How to Troubleshoot Temperature Excursions?
Download our free temperature excursions poster below detailing everything from this blog including:
- Warning signs of a temperature excursion
- Actions items to take during an excursion event
- Preventative measures you can take
As a primer, we’ve detailed below everything you need to know about temperature excursions so you can be prepared if one happens inside your healthcare facility.
What Is a Temperature Excursion?
In the United States, the CDC defines a temperature excursion as "any break in the cold chain of a pharmaceutical product" . The "cold chain", is a series of refrigerated production , storage and distribution activities. These processes ensure that vaccines and other pharmaceuticals remain at the correct temperature. Maintaining proper temperatures and avoiding excursions ensures that materials remain safe and effective.
Meanwhile, according to the European Compliance Academy , a temperature excursion is defined as: " a deviation from the labeled storage condition of a product for any duration " .
This includes excursions during transportation or distribution.
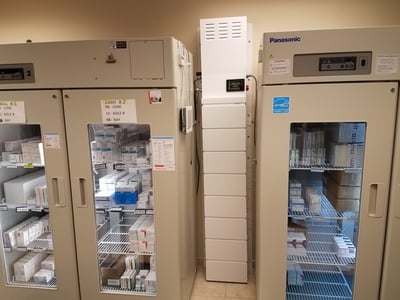
As a result of this degradation, there will be a generation of impurities in the product. Degradation products like this are not wanted in the manufacturing chain. Additionally, they present a threat to patients' health.
As such, the raw materials needed to produce pharmaceuticals need to be maintained as well . Active pharmaceutical ingredients (API) need proper temperatures during the manufacturing process. If not properly handled, they can lose potency, effectiveness and safety before they're even distributed.
Deviating from optimal storage conditions can result in significant changes in the API. This includes degradation, decay, polymerization, and an increase in impurity levels.
Reasons Why Temperature Excursions Occur
Air handling units (AHU) maintain the required temperature in pharmaceutical factories. So, the design and capacity of these units reflect the API manufacturers temperature requirements during the production process. Regardless, temperature excursions are unavoidable. Even in the manufacturing area and during transportation.
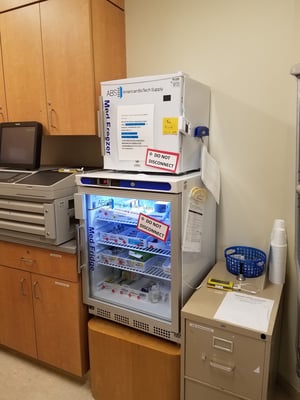
- An insufficient number of air handling units to maintain the desired temperature conditions
- A leaked or ruptured air duct
- Mechanical failure in air handling units
- Unprecedented temperature fluctuations.
- Power outages interrupting the AHU's operation
- Poor adherence to good manufacturing practices (GMP) on the production floor
- Poor staff oversight of temperatures/ Poor Quality Control
- Extreme weather conditions and poor or absent SOPs for handling them
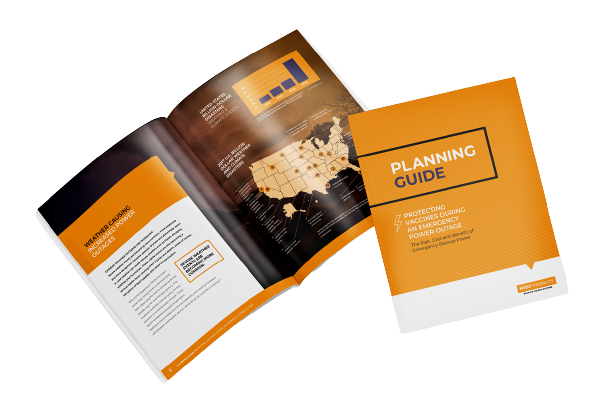
Effects Of Temperature Excursion
A temperature excursion affects product quality in two potential ways. But, the impacts of high temperatures are different than those of temperatures that are too low. Though the result is the same nonetheless - a compromised product.
Products sensitive to high temperatures experience degradation that can cause a decrease in the active ingredient content of the product .
This happens due transformations in the affected, degraded components within. The transformation could be oxidative, hydrolytic, or some other form. As a result, much more toxic variants of the compound begin to appear. The amount of degradation/toxicity is dependent on length and severity of the temperature excursion, which can result in:
- Components becoming discolored.
- Changes in dissolution rates
- Separation of emulsions
Products sensitive to a low temperature usually get damaged by phase changes caused by the freezing process . As a result, the physical atomic structures of the chemicals experience permanent changes.
Products with large quantities of water, like creams or biologicals, are especially prone. Often, they'll lose their properties after experiencing excessive freeze-thaw or temperature cycles. Because water can easily change temperature, the presence of ice quickly damages product.
How To Measure Temperature Excursions
One responsibility of pharmaceutical manufacturers is guaranteeing every batch is a safe and effective high quality product. One of the ways to make this happen is through continuous temperature monitoring. By monitoring the temperature range, they can catch any excursions that occur. After which, they can take the necessary steps to address them immediately.
The Center for Disease Control recommends using a continuous temperature monitoring device (TMD) to track excursions . The recommended TMD is a digital data logger (DDL) that has the following characteristics:
- Ability to record the logged temperature values to a computer system for review.
- The digital data logger's calibration status must be regularly verified, with up-to-date software. This ensures accurate information about storage conditions.
The following information is required when documenting temperature excursions:
- The date and time the temperature excursion occurred
- An inventory of affected products
- The storage unit air temperatures. Including the minimum and maximum temperatures observed during the temperature excursion, if available
- Ambient temperature (also referred to as “room temperature”).
- A general description of the event, such as the length of the temperature event. The digital data logger should provide this data.
- A list of any other items in the storage unit
- Documentation of any problems with the storage unit
All the data should be compiled, and a copy given to the distributor and recipient of the affected product(s).
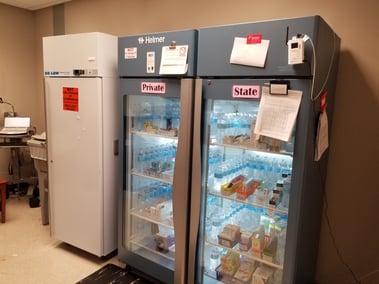
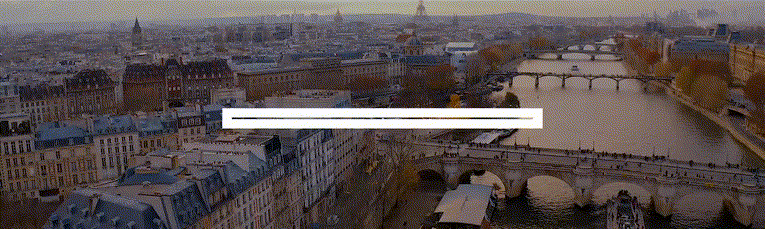
How To Avoid and Combat Temperature Excursions
Simply put, any temperature excursion is a result of prolonged exposure to air that is room temperature or above. For end users of pharmaceuticals, the most likely scenario for them to experience a temperature excursion is because they’ve left the door open to a medical refrigerator/medical freezer or it has stopped running.
If Your refrigerator/freezer temperature has dropped below 35°F (2.0°C) for 15 or more minutes consecutively:
- Check the Placement of the Thermometer probe – Place in the middle and monitor temperature in 30-minute intervals
- Adjust Refrigerator/Freezer Temperature - Change temperature of appliance, if possible, to a warmer setting. Monitor and record = temperature every 30 minutes for next 2 hours.
- If temperatures remain out of range — implement facilities’ relocation plan. Immediately call vaccine manufacturer/distributor.
If your Refrigerator has risen above 46°F (7.7°C) or Freezer has risen above 5°F(-15°C) for 60 minutes or more consecutively:
- Check your power supply — if your area is experiencing a power outage estimated to last 2 or more hours and your facility does NOT have a dedicated backup power source for your cold storage appliances, implement emergency relocation plan
- Check the door to the storage unit — ensure nothing is preventing door from securely closing. If so, adjust accordingly and shut door completely.
- Adjust the refrigerator/freezer temperature — Change temperature of appliance to a cooler temperature is possible. Monitor and record temperature every 30 minutes for next 2 hours.
- If temperatures remain out of range — implement facilities’ relocation plan. Immediately call vaccine manufacturer/distributor. In short, unless total failure of the appliance has occurred, the most common solutions to these problems are:
- A door alarm to ensure staff always close it
- A backup power solution to ensure any medical and pharmaceutical refrigerators continue to operate as normal.
While door alarms are fairly easy to source tools, a reliable and powerful backup power system for refrigerators can be harder to find.
Luckily, battery backup systems offer instant and automatic power for medical appliances as soon as the power goes out. As a result, no staff needs to be on-site to keep track of or start the generator and vaccines will continue to remain safe—with no extra work required.
Additionally, their vertical, cabinet-like design and leak-proof batteries mean they can be installed in even the tightest spaces and oriented in anyway to make them fit. Plus, if your medication or vaccine room is truly tight on space, a hardwired backup power unit can instantly supply remote power to your appliance—directly via the outlet its already plugged into.
Regardless of what kind of system is the best fit, they ensure that your entire stock of vaccines are protected from a sudden loss of power (and the resulting temperature excursions) by guaranteeing a seamless transition from utility power to backup power.
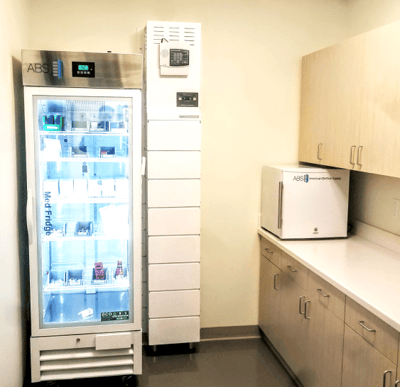
- Over a weekend
- Or even for a whole week.
So, to protect your facility from tens of thousands of dollars in lost vaccine stock, speak to a Medi-Products battery backup expert.
They’ll help design you a system that both meets your power needs and will fit inside your facility—for a much lower cost than what your vaccines are worth. So a backup power system pays for itself the first time your power goes out.
Designing a system for you is as easy as taking a picture of your appliance’s nameplate, and a photo of the room where it’s in.
Then, you just email both photos to our Product experts, and we’ll provide you with multiple options for backup power protection.
For more information contact:
1.800.7653237
Battery Backup for Vaccine Refrigerators and Freezers.
