even, like a fluid, slosh up and over hills.
A landslide is defined as the movement of a mass of rock, debris, or earth down a slope. Landslides are a type of "mass wasting," which denotes any down-slope movement of soil and rock under the direct influence of gravity. The term "landslide" encompasses five modes of slope movement: falls, topples, slides, spreads, and flows. These are further subdivided by the type of geologic material ...
Landslides are masses of earth, rock, or debris that move down slopes. Landslides are triggered by one event, but many causes can weaken slopes over time and make them more likely to fail when there is a triggering event. These causes can be both natural and artificial. Landslides often occur in areas with oversteepened slopes, weak soils ...
A computer model could explain why some landslides can travel much longer distances than others and cause damage far away from where they started. The work suggests that vibrations generated by large slides can cause tons of rock to flow like a fluid, enabling the rocks to rumble across vast distances.
A landslide is the movement of rock, earth, or debris down a sloped section of land. Landslides are caused by rain, earthquakes, volcanoes, or other factors that make the slope unstable. Geologists, scientists who study the physical formations of Earth, sometimes describe landslides as one type of mass wasting.A mass wasting is any downward movement in which Earth's surface is worn away.
Lesson 8: Landslides Hazards. Earthquakes are a major cause of landslides. Landslides occur when masses of rock, earth material, or debris flows move down a slope due to gravity. Landslides can ...
The toe marks the runout, or maximum distance traveled, of the landslide. In rotational landslides, the toe is often a large, disturbed mound of geologic material, forming as the landslide moves past its original rupture surface. Rotational and translational landslides often have extensional cracks, sag ponds, hummocky terrain, and pressure ridges.
Slow-moving landslides rarely claim lives 5, but fast-moving (m s −1) debris flows can initiate from within the slow-moving landslide mass and inundate large areas 6, 7. In addition, slow-moving ...
Landslides & Debris Flows. Many geologic processes, including those shaping the land surface, are slow-acting, involving feedbacks that operate over many years. But surface processes can be rapid and episodic, too. In the previous lecture, we noted that the erosion of bedrock by river networks is the primary mechanism shaping many landscapes.
Know what to do to protect yourself if you live in an area prone to landslides or mudslides. Landslides. Landslides occur when masses of rock, earth, or debris move down a slope. Mudslides or debris flows. Debris flows, also known as mudslides, are a common type of fast-moving landslide that tends to flow in channels.
Vibrations make large landslides flow like fluid. New research shows why some large landslides travel greater distances across flat land than scientists would generally expect, sometimes putting towns and populations far from mountainsides at risk. Long-runout - This 2014 landslide in Mesa County, Colorado contained 30 million cubic meters or ...
An average of 25-50 people are killed by landslides each year in the United States. The worldwide death toll per year due to landslides is in the thousands. Most landslide fatalities are from rock falls, debris flows, or volcanic debris flows (called lahars). Twenty-three people were killed, at least 167 injured, and more than 400 homes were ...
What is a landslide? A landslide is a mass movement of material, such as rock, earth or debris, down a slope. They can happen suddenly or more slowly over long periods of time. When the force of gravity acting on a slope exceeds the resisting forces of a slope, the slope will fail and a landslide occurs. External factors can lead to landslides ...
In the most destructive and catastrophic landslide events, rocks, soil and fluids can travel at speeds approaching several tens of metres per second. However, many landslides, commonly referred to as slow-moving landslides, creep at rates ranging from millimetres to several metres per year and can persist for years to decades.
1. A historic yet well observed landslide in the East Kootenays of British Columbia took approximately 100 seconds to settle, Frank Slide. The slides at Sierra Leone and the Philippines that you mentioned are a different type of slide made of mud and other unconsolidated materials. I cannot find any information on them but I have witness a few ...
In areas burned by forest and brush fires, a lower threshold of precipitation may initiate landslides. Debris flows, sometimes referred to as mudslides, mudflows, lahars, or debris avalanches, are common types of fast-moving landslides. These flows generally occur during periods of intense rainfall or rapid snow melt.
How Fast Do Landslides Travel? Landslides can travel at a slow rate or a fast rate. Some landslides can travel at: 200 miles per hour. 30-50 miles per hour. There are different things that depend on how fast the landslide will travel such as: How steep the slope is. What type of Earth is on the slope. The angle of the slope.
When and where will landslides happen? How far will they go, how big and how fast will they be? These questions are difficult to answer because many factors contribute to landslide occurrence, magnitude, and mobility; some factors remain unknown, while nearly all are very difficult to quantify and account for. Researchers use surface, subsurface, remote sensing, and laboratory observations ...
A "translational" landslide can happen rapidly and result in all of the soil on a hillside being stripped off and filling the area at the bottom of the slope. What is the speed of landslides in KM? Mudslides like this one are the fastest-moving type of landslide, or "mass wasting.". Mudslides can move at speeds of 80 kilometers (50 ...
Wayfarers Chapel looks to relocate due to threatening landslide. April 4, 2024. "We are taking immediate action to carefully disassemble the chapel's historic materials as a necessary step in ...
In the most destructive and catastrophic landslide events, rocks, soil and fluids can travel at speeds approaching several tens of metres per second. However, many landslides, commonly referred to as slow-moving landslides, creep at rates ranging from millimetres to several metres per year and can persist for years to decades.
The huge solar storm is keeping power grid and satellite operators on edge. NASA's Solar Dynamics Observatory captured this image of solar flares early Saturday afternoon. The National Oceanic and ...
Not all landslides occur as catastrophic events. Some creep along at a snail's pace, stopping and starting, and not advancing more than 1 meter per year, and many of these are called earthflows. Earthflows are extremely common all over the world, in fact, they're much more common than the fast-moving, deadly landslides.
The bottom one has eight dots on it, the second one up has 16, the third one has 32, and the topmost one has 64. Those dots correspond to the number of eggs you've found. Once you've found ...
Build and regularly maintain an emergency kit and create and practice a household evacuation plan that includes your pets. Work with your neighbors to help them understand the hazard. Neighbors living on or below a slope should work together to reduce their landslide risk. Landslides do not respect property lines.