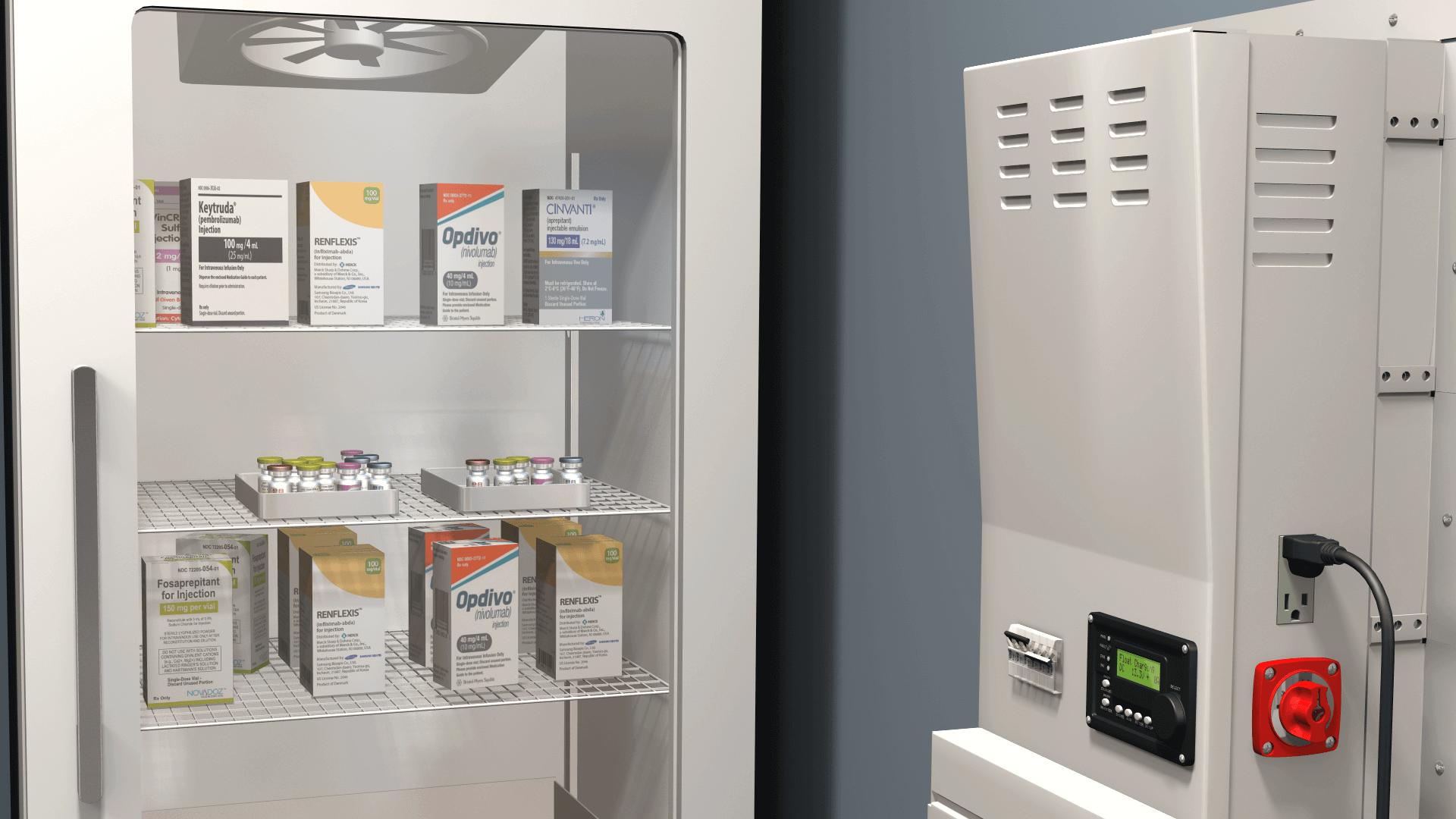
Our powerful battery backup systems will instantly power multiple appliances during a power outage. These custom sized systems can provide power for up to 72 hours of runtime!
POPULAR QUESTIONS
Blog categories.
- Healthcare Compliance (30)
- Healthcare Management (26)
- Healthcare Storage (22)
- Healthcare Design (21)
- Vaccine Storage (20)
- Backup Generators (18)
- Power Outages (18)
- Energy-Efficient Solutions (1)
VISIT OUR LEARNING CENTER
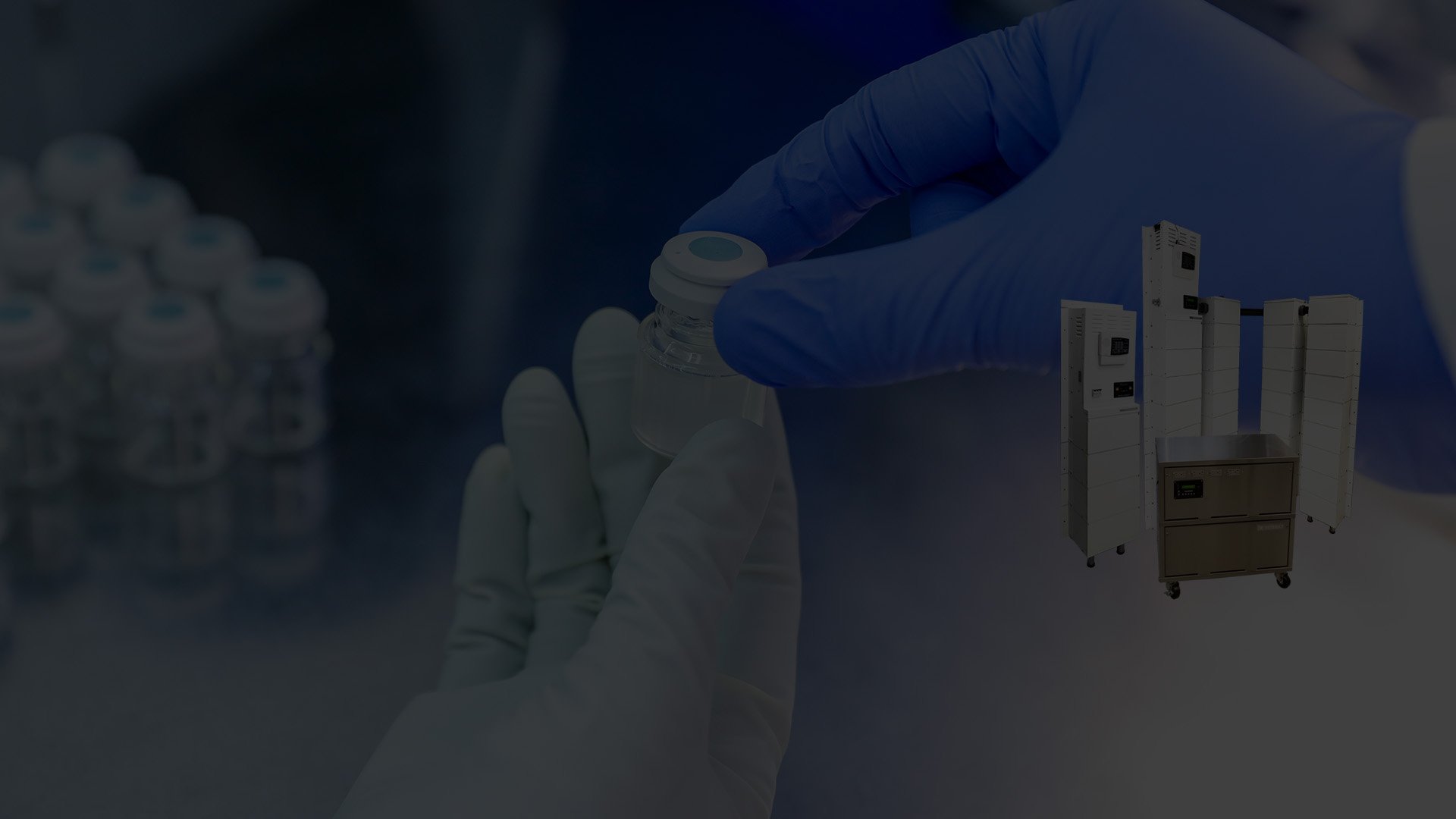
We would like to hear from you
Our sales and technical support staff are available 8-5 EST, Mon-Fri
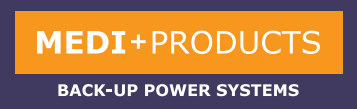
Temperature Excursion Requirements for Refrigerated Medications
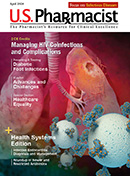
Related Content
A review of infused allergy treatments, fda grants accelerated approval for controversial novel alzheimer’s drug, new guidelines shore up smart infusion pump safety, convalescent plasma for covid-19 shows promise earlier in illness for elderly.

- Advertising Contacts
- Editorial Staff
- Professional Organizations
- Submitting a Manuscript
- Privacy Policy

- Classifieds
Comprehensive Temperature Excursion Management Program for the Commercial Distribution of Biopharmaceutical Drug Products
Affiliations.
- 1 Biopharmaceutical Product Sciences, GlaxoSmithKline, 1250 S Collegeville Road, Collegeville, Pennsylvania 19425. Electronic address: [email protected].
- 2 Biopharmaceutical Product Sciences, GlaxoSmithKline, 1250 S Collegeville Road, Collegeville, Pennsylvania 19425.
- PMID: 32315663
- DOI: 10.1016/j.xphs.2020.04.006
Biopharmaceutical drug products may be exposed to temperatures outside of the intended storage temperature range (typically 2-8°C) during commercial distribution due to uncontrolled variables and unexpected events. Pharmaceutical companies are expected to ensure that product quality and stability are not negatively impacted by temperature excursions defined as being acceptable for the product. It is imperative that all firms involved in the distribution understand key elements of the temperature excursion management program in place to overcome the challenges of global distribution and comply with regulatory requirements. Proactive implementation of a comprehensive temperature excursion management program is expected to help achieve successful commercial distribution. In this article, important aspects related to the key elements of a comprehensive temperature excursion management program are summarized, including standard stability testing, regulatory expectations related to the justification of temperature excursions, thermal cycling studies to assess and support potential temperature excursions (including how/when thermal cycling study data is used to support temperature excursions), good distribution practices to minimize temperature excursions and use of theoretical methods/mathematical simulation models to assess temperature excursions. A comprehensive temperature excursion management program is expected to ensure product quality and help minimize, assess, and justify temperature excursions more efficiently, ensure regulatory compliance and avoid business impact caused by the loss of products or inadequate supply.
Keywords: Biopharmaceutical drug product; Commercial distribution; Good distribution practices; Mean kinetic temperature; Regulatory expectations; Stability testing; Temperature excursion; Thermal cycling; mAb.
Copyright © 2020 American Pharmacists Association®. Published by Elsevier Inc. All rights reserved.
- Biological Products*
- Pharmaceutical Preparations*
- Temperature
- Biological Products
- Pharmaceutical Preparations
Explore how our CDMO services can take your biologic further at BIO 2024. Schedule a meeting.
Managing Temperature Excursions: Key Pointers That Must Be Addressed
The maintenance of cold chain product integrity across the entire supply chain demands rigorous processes and cold chain expertise of the highest caliber—from packaging, handling, storage and distribution of temperature sensitive Investigational Medicinal Products (IMP), all the way to the investigator site.
Part 4 clearly outlines what happens when a temperature excursion occurs. Top tips are given on the best course of action to take.
Discover what to do when a temperature excursion occurs and get summary recommendations for sponsors on how to handle cold chain or temperature sensitive IMP across the supply chain, up until delivery to the investigator site.
Ready to discuss your project?
Connect with us
Temperature Excursion Management – A Novel Approach Of Quality System In Pharmaceutical Industry
Authors: nirmal kumar, ajey jha.
Quality of pharmaceutical product largely depends upon by the environment controls during its storage and handling. Each pharmaceutical product should be handled and stored under specified storage condition labelled on product information data sheet or product pack. Hence the temperature excursions during receipt of raw materials, manufacturing of pharmaceutical products and distribution should be managed during entire product life cycle with holistic approach. The research is based on primary data and exploratory study through literature review. The temperature excursion may be observed during transportation of raw materials manufacturing as well as distribution of pharmaceutical products, which have potential to deteriorate the product quality. Temperature excursion in pharmaceutical industry should be recorded and reported to the manufacturer for further investigation and risk analysis. The concept of temperature excursions, its reasons, consequences and handling mechanism should be well understood to ensure the concerted efforts under the aegis of Quality Management System. Based on the reasons and consequences of temperature excursions during pharmaceutical operations, a system based quality management has been envisaged through this study. The concept an d procedure to handle temperature excursion have evolved after this study which shall be useful to pharmaceutical industry as well as to medicine distributors and consumers.
GMP; Temperature excursions management; Formulation; Quality by Design; Degradation products
Citation: Kumar, N., Jha, A., Temperature excursion management – A novel approach of quality system in pharmaceutical industry, Saudi Pharmaceutical Journal(2016),doi: http://dx.doi.org/10.1016/j.jsps.2016.07.001
Received: 12 December 2015, Accepted: 1 July 2016, Available online: 9 July 2016
Copyright: © 2016 Production and hosting by Elsevier B.V. on behalf of King Saud University.Open Access funded by King Saud University
Temperature excursion is a general term that represents the environmental excursions. However, there is a need of holistic approach to handle the temperature excursions starting from raw material manufacturing site to medicine retailers shop to protect quality of product. The temperature excursion at any stage of pharmaceutical business operation must be reported as soon as possible and investigated appropriately. The consequences of deviation against temperature and humidity limits should be studied appropriately by quality assurance personnel. The risk of temperature excursions cannot be ruled out, but it can be minimized through effective system. Alternative is to use thermal resistant packaging and stringent control measures during transit and shipment, to avert the undesired quality impact on pharmaceutical product. The systematic approach to handle the issues related temperature excursions become inevitable for pharmaceutical manufacture.
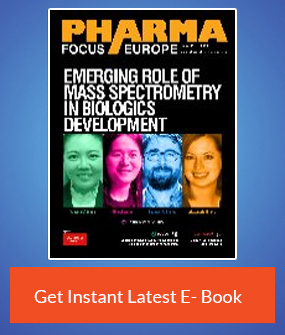
- Instructors
- Getting Help
- Orientation
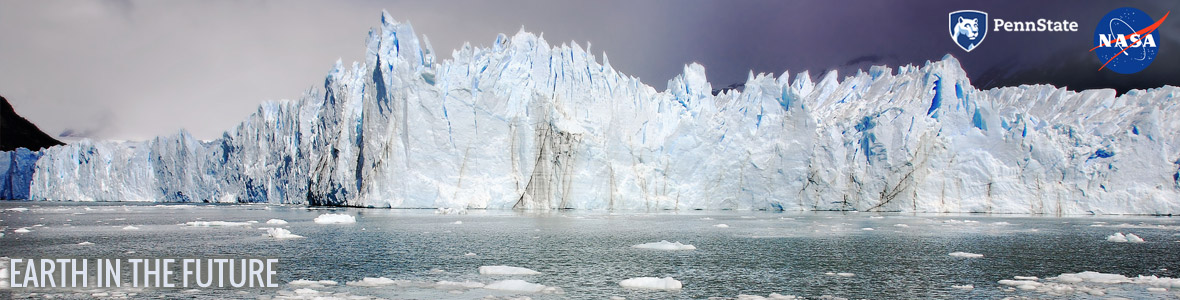
Ancient Climate Events: Paleocene Eocene Thermal Maximum
The Paleocene-Eocene Thermal Maximum (PETM) at 56 million years before present is arguably the best ancient analog of modern climate change. The PETM involved more than 5 o C of warming in 15-20 thousand years (actually a little slower than rates of warming over the last 50 years), fueled by the input of more than 2000 gigatons (a gigaton is a billion tons!) of carbon into the atmosphere. The PETM was associated with the largest deep-sea mass extinction event in the last 93 million years and remarkable diversification of life in the surface ocean and on land. Because of its potential significance, geologists have swarmed to study the event, and it's been the topic of great interest, and more than a little controversy, for the last 25 years. For this reason, also, we devote considerable attention to the event here.
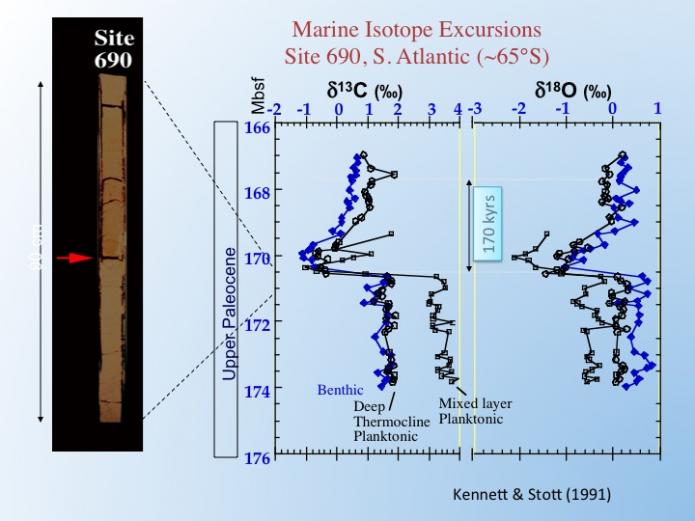
The evidence for warming comes from a variety of sources, the most compelling of which is the oxygen isotopes of planktonic and benthic forams in deep-sea cores. These data suggest significant warming of 6-8 o C of ocean surface waters at a range of latitudes, as well as of deep waters. We don’t need to go into the detail here, but this converts to 4-5 o C of the average Earth temperature, which is pretty significant. For comparison, global warming since the industrial revolution is about 1.2 o C. Today, warming is a result of human activities, but only very primitive primates were around at the time of the PETM, and they did not drive cars (!), so what caused the warming back then?
Corresponding to the oxygen isotope shift is a large and negative 4 to 5 per mil change in carbon isotopes that is used to define the geological extent of the event. The isotope excursion has been identified in sediments deposited in the ocean and those laid down in terrestrial environments such as lakes and rivers. It is called a golden spike because it can be correlated around the world and it marks a precise time horizon, in fact, the excursion is now the formal definition of the boundary between the Paleocene and Eocene eras. Moreover, the excursion in terrestrial sediments allows us to correlate the changes that occurred on land during the PETM with those that took place in the ocean. Below is what the PETM looks like in the Big Horn Basin in Wyoming.
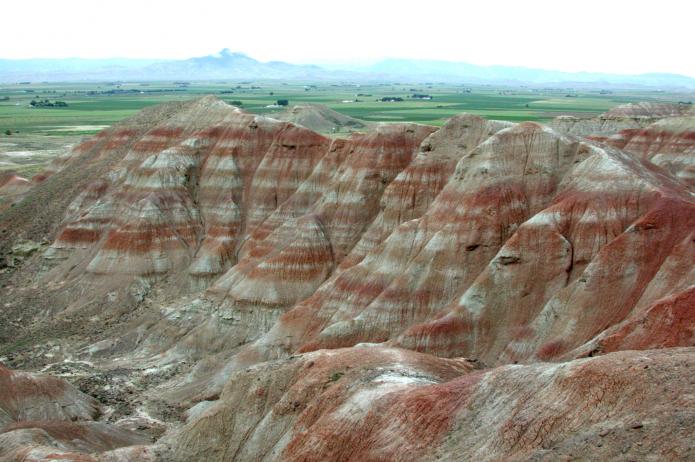
The content of CO 2 in the atmosphere increased 3-4 times during the PETM. Regardless of whether it comes from cars, factories or from non-human sources, CO 2 is a greenhouse gas, and it causes the atmosphere to warm. As a consequence, surface ocean temperatures at the peak of the event were extremely warm, especially in the high latitudes. Off the coast of Antarctica, a location today that is close to freezing, the oceans were about 20 o C (68 o F) at the peak of the PETM! Imagine jumping into the ocean from Antarctica today! Tropical ocean temperatures were really hot. A recent paper indicates that temperatures off the coast of West Africa were 36 o C which is 97 o F! Now I’ve been swimming in Miami in August and it feels like a bathtub at 88 o F, but 97 o F is virtually uninhabitable! The PETM was also associated with major changes in the properties of the deep ocean. Unlike today, when deep ocean waters are characterized by temperatures close to freezing, PETM deep waters were 10-15 o C. This may not seem that warm all considering, but there is no doubt that is caused a fundamental change in the way circulation in the ocean worked. Sea level was quite a bit higher during the PETM, and the continents were in different positions, as shown on the map below.
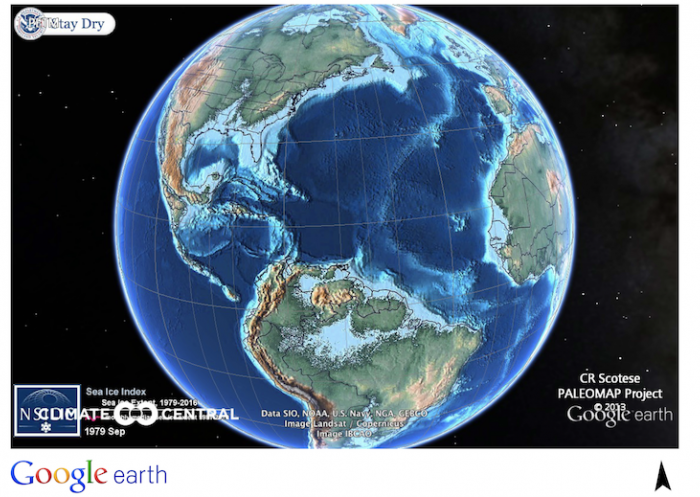
Likely, the cause of these warm deep waters was that they came from different surface ocean locations than they do today (see Module 6 for more detail on the sources of modern deep waters), combined with the warming that took place in the surface ocean. As warmer waters hold less oxygen than cold waters, PETM deep waters in many locations likely were possibly close to a condition that is known as hypoxia (we will learn more about this in Module 6). The word hypoxia does not sound too distressing but imagine for a minute that you are a fish, and you needed oxygen for respiration. Hypoxia would have been truly awful for such a creature! Finally, the input of so much CO 2 into the ocean caused ocean waters to become more acidic and led to a condition known as ocean acidification (sorry to keep jumping ahead, but we will learn a lot more about this in Module 7!). Acidification of the deep ocean during the PETM is well accepted and is observed by complete dissolution of all CaCO 3 shells that rained down on the seafloor. For creatures that require a shell for protection against predators and to protect the soft cellular parts from the harsh ocean, acidification would have been disastrous. By comparison, the shallow ocean experienced a much more minor decrease in its acidity and shelly creatures continued to thrive there.

Paleocene-Eocene Thermal Maximum
- Reference work entry
- Cite this reference work entry
- Gavin A. Schmidt
Part of the book series: Encyclopedia of Earth Sciences Series ((EESS))
542 Accesses
Approximately 55.5 Ma ago in marine and terrestrial sections all around the globe, there was an exceptionally large carbon isotope ( δ 13 C) excursion (CIE) which is unique in the Tertiary for its size and rapidity of its onset. This event, which was first recognized in multiple cores by Kennett and Stott ( 1991 ), is contemporaneous with a significant warm anomaly (and oxygen isotope anomaly) and has thus been named the Paleocene-Eocene Thermal Maximum (PETM) (Figure P8 ). Due to the singular nature of this perturbation and the ecological, faunal and physical gradients across it, the base of this event is now used to define the boundary of the Paleocene-Eocene transition. The previous definition of the P/E boundary was significantly later (at the base of the Ypresian section in Europe), and thus placed the CIE in the late Paleocene. Previously authors had therefore referred to this event as the Late (or Latest) Paleocene Thermal Maximum (LPTM). Some authors have since referred to it as...
This is a preview of subscription content, log in via an institution to check access.
Access this chapter
- Available as PDF
- Read on any device
- Instant download
- Own it forever
- Durable hardcover edition
- Dispatched in 3 to 5 business days
- Free shipping worldwide - see info
Tax calculation will be finalised at checkout
Purchases are for personal use only
Institutional subscriptions
Bibliography
Aubry, M.-P., Berggren, W.A., Stott, L., and Sinha, A., 1996. The upper Paleocene-lower Eocene stratigraphic record and the Paleocene-Eocene boundary carbon isotope excursion: Implications for geochronology. In Knox, R.W.O., Corfield, R.M., and Dunay, R.E. (eds.), Correlation of the Early Paleogene in Northwest Europe . London: Geological Society, Special Publication, vol. 101, pp. 353–380.
Google Scholar
Aubry, M.-P., Lucas, S., and Berggren, W.A. (eds.), 1998. Late Paleocene-early Eocene Biotic and Climatic Events in the Marine and Terrestrial Records . New York: Columbia University Press.
Bains, S., Corfield, R.M., and Norris, R.D., 1999. Mechanisms of climate warming at the end of the Paleocene. Science , 285 , 724–727.
Beerling, D., 2000. Increased terrestrial carbon storage across the Palaeocene-Eocene boundary. Palaeogeogr. Palaeoclimatol. Palaeoecol. , 161 , 395–405.
Berner, R.A., 1994. 3GEOCARB II: A revised model of atmospheric CO 2 over Phanerozoic time. Am. J. Sci. , 294 , 56–91.
Bice, K.L., and Marotzke, J., 2001. Numerical evidence against reversed thermohaline circulation in the warm Paleocene/Eocene ocean. J. Geophys. Res. , 106 , 11529–11542.
Bice, K.L., and Marotzke, J., 2002. Could changing ocean circulation have destabilized methane hydrate at the Paleocene/Eocene boundary? Paleoceanography , 17 (2), 1018, doi: 10.1029/2001PA000678.
Bralower, T.J., Thomas, D.J., Zachos, J.C., Hirschmann, M.M., Röhl, U., Sigurdsson, H., Thomas, E., and Whitney, D.L., 1997. High-resolution records of the late Paleocene thermal maximum and circum-Caribbean volcanism: Is there a link? Geology , 25 , 963–966.
Corfield, R.M., and Norris, R.D., 1998. The oxygen and carbon isotope context of the Paleocene/Eocene epoch boundary. In Aubry, M.-P., Lucas, S., and Berggren, W.A. (eds.), Late Paleocene-early Eocene Biotic and Climatic Events in the Marine and Terrestrial Records , New York: Columbia University Press.
Dickens, G.R., 2001. Carbon addition and removal during the late Paleocene thermal maximum: Basic theory with a preliminary treatment of the isotope record at Ocean Drilling Program Site 1051, Blake Nose. In Norris, R.D., Kroon, D., and Klaus, A. (eds.), Western North Atlantic Paleogene and Cretaceous Paleoceanography . London: Geological Society, pp. 293–306.
Dickens, G.R., O’Neil, J.R., Rea, D.K., and Owen, R.M., 1995. Dissociation of oceanic methane hydrate as a cause of the carbon isotope excursion at the end of the Paleocene. Paleoceanography , 10 , 965–971.
Hesselbo, S.P., Grocke, D.R., Jenkyns, H.C., Bjerrum, C.J., Farrimond, P., Morgans-Bell, H.S., and Green, O.R., Massive dissociation of gas hydrate during a Jurassic oceanic anoxic event. Nature , 406 , 392–395.
Huber, M., and Sloan, L.C., 2001. Heat transport, deep waters, and thermal gradients: Coupled simulation of an Eocene greenhouse climate. Geophys. Res. Lett. , 28 , 3481–3484.
Hudson, T.L., and Magoon, L.B., 2002. Tectonic controls on greenhouse gas flux to the Paleogene atmosphere from the Gulf of Alaska accretionary prism. Geology , 30 , 547–550.
Jahren, A.H., Arens, N.C., Sarmiento, G., Guerrero, J., and Amundson, R., 2001. Terrestrial record of methane hydrate dissociation in the Early Cretaceous. Geology , 29 , 159–162.
Katz, M.E., Pak, D.K., Dickens, G.R., and Miller, K.G., 1999. The source and fate of massive carbon input during the latest Paleocene thermal maximum, Science. 286 , 1531–1533.
Kennett, J., and Stott, L.D., 1991. Abrupt deep sea warming, paleoceanographic changes and benthic extinctions at the end of the Paleocene. Nature , 353 , 319–322.
Kent, D.V., Cramer, B.S., Lanci, L., Wang, D., Wright, J.D., and der Voo, R.V., 2003. A case for a comet impact trigger for the Paleocene/Eocene thermal maximum and carbon isotope excursion. Earth Planet. Sci. Lett. , 211 , 13–26.
Koch, P.L., Zachos, J.C., and Gingerich, P., 1992. Correlation between isotope records in marine and continental carbon reservoirs near the Palaeocene/Eocene boundary. Nature , 358 , 319–322.
Pearson, P., and Palmer, N., 2001. Atmospheric carbon dioxide concentrations over the past 60 million years. Nature , 406 , 695–699.
Rea, D.K., 1994. The paleoclimatic record provided by eoilian deposition in the deep sea: The geologic history of wind. Rev. Geophys. , 32 , 159–195.
Robert, C., and Kennett, J.P., 1994. Antarctic subtropical humid episode at the Paleocene-Eocene boundary: Clay mineral evidence. Geology , 22 , 211–214.
Röhl, U., Bralower, T.J., Norris, R.D., and Wefer, G., 2000. New chronology for the late Paleocene thermal maximum and its environmental implications. Geology , 28 , 927–930.
Royer, D.L., Wing, S.L., Beerling, D.J., Jolley, D.W., Koch, P.L., Hickley, L.J., and Berner, R.A., 2001. Paleobotanical evidence for near present day levels of atmospheric CO 2 during part of the tertiary. Science , 292 , 2310–2313.
Schmidt, G.A., and Shindell, D.T., 2003. Atmospheric composition, radiative forcing and climate change as a consequence of a massive methane release from gas hydrates. Paleoceanography , 18 , 1004, doi: 10.1029/2002PA000757.
Sinha, A., and Stott, L.D., 1994. New atmospheric pCO 2 estimates from palesols during the late Paleocene/early Eocene global warming interval. Global Planet. Change. 9 , 297–307.
Sloan, L.C., and Pollard, D., 1998. Polar stratospheric clouds: A high latitude warming mechanism in an ancient greenhouse world. Geophys. Res. Lett. , 25 , 3517–3520.
Sloan, L.C., and Rea, D.K., 1995. Atmospheric carbon dioxide and early Eocene climate: A general circulation modeling sensitivity study. Palaeogeogr. Palaeoclimatol. Palaeoecol. , 119 , 275–292.
Sloan, L.C., Walker, J.C.G., Moore, T.C., Rea, D.K., and Zachos, J.C., 1992. Possible methane induced warming in the early Eocene. Nature , 357 , 320–322.
Sloan, L.C., Huber, M., and Ewing, A., 1999. Polar straospheric cloud forcing in a greenhouse world: A climate modeling sensitivity study. In Abrantes F., and Mix A. (eds.), Reconstructing Ocean History . New York: Kluwer, pp. 273–293.
Thomas, E., and Shackleton, N.J., 1996. The Paleocene-Eocene benthic foraminiferal extinction and stable isotope anomalies. In Knox, R.W.O., Corfield, R.M., and Dunay, R.E. (eds.), Correlation of the Early Paleogene in Northwest Europe . London: Geological Society, Special Publication, vol. 101, pp. 401–441.
Zachos, J.C., Pagani, M., Sloan, L., Thomas, E., and Billups, K., 2000. Trends, rhythms, and aberrations in global climate 65 Ma to present. Science , 292 , 686–693.
Zachos, J.C., Thomas, D., Bralower, T., and Thomas, E., 2001. New constraints on the timing and magnitude of the Paleocene-Eocene boundary carbon isotope excursion in marine environments. Eos. Trans. AGU Fall Meet. Suppl. , 82 , 767.
Download references
You can also search for this author in PubMed Google Scholar
Editor information
Editors and affiliations.
Goddard Institute for Space Studies, Columbia University, New York, USA
Vivien Gornitz
Rights and permissions
Reprints and permissions
Copyright information
© 2009 Springer-Verlag
About this entry
Cite this entry.
Schmidt, G.A. (2009). Paleocene-Eocene Thermal Maximum. In: Gornitz, V. (eds) Encyclopedia of Paleoclimatology and Ancient Environments. Encyclopedia of Earth Sciences Series. Springer, Dordrecht. https://doi.org/10.1007/978-1-4020-4411-3_168
Download citation
DOI : https://doi.org/10.1007/978-1-4020-4411-3_168
Publisher Name : Springer, Dordrecht
Print ISBN : 978-1-4020-4551-6
Online ISBN : 978-1-4020-4411-3
eBook Packages : Earth and Environmental Science Reference Module Physical and Materials Science Reference Module Earth and Environmental Sciences
Share this entry
Anyone you share the following link with will be able to read this content:
Sorry, a shareable link is not currently available for this article.
Provided by the Springer Nature SharedIt content-sharing initiative
- Publish with us
Policies and ethics
- Find a journal
- Track your research
- Structure of Metals
- Properties of Metals
- Material Selection
Thermal Stress
- Brittle Fracture
- Unit Systems
- Force and Motion
- Newton's Laws
- Energy, Work, and Power
- Continuity Equation
- Laminar/Turbulent Flow
- Bernoulli's Equation
- Natural Circulation
- Two-Phase Fluid Flow
- Centrifugal Pumps
- Terminology
- Radiant Heat Transfer
- Heat Exchangers
- Boiling Heat Transfer
- Heat Generation
Check out these structural calculators :   • Beam Analysis   • Bolted Joints   • Lug Analysis • Column Buckling
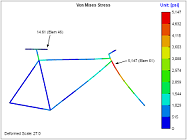
Thermal Shock
This page provides the chapters on thermal shock (thermal stress) from the "DOE Fundamentals Handbook: Material Science," DOE-HDBK-1017/1-93 , U.S. Department of Energy, Jan 1993.
Other related chapters from the "DOE Fundamentals Handbook: Material Science" can be seen to the right.
Thermal stresses arise in materials when they are heated or cooled. Thermal stresses effect the operation of facilities, both because of the large components subject to stress and because they are effected by the way in which the plant is operated. This chapter describes the concerns associated with thermal stress.
Thermal shock (stress) can lead to excessive thermal gradients on materials, which lead to excessive stresses. These stresses can be comprised of tensile stress , which is stress arising from forces acting in opposite directions tending to pull a material apart, and compressive stress , which is stress arising from forces acting in opposite directions tending to push a material together. These stresses, cyclic in nature, can lead to fatigue failure of the materials .
Thermal shock is caused by nonuniform heating or cooling of a uniform material, or uniform heating of nonuniform materials. Suppose a body is heated and constrained so that it cannot expand. When the temperature of the material increases, the increased activity of the molecules causes them to press against the constraining boundaries , thus setting up thermal stresses.
If the material is not constrained, it expands, and one or more of its dimensions increases. The thermal expansion coefficient ( α ) relates the fractional change in length ΔL/L , called thermal strain, to the change in temperature per degree ΔT .
Table 1 lists the coefficients of linear thermal expansion for several commonly-encountered materials.
In the simple case where two ends of a material are strictly constrained, the thermal stress can be calculated using Hooke's Law by equating values of ΔL/L from Equations (3-1), (3-2), and (3-3).
Example: Given a carbon steel bar constrained at both ends, what is the thermal stress when heated from 60°F to 540°F?
Thermal stresses are a major concern in reactor systems due to the magnitude of the stresses involved. With rapid heating (or cooling) of a thick-walled vessel such as the reactor pressure vessel, one part of the wall may try to expand (or contract) while the adjacent section, which has not yet been exposed to the temperature change, tries to restrain it. Thus, both sections are under stress. Figure 1 illustrates what takes place.
A vessel is considered to be thick-walled or thin-walled based on comparing the thickness of the vessel wall to the radius of the vessel. If the thickness of the vessel wall is less than about 1 percent of the vessel's radius, it is usually considered a thin-walled vessel . If the thickness of the vessel wall is more than 5 percent to 10 percent of the vessel's radius, it is considered a thick-walled vessel . Whether a vessel with wall thickness between 1 percent and 5 percent of radius is considered thin-walled or thick-walled depends on the exact design, construction, and application of the vessel.
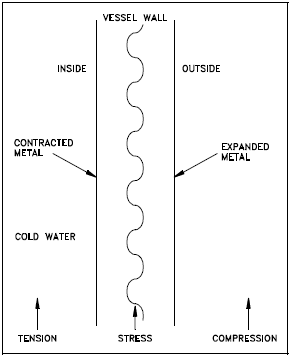
When cold water enters the vessel, the cold water causes the metal on the inside wall (left side of Figure 1) to cool before the metal on the outside. When the metal on the inside wall cools, it contracts, while the hot metal on the outside wall is still expanded. This sets up a thermal stress, placing the cold side in tensile stress and the hot side in compressive stress, which can cause cracks in the cold side of the wall . These stresses are illustrated in Figure 2 and Figure 3 in the next chapter.
The heatup and cooldown of the reactor vessel and the addition of makeup water to the reactor coolant system can cause significant temperature changes and thereby induce sizable thermal stresses. Slow controlled heating and cooling of the reactor system and controlled makeup water addition rates are necessary to minimize cyclic thermal stress, thus decreasing the potential for fatigue failure of reactor system components.
Operating procedures are designed to reduce both the magnitude and the frequency of these stresses. Operational limitations include heatup and cooldown rate limits for components, temperature limits for placing systems in operation, and specific temperatures for specific pressures for system operations. These limitations permit material structures to change temperature at a more even rate, minimizing thermal stresses.
We have a number of structural calculators to choose from. Here are just a few:
- Beam Calculator
- Bolted Joint Calculator
- Bolt Pattern Force Distribution
- Lug Calculator
- Column Buckling Calculator
- Fatigue Crack Growth Calculator
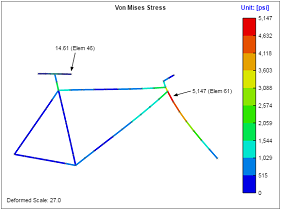
Pressurized Thermal Shock
Personnel need to be aware how pressure combined with thermal stress can cause failure of plant materials. This chapter addresses thermal shock (stress) with pressure excursions.
One safety issue that is a long-term problem brought on by the aging of nuclear facilities is pressurized thermal shock (PTS). PTS is the shock experienced by a thick-walled vessel due to the combined stresses from a rapid temperature and/or pressure change. Nonuniform temperature distribution and subsequent differential expansion and contraction are the causes of the stresses involved. As the facilities get older in terms of full power operating years, the neutron radiation causes a change in the ductility of the vessel material , making it more susceptible to embrittlement . Thus, if an older reactor vessel is cooled rapidly at high pressure, the potential for failure by cracking increases greatly.
Evaluating Effects of PTS
Changes from one steady-state temperature or pressure to another are of interest for evaluating the effects of PTS on the reactor vessel integrity. This is especially true with the changes involved in a rapid cooldown of the reactor system, which causes thermal shock to the reactor vessel. These changes are called transients. Pressure in the reactor system raises the severity of the thermal shock due to the addition of stress from pressure. Transients, which combine high system pressure and a severe thermal shock, are potentially more dangerous due to the added effect of the tensile stresses on the inside of the reactor vessel wall. In addition, the material toughness of the reactor vessel is reduced as the temperature rapidly decreases.
Stresses arising from coolant system pressure exerted against the inside vessel wall (where neutron fluence is greatest) are always tensile in nature. Stresses arising from temperature gradients across the vessel wall can either be tensile or compressive. The type of stress is a function of the wall thickness and reverses from heatup to cooldown. During system heatup, the vessel outer wall temperature lags the inner wall temperature. The stresses produced by this temperature gradient and by system pressure will produce the profile shown in Figure 2.
During heatup, it can be seen that while the pressure stresses are always tensile, at the 1/4 thickness (1/4 T), the temperature stresses are compressive. Thus, the stresses at the 1/4 T location tend to cancel during system heatup. At the 3/4 T location, however, the stresses from both temperature and pressure are tensile and thus, reinforce each other during system heatup. For this reason the 3/4 T location is limiting during system heatup.
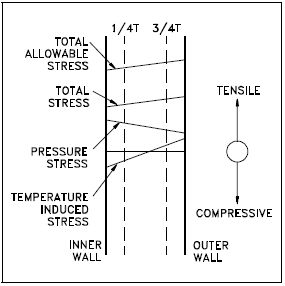
During system cooldown, the stress profile of Figure 3 is obtained. During cooldown, the outer wall lags the temperature drop of the inner wall and is at a higher temperature. It can be seen that during cooldown, the stresses at the 3/4 T location are tensile due to system pressure and compressive due to the temperature gradient. Thus during cooldown, the stresses at the 3/4 T location tend to cancel. At the 1/4 T location, however, the pressure and temperature stresses are both tensile and reinforce each other. Thus, the 1/4 T location is limiting during system cooldown.
Plant temperature transients that have the greatest potential for causing thermal shock include excessive plant heatup and cooldown, plant scrams, plant pressure excursions outside of normal pressure bands, and loss of coolant accidents (LOCAs). In pressurized water reactors (PWRs), the two transients that can cause the most severe thermal shock to the reactor pressure vessel are the LOCA with subsequent injection of emergency core cooling system (ECCS) water and a severe increase in the primary-tosecondary heat transfer.
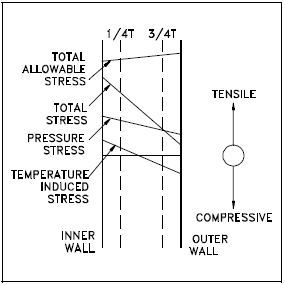
Locations of Primary Concern
Locations in the reactor system, in addition to the reactor pressure vessel, that are primary concerns for thermal shock include the pressurizer spray line and the purification system.

Thermal Cycling Study
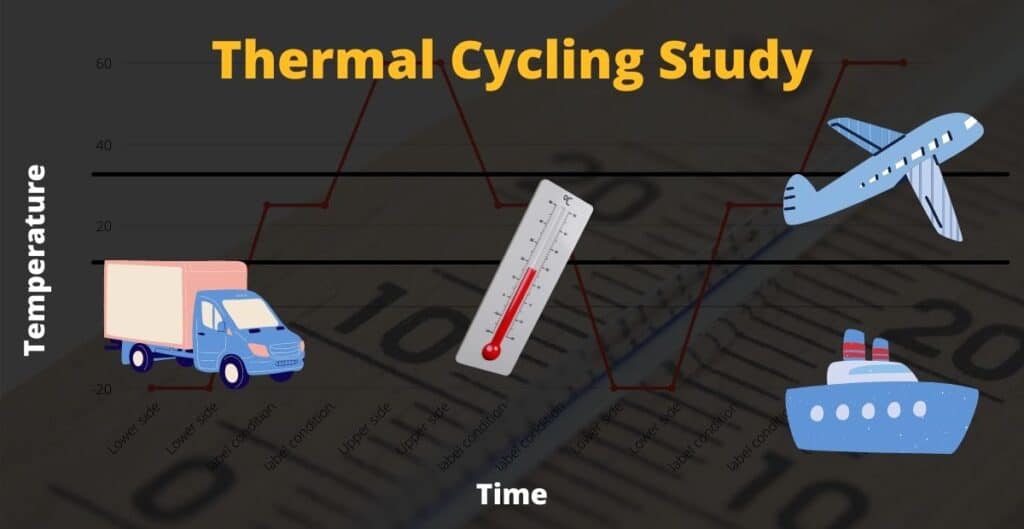
What is a thermal cycling study?
A thermal cycling study is done to determine the impact of temperature excursion on the edge and outside of a product’s labeled storage conditions. The excursion is simulated below and above the product’s labeled storage conditions for multiple cycles, and the impact on the product quality will be evaluated because of thermal cycling.
An example of thermal cycling is illustrated in the following diagrams.
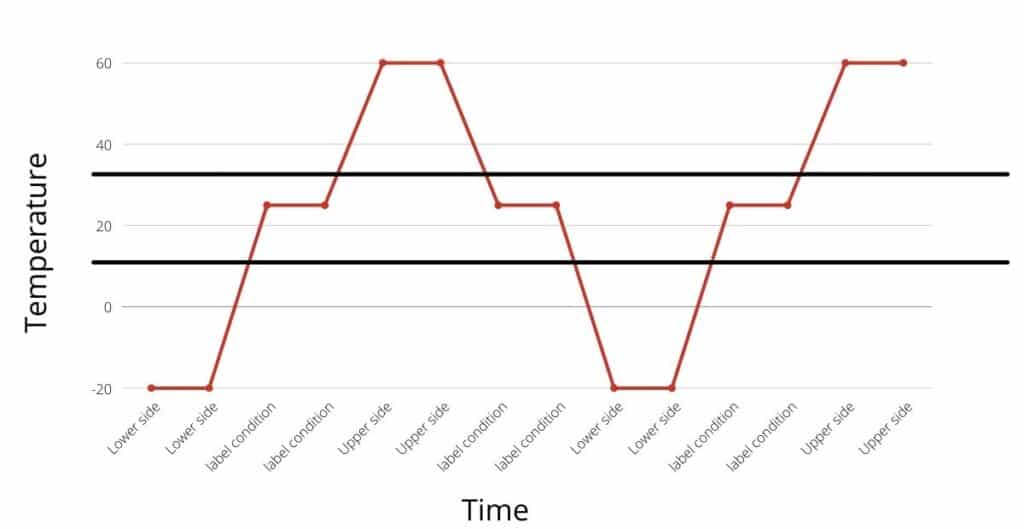
How is thermal cycling study different from excursion studies?
A thermal cycling study is done by simulating upper and lower side excursion multiple times and assessing its impact on product quality. Whereas, in the temperature excursion study, the one-sided excursion is simulated without cycling.
What are the reference guidelines?
There is no direct reference in any of the regulatory guidelines regarding testing to determine the suitability of transport conditions for sensitive products; however, you can find general instructions in various guidance.
ICH Q1A and WHO guidance provide general direction to perform stress testing. The study’s objective is to evaluate the impact of stress conditions (temperature, humidity, oxidation, pH, and light) on drug products.
ICH Q1A and WHO also suggest that the data from accelerated stability studies can be used to evaluate the effect of short-term excursions higher or lower than the label storage conditions that may occur during the shipping of drug products.
The guidelines also direct for the drug substance to be stored in a freezer: “…testing on a single batch at an elevated temperature (e.g., 5°C ± 3°C or 25°C ± 2°C) for an appropriate time period should be conducted to address the effect of short term excursions outside the proposed label storage condition, e.g., during shipping or handling.”
Similarly, for the drug products to be stored in a freezer, “In the absence of an accelerated storage condition for drug products intended to be stored in a freezer, testing on a single batch at an elevated temperature (e.g., 5°C ± 3°C or 25°C ± 2°C) for an appropriate time period should be conducted to address the effect of short term excursions outside the proposed label storage condition.”
ICH Q5C directs for the stability of Biotechnological/ Biological Products that “Studies under stress conditions may be useful in determining whether accidental exposures to conditions other than those proposed (e.g., during transportation) are deleterious to the product.”
Work had done by Bishara and Seevers has now been included in the Parenteral Drug Association (PDA) documents. In this document, temperature excursions and temperature cycling conditions are proposed.
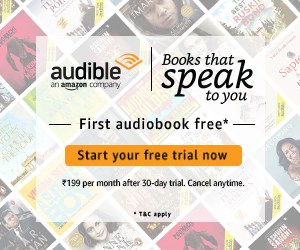
Why is thermal cycling study required?
Drug products are stored in the controlled environment in the facility, whereas when the products are transported in a commercial environment, it is a different scenario. Temperature conditions and excursions in the facility can be easily controlled; however, it may not be always possible during transportation. The impact on drug product due to unforeseen transport events could not be covered during the long-term and accelerated stability studies. Hence, the purpose of the thermal cycling study is to consider anticipated extreme challenges, including expected ambient temperature variation and shipment duration. In addition, the study will demonstrate the robustness of the product against usual excursions experienced during transportation.
How will it be helpful to justify excursions?
The drug product manufacturer’s responsibility is to ensure that the drug product reaches the patient without loss of therapeutic properties.
It is unpredictable that what types of excursion may occur during the transportation of pharmaceutical products, how long and how many times. For example, accidental temperature excursion may occur, which is beyond the pharmacopoeial acceptable excursion range. In such scenarios, the drug product would no longer be acceptable for patient consumption without sufficient scientific data.
Thermal cycling study results will help to determine product behavior and product quality impact when it experiences different environmental conditions during the transportation condition. In addition, the study data will help to understand the effect of accidental excursions on the drug product quality throughout the shelf life of the drug product.
How to design the thermal cycling study?
While designing the protocol for thermal cycling study, various factors should be considered such as product development data, Route of transportation, possible extreme conditions, and product strengths.
a. Support of product development data:
During the development of drug substances and drug products, R&D performs stress studies to determine the impact of various stress conditions on drug product, such as temperature, humidity, light, and oxygen. In this article, we will mainly discuss thermal stress. Suppose developmental studies show study product does not get degraded with extreme stress conditions. Those data may support accidental excursion during transportation, or these development data can support the design of thermal cycling study in terms of duration and extremes of stress condition. When development data shows the degradation of the product at specific stress conditions, there is no point in covering such situations during the study even though that could be expected excursion during transportation. In such a scenario, transportation facilities should be designed to maintain storage conditions so that such excursions would not occur.
b. Route of transportation:
The simplest way to design the study is to map the transportation routes from starting to recipient locations and identify the route’s seasonal temperature conditions and time required to reach from starting location to recipient locations. Typically two seasons are mapped, winter and summer. Consideration should be made for the day and night temperatures of the route. The external environmental temperature data can be collected using previous transportations or collected from historical meteorological data.
If one location is the Northern hemisphere and the other is the Southern hemisphere, the transportation route’s actual condition should be used. Such types of seasonal conditions are called summer-winter or winter-summer conditions. For example, a product is supplied to three different destinations. Temperature conditions should be mapped at the ambient environment to understand the potential excursions during transportation for respective destinations.
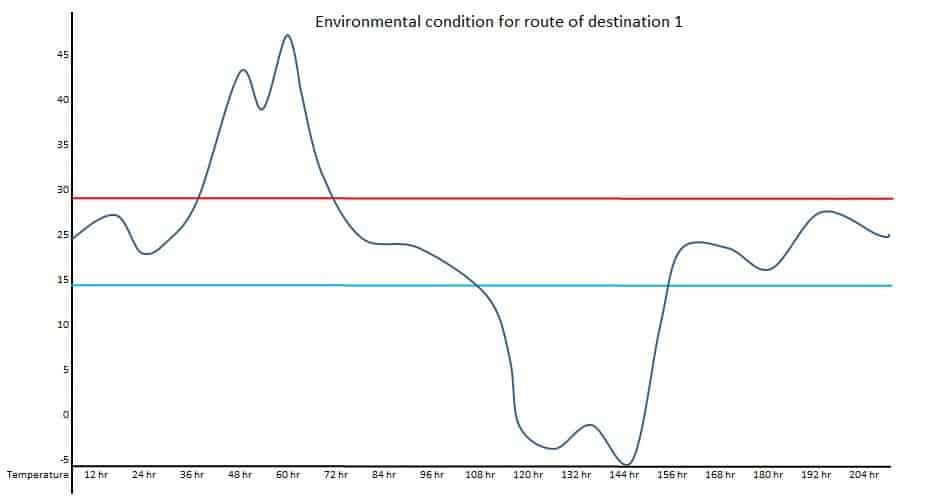
Data of above diagrams are summarized in following table.
Observed upper extreme temperature: 47 Deg. C
Observed lower extreme temperature: -5 Deg. C
Number of time upper side excursion during one run: 5 times
Number of time upper side excursion during one run: 2 times
Maximum total duration of upper side excursion: 55 hr
Maximum total duration of lower side excursion: 48 hr
Maximum duration of transportation: 228 hr
The above data can provide potential worst-case conditions during the transportation for the selected season. The study design for thermal cycling can be done based on the above data, such as total duration of the study, a number of excursion cycles, upper and lower extreme temperature, duration of each cycle, etc.
As per PDA Technical Report No. 39 (2007) suggest the following example for thermal cycling study for different storage conditions:
The above approach can be modified based on collected data from the field study data presented in Table: 1 for the storage condition of Controlled Room Temperature, 20 to 25°C. [Opinion of the author of this article]
c. Consideration of extremes :
There are natural temperature ranges that could be defined. It will be very unusual to have temperatures below −100°C or over 100°C in a natural environment. In Antarctica, temperature minima have been measured at −89°C. Temperature maxima have been measured at +58°C in Libya and in Death Valley. But even if a product is stored in a closed environment under the sun, it will not be heated over 100°C.
d. Consideration of product and strength selection :
The bracketing approach with higher and lower strength can be considered as one of the approaches. Product strengths to be bracketed can be defined using the Quality Risk Management principle.
Conclusion:
Systemically designed thermal cycling study will provide valuable insight about product robustness under stress condition and accidental exposure of product with high or low temperature can be scientifically justified. Based on the study performed, Product-Specific Transportation Control Strategy Document can be prepared for to determine the Effect of Temperature Excursions as follows:

Thermal excursion accelerating factors [GaAs reliability testing]
Ieee account.
- Change Username/Password
- Update Address
Purchase Details
- Payment Options
- Order History
- View Purchased Documents
Profile Information
- Communications Preferences
- Profession and Education
- Technical Interests
- US & Canada: +1 800 678 4333
- Worldwide: +1 732 981 0060
- Contact & Support
- About IEEE Xplore
- Accessibility
- Terms of Use
- Nondiscrimination Policy
- Privacy & Opting Out of Cookies
A not-for-profit organization, IEEE is the world's largest technical professional organization dedicated to advancing technology for the benefit of humanity. © Copyright 2024 IEEE - All rights reserved. Use of this web site signifies your agreement to the terms and conditions.
Thank you for visiting nature.com. You are using a browser version with limited support for CSS. To obtain the best experience, we recommend you use a more up to date browser (or turn off compatibility mode in Internet Explorer). In the meantime, to ensure continued support, we are displaying the site without styles and JavaScript.
- View all journals
- My Account Login
- Explore content
- About the journal
- Publish with us
- Sign up for alerts
- Open access
- Published: 24 September 2022
Astrochronology of the Paleocene-Eocene Thermal Maximum on the Atlantic Coastal Plain
- Mingsong Li ORCID: orcid.org/0000-0002-5542-8106 1 , 2 ,
- Timothy J. Bralower ORCID: orcid.org/0000-0002-3503-859X 2 ,
- Lee R. Kump ORCID: orcid.org/0000-0001-6009-8247 2 ,
- Jean M. Self-Trail 3 ,
- James C. Zachos 4 ,
- William D. Rush ORCID: orcid.org/0000-0002-8522-5684 4 , 5 , 6 &
- Marci M. Robinson ORCID: orcid.org/0000-0002-9200-4097 3
Nature Communications volume 13 , Article number: 5618 ( 2022 ) Cite this article
8946 Accesses
12 Citations
281 Altmetric
Metrics details
- Carbon cycle
- Palaeoceanography
- Palaeoclimate
- Sedimentology
The chronology of the Paleocene-Eocene Thermal Maximum (PETM, ~56 Ma) remains disputed, hampering complete understanding of the possible trigger mechanisms of this event. Here we present an astrochronology for the PETM carbon isotope excursion from Howards Tract, Maryland a paleoshelf environment, on the mid-Atlantic Coastal Plain. Statistical evaluation of variations in calcium content and magnetic susceptibility indicates astronomical forcing was involved and the PETM onset lasted about 6 kyr. The astrochronology and Earth system modeling suggest that the PETM onset occurred at an extreme in precession during a maximum in eccentricity, thus favoring high temperatures, indicating that astronomical forcing could have played a role in triggering the event. Ca content data on the paleo-shelf, along with other marine records, support the notion that a carbonate saturation overshoot followed global ocean acidification during the PETM.
Similar content being viewed by others
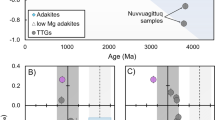
Calcium isotope evidence for early Archaean carbonates and subduction of oceanic crust
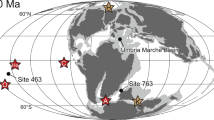
Mid-Cretaceous marine Os isotope evidence for heterogeneous cause of oceanic anoxic events
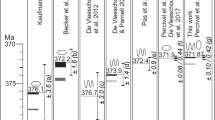
Anchoring the Late Devonian mass extinction in absolute time by integrating climatic controls and radio-isotopic dating
Introduction.
The Paleocene-Eocene Thermal Maximum (PETM) was an interval of global warming that occurred ca. 56 million years ago (Ma) and was characterized by a 4–5 °C global mean surface temperature increase 1 . Estimates of the total amount of carbon released during the PETM range from ~3000 Pg to more than 13,000 Pg 2 , 3 , 4 , which span the current assessments of remaining fossil fuel reserves 5 . The PETM is considered to have the highest carbon release rates for the past 66 million years 6 , although estimates of rate are still limited by the low fidelity of records. Proposed triggers for the PETM include volcanism associated with the North Atlantic Igneous Province 4 , 7 , dissociation of methane hydrates (e.g., ref. 8 ), variations in Earth’s orbit that controlled massive carbon release from permafrost melting or oceanic methane hydrates 9 , 10 , 11 , 12 , and an extraterrestrial impact 13 , 14 . To further complicate the matter, estimates for the onset of carbon isotope excursion (CIE) at the PETM range from several years 15 , 16 to thousands and even tens of thousands of years 10 , 12 , 17 , 18 , 19 , 20 in duration.
The PETM CIE onset is defined by a negative shift of δ 13 C. Over the past few decades, considerable effort has been made to reconstruct the chronology of the CIE using astrochronology 17 , 18 , 19 , 21 , 3 He isotope measurements 22 , 23 , and modeling experiments 6 , 24 . At one extreme, the CIE onset was estimated to have spanned only 13 years based on assumed annual “bedding” couplets at a paleo-shelf section on the mid-Atlantic Coastal Plain 15 , an assumption contradicted by evidence for coring artefacts produced via biscuiting whereby the formation is fractured during coring and drilling mud is injected in between layers. The 13-year duration is also contradicted by evidence from foraminifer accumulation rates 25 , 26 , and carbon cycle/climate modeling 6 , 27 . At the other extreme are estimates ranging up to 20 kyr as derived from deep sea cores 28 , 29 . These estimates, however, are complicated by slow sedimentation rates coupled with carbonate dissolution and bioturbation 12 , 19 , 30 . Independent astrochronologic studies for the basinal, shallow marine, and terrestrial sites with high sedimentation rates are few 18 , 21 , 31 , 32 and can be complicated due to the prevailing autogenetic sedimentation processes in stratigraphy 33 . Astrochronological age estimates from coastal/shelf records that have high sedimentation rates are still lacking, hindering the evaluation of the timing and the trigger of the PETM. In the coastal/shelf environment, non-orbital, 10 3 −10 5 year-scale sedimentary ‘noise’ resulting from storms, tides, bioturbation, variable sedimentation rates, short-term erosion, and diagenesis 34 , as well as mobile deltaic and continental shelf muds 35 , 36 can be strong, hampering a straightforward interpretation of the astronomical signal in cyclostratigraphy. Moreover, although astronomical cycles have long been recognized in the PETM interval 10 , 18 , 29 , 37 , statistical evaluation of the null hypothesis ( H 0 , no astronomical forcing) is rare, and links between astronomical forcing and proxy oscillations are unclear.
The Aquia Formation and Marlboro Clay from the Howards Tract cores (38.44827°N, 76.14159°W), two vertically offset holes in the Blackwater National Wildlife Refuge of Maryland (HT1 and HT2; Fig. 1 ), provide a unique opportunity to evaluate the PETM in a coastal/shelf environment using astrochronology. The Atlantic paleo-shelf sediments of the Marlboro Clay record the PETM in an exceptionally thick (5–15 m) deposit of the global low carbon value “core” of the PETM, which requires an order of magnitude faster sedimentation rate than deep-sea deposits, thus representing one of the most continuous paleo-shelf records from the mid-Atlantic Coastal Plain 38 . The spliced cores offer high temporal resolution paleoclimate proxies for the late Paleocene and early Eocene, e.g., calcium content and magnetic susceptibility (MS). Various studies demonstrate that Ca content and MS are two of the best recorders of astronomical cycles (refs. 39 , 40 and references within). Ca content has long been used as a proxy of carbonate productivity in response to astronomically forced climate change 41 . MS, a measurement of the concentration of magnetic minerals, is a proxy of detrital fluxes from terrestrial sources in the marine environment 42 .
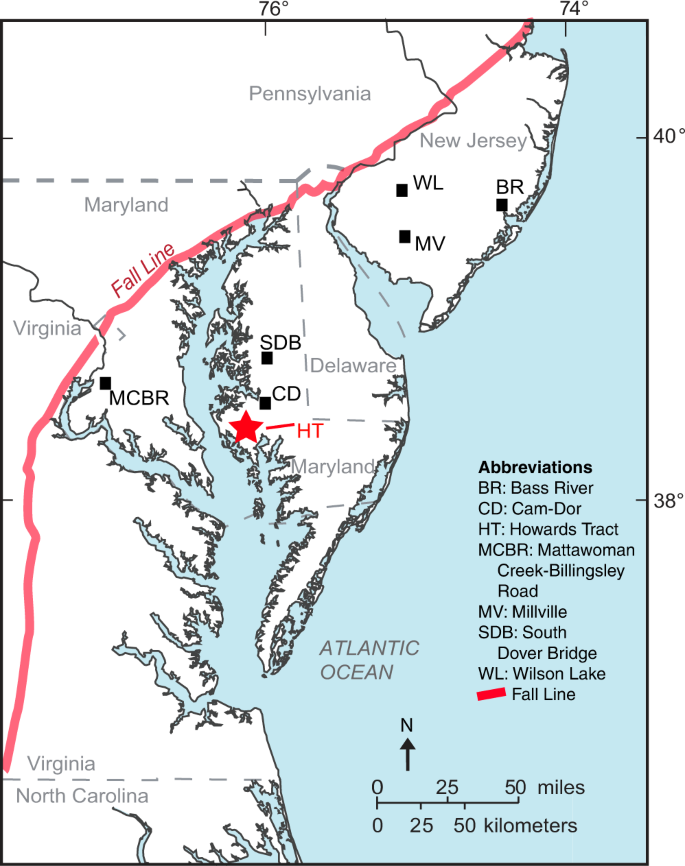
Fall line represents the paleo-shoreline of the Atlantic Ocean during the Paleocene-Eocene Thermal Maximum (PETM). BR Bass River; CD Cam-Dor; HT Howards Tract; MCBR Mattawoman Creek-Billingsley Road; MV Millville; SDB South Dover Bridge; WL Wilson Lake.
In this work, time series analysis of the proxy data (i.e., Ca content and MS) enables the recognition of astronomically forced sedimentary cycles at HT, leading to a high-resolution astrochronology for the PETM. The astrochronology is supported by statistical methods of sedimentation rate evaluation and an Earth system model of intermediate complexity. Earth system modeling of the effects of transient astronomical forcing using cGENIE provides a rare chance to elucidate the links between orbital forcing and paleoclimate proxies, e.g., Ca content, as well as the trigger to the PETM.
Results and discussion
Paleoclimate proxy records.
The studied interval includes from base to top the glauconite-rich quartz sands of the Aquia Formation, the sandy clay to clay of the Marlboro Clay, and the clayey sand of the Nanjemoy Formation. The contact between the Aquia Formation and the Marlboro Clay is gradational with decreasing coarse fraction and CaCO 3 content, and a gradual color change from dark greenish gray to brownish gray. In comparison, the highly burrowed interval between the Marlboro Clay and the Nanjemoy Formation indicates a disconformable contact. The high-resolution bulk carbonate δ 13 C record shows considerable variability at HT. Bulk carbonate δ 13 C records indicate the PETM CIE onset spans a 60-cm-thick interval (i.e., 200.47 to 199.89 m, pink bars in Figs. 2 – 3 ), which is defined by the initial sharp decline in the δ 13 C series and the changepoint analysis (see Methods and Supplementary Information). The magnitudes of the bulk carbonate δ 13 C and δ 18 O shifts at HT (Fig. 3a, b ) are far larger than those from most PETM sequences, an artefact of early diagenetic carbonate siderite, common in Marlboro Clay sediments 38 . In contrast, a lower resolution benthic isotope record shows δ 13 C and δ 18 O shifts with magnitudes consistent with other sections along the Atlantic margin (Fig. 2b, c ).
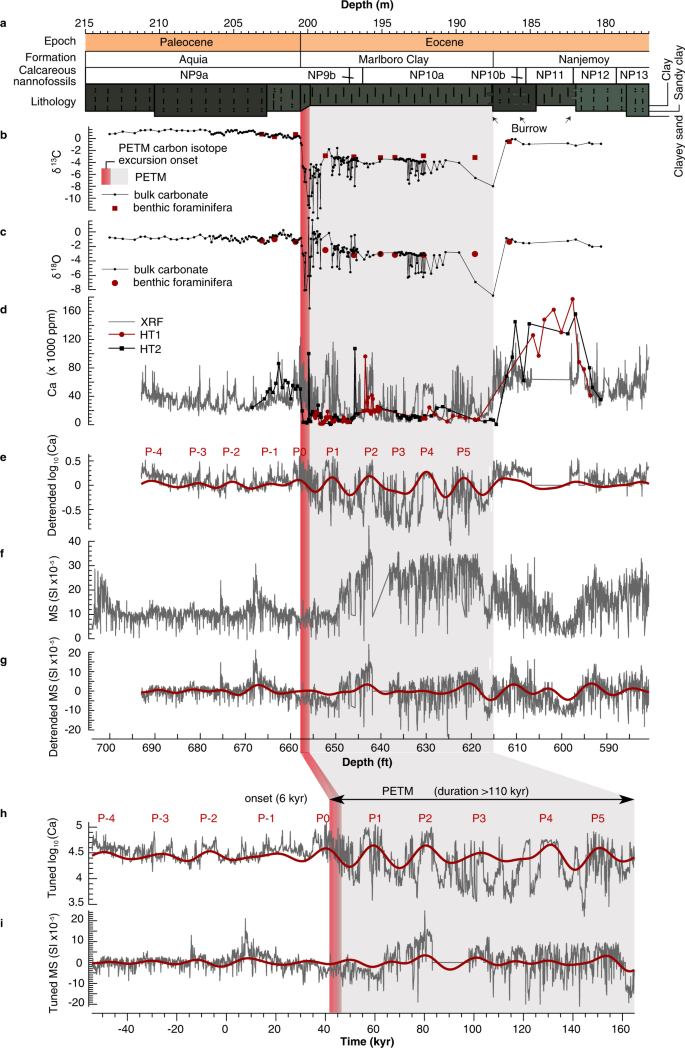
a Chronostratigraphy, lithostratigraphy, calcareous nannofossil zones, and lithology for the Howards Tract (HT) cores. The schematic core log shows the grain size and sediment color of the cores. b δ 13 C of the HT cores. c δ 18 O of the HT cores. d Calcium content generated from X-ray fluorescence (XRF) scans of the HT cores correlates well with carbonate content from both Howards Tract 1 (HT1) and Howards Tract 2 (HT2). Ca data from 182–185 m is missing due to a coring gap. e Filtered 2.8 m cycles indicative of precession (P) cycles (red, Gaussian filter with a frequency of 0.35 ± 0.15 m −1 ) of the detrended log 10 (Ca) content (gray). f Magnetic susceptibility (MS) of the HT cores. g Filtered 2.8 m cycles indicative of precession cycles (red, Gaussian filter with a frequency of 0.35 ± 0.15 m −1 ) of the detrended magnetic susceptibility. h , i Tuned log 10 (Ca) ( h ) and MS ( i ) and filtered precession (P) cycles (red, Gaussian filter with a frequency of 0.05 ± 0.018 kyr −1 ). Pink bar: Paleocene-Eocene Thermal Maximum (PETM) carbon isotope excursion (CIE) onset. Shaded region: PETM.
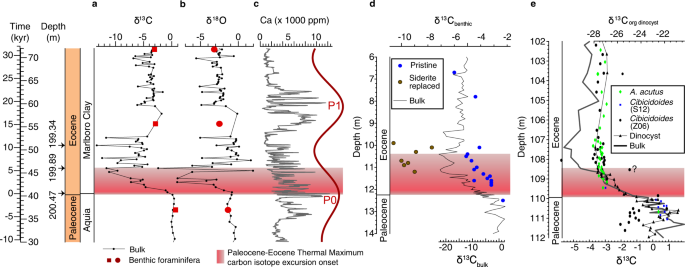
a Tuned δ 13 C values of the Howards Tract (HT) cores. b Tuned δ 18 O values of the HT cores. c Tuned calcium content generated from X-ray fluorescence (XRF) scans (gray line) and filtered precession (P) cycles (red line, detailed in Fig. 2h ) of the HT cores. d δ 13 C values of the Mattawoman Creek-Billingsley Road (MCBR) cores in stratigraphic depth 20 . e δ 13 C values of the Wilson Lake core in stratigraphic depth. The data source for Wilson Lake: Cibicidoides spp. and bulk carbonate (Z06 denotes ref. 89 ); Anomalinoides acutus and Cibicidoides spp. (S12 denotes ref. 53 ); organic matter of dinocysts 90 .
We measured Ca content using a Geotek X-ray fluorescence (XRF) scanner and magnetic susceptibility (MS) at 5 mm resolution. The XRF-generated Ca values generally match those measured in the lab (Fig. 2d ), confirming the reliability of the Ca content from XRF scanning. The XRF-generated Ca content in the Aquia Formation is low (median 3.3%) with lower amplitude oscillations. The Marlboro Clay interval has a very low Ca content (median 1.9%) with occasionally more elevated values. The Ca content increases abruptly in the basal Nanjemoy Formation (median 6.3%) and remains high throughout the section (median 9.9%). MS values are relatively low in the Aquia Formation and basal Marlboro Clay and then reach their highest values in the middle Marlboro Clay, before gradually decreasing in the Nanjemoy Formation. The exceptionally low values at 182 m coincide with a minor unconformity; values rise again in the upper Nanjemoy sediments (Fig. 2f ).
Cyclostratigraphic results
Time series analysis of the proxy data below the unconformity at the base of the Nanjemoy Formation shows astronomical cycle-paced variations of the detrended log 10 (Ca) and MS series (Fig. 4 and Supplementary Figs. 1 – 6 ). The Lomb-Scargle spectra of log 10 (Ca) and MS show dominant wavelengths of ~12 m and 2.2–3.4 m, respectively. There are also two higher-frequency cycles at 1.0–1.2 m and 0.67–0.73 m wavelength (Fig. 4a, f ). The statistical tuning of correlation coefficient (COCO) method 12 shows that optimal mean sedimentation rates are 8–15 cm/kyr, and the significance level of the null hypothesis of no orbital forcing is less than 0.05 (Fig. 4 ). Moreover, the average spectral misfit (ASM) method 43 , which objectively evaluates potential sedimentation rates, indicates the most likely mean sediment accumulation rate is 10–16 cm/kyr (Fig. 4f, g ), at which the significance level of the null hypothesis of no orbital forcing is as low as 0.0014 (Ca) and 0.0012 (MS). In other words, confidence levels of astronomically forced variations in Ca and MS are higher than 98.6%. Therefore, the ~12 m, 2.2–3.4 m, 1.0 m, and 0.63–0.75 m cycles represent ~100 kyr short eccentricity, ~20 kyr precession, and sub-Milankovitch cycles (~10 kyr and ~7 kyr), respectively.
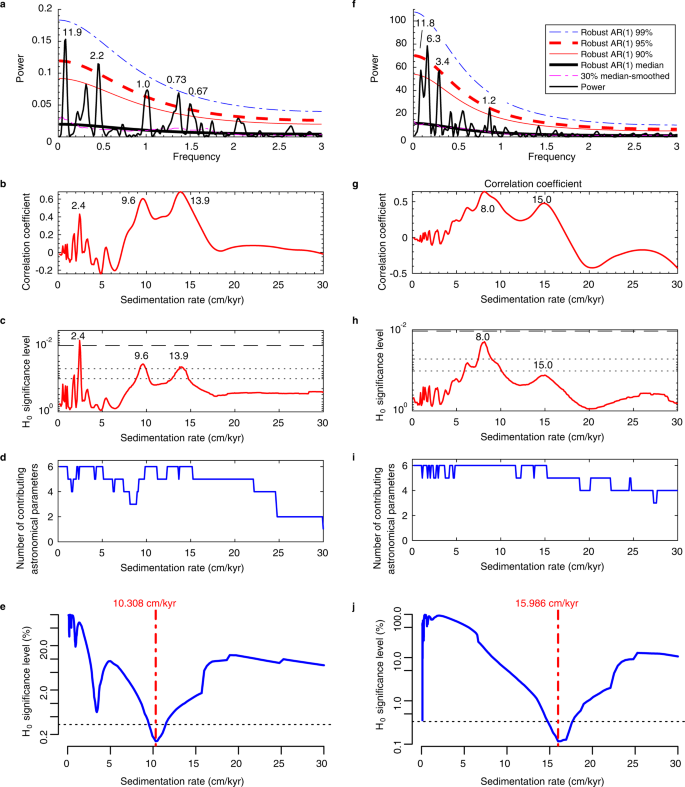
a , f Lomb-Scargle spectra of the detrended log 10 (Ca) ( a ) and detrended MS ( f ) series shown with confidence levels test against robust first-order autoregressive AR(1) red noise models. Significant periodicities are labeled with the unit of meters. The 1-slice correlation coefficient (COCO) spectra of the detrended log 10 (Ca) ( b – d ) and detrended MS ( g – i ) series. Correlation coefficient ( b, g ), null hypothesis (H 0 ) significance level ( c, h ), and number of contributing astronomical parameters (See Supplementary Information for details) ( d, i ) are shown. e, j Average spectral misfit (ASM) of the detrended log 10 (Ca) ( e ) and detrended MS ( j ) indicate roughly consistent mean sediment accumulation rate of 10–16 cm/kyr.
The evolutionary fast Fourier transform (FFT), wavelet transform and Spectral Moments 44 of both Ca and MS reveals similar first-order trends in sedimentation (SI): the dominant ~2 m precession cycle at ~205–200 m increases upward gradually to an ~3 m cycle at ~185 m (Supplementary Figs. 7 and 8 ). This suggests the accumulation rate increased ~1.5 times from the pre-PETM to the recovery phase (Supplementary Fig. 9 ). Tuning of ~3 m cycles of the Ca and MS series to the 20 kyr precession cycles enables for the recognition of 5.5 precession cycles from the PETM CIE onset through the hiatus at the top of the Marlboro Clay (Supplementary Tab. 1 ), suggesting the Howards Tract cores preserved the lowermost 110 kyr of the PETM event (Fig. 2 ). Assuming the duration of each filtered precession-related cycle was 20 kyr, this astrochronology suggests the PETM CIE onset was approximately 6 kyr (Fig. 3 ).
Duration of the carbon isotope excursion onset
There are two sources of uncertainty with the 6 kyr estimate for the PETM onset duration, including the definition of the CIE onset at HT and the uniformity of sedimentation rates. The Marlboro Clay is thought to have been deposited rapidly on a fluvial-deltaic-dominated shelf 36 . This energetic shelf was considered as an analog of the mobile mud belt on the modern Amazon shelf 35 , 45 . The combination of abundant Fe from weathering, and a suboxic early diagenetic environment in which alkalinity built up during the remineralization of organic matter via microbial sulfate reduction (cf. ref. 46 ), led to the precipitation of abundant siderite. The siderite formed in this early diagenetic setting incorporates low δ 13 C from the remineralized organic matter, particularly where the primary biogenic carbonate content is low 20 , 38 . This would be the case during the onset, which lies within a near carbonate-free layer. As siderite formation is driven by environmental changes associated with the PETM, the global carbon isotopic excursion of ~4-5 ‰ is amplified to ~13 ‰ at HT. Moreover, because the onset of the CIE at HT coincides closely with the base of the Marlboro Clay, the possibility exists that the timing of the isotope excursion reflects both the depositional and early diagenetic environment of the mobile mud belt as well as the input of isotopically light carbon that fueled the PETM warming recorded at sites globally.
To attempt to deconvolve these two factors, we compare the timing of the CIE onset at HT with other sections in Maryland and New Jersey where siderite is also present, yet the bulk carbonate δ 13 C still captures the global carbon isotope signal as represented in high resolution planktonic and benthic foraminifera records from the same sections. We compare the onset with the timing of the base of the Marlboro Clay as well as three nannoplankton datums to determine whether the initial stage of the CIE at HT was more abrupt than in the other sections; such an abrupt onset could be a result of a relationship with the deposition of the mobile mud belt or early diagenetic conditions within it (Fig. 5 ).
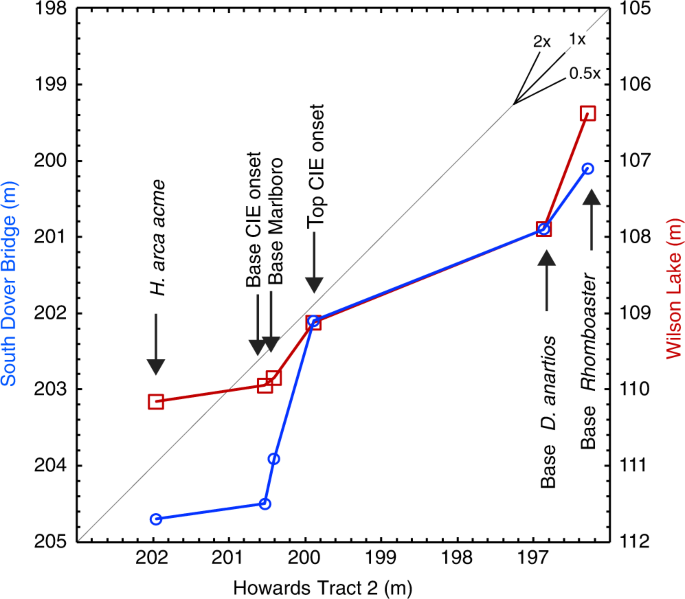
Blue: Howards Tract 2 versus South Dover Bridge (left); Red: Howards Tract 2 versus Wilson Lake (right). See Supplementary Information for details and discussion.
Identification of datums used in this analysis can be subjective, including the base of the Marlboro Clay 38 , change points in the carbon isotope excursion, and biostratigraphic datums, and we attempt to be as consistent as possible with the definition of all three types of datums (see Supplementary Information for more discussion). The analysis shows that the base of the onset of the CIE lies in an identical position to the base of the Marlboro Clay in HT as in the other two sections (Fig. 5 ). Moreover, the onset does not appear to be more abrupt at HT compared to Wilson Lake, New Jersey, as determined by its position relative to the three nannofossil datums, but it does appear to be two times more abrupt at HT than at South Dover Bridge, Maryland. The more abrupt onset at HT relative to the relatively close by South Dover Bridge section may be an artifact of a more condensed basal Marlboro Clay interval at HT; however, we cannot rule out the possibility that the presence of early diagenetic carbonate has made the CIE onset appear more abrupt than the original global signal. Indeed this looks to be the case at the Mattawoman Creek-Billingsley Road section in Maryland, where siderite is abundant, and the CIE onset in bulk carbonate δ 13 C is more abrupt than in benthic foraminifera 20 .
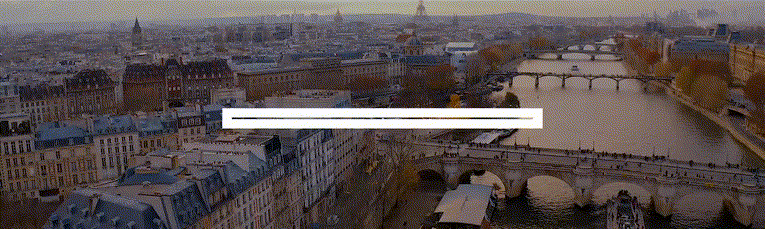
Sedimentation rate at Howards Tract
Our estimation for the PETM CIE onset duration assumes a constant sedimentation rate within the first precession cycle (P 0 in Figs. 2 – 3 ), a cycle that includes the transition between the Aquia Formation and the Marlboro Clay. The onset of the deposition of the mobile mud belt in the Marlboro Clay may have involved a significant increase in sedimentation rate that would weaken the constant sedimentation rate assumption, thus assuming a constant sedimentation rate would overestimate the duration of the CIE onset, which lies almost entirely in the Marlboro Clay. Nonetheless, spectral moments of both log 10 (Ca) and MS series indicate the mean sedimentation rate within each 4 m sliding window increases only slightly between the Aquia Formation and the Marlboro Clay (Supplementary Figs. 7 – 9 ); we consider the ca. 6 kyr duration of the CIE onset determined at HT given the uncertainty related to the definition of the CIE onset and the impact of diagenesis as discussed above.
High detrital accumulation rates are thought to enhance organic carbon sequestration during the PETM, which along with the silicate weathering feedback, drove the recovery of the Earth system from PETM CO 2 emissions as indicated in part by the termination of the CIE 47 , 48 . Indeed, many studies demonstrate that the hydrological cycle intensified during the PETM 49 , 50 , which is reflected in large part by the dramatic increase in sedimentation rates during the PETM in continental margin settings including Belluno Basin, Italy 31 , Tunisia 51 , Paleotethys 52 , Atlantic Coastal Plain sections 47 , 53 , west coast of North America 47 , Lomonosov Ridge in Arctic Ocean 54 , and North Sea Basin 32 . However, both the compilation of hydrologic records and Earth system modeling suggests the climate response had significant regional variability – some areas are characterized by increased precipitation-evaporation, whilst others are associated with a decrease 18 , 21 , 55 . Our high-resolution astrochronology indicates that the mean sedimentation rate during the PETM at HT was ~10 cm/kyr, which is consistent with the estimates from other sites on the Atlantic Coastal Plain 53 . The evolutionary FFT, wavelet, and Spectral Moments analyses indicate a generally smooth increase in the mean sedimentation rate in the HT cores (See SI), rather than a dramatic 2.8- to 220-fold increase (i.e., from 0.1–1.0 cm/kyr for the pre-CIE to 2.8–22 cm/kyr during the CIE) in regional sedimentation rates 53 . The previous sedimentation rates were estimated via the division of the stratigraphic thickness by the corresponding duration 53 , which was determined by stratigraphic correlation using biozones and the CIE shape, both of which are low resolution and can be affected by sporadic deposition and erosion in the Atlantic Coastal Plain. In comparison, the astrochronology as applied here and elsewhere is high resolution and relies on the net sediment accumulation rate.
Astrochronology of the Paleocene-Eocene thermal maximum
Our analysis of the Howards Tract cores is generally consistent with and more resolved than published astrochronologies and 3 He chronological models for the PETM. Cyclostratigraphy of deep-sea cores at ODP Sites 1051 (western North Atlantic) and 690 (Weddell Sea, Southern Ocean) suggested the PETM spanned 11 precession cycles yielding a duration of 210–220 kyr, and the PETM CIE onset of initial decrease in δ 13 C took over 20 kyr, while 52 kyr elapsed between the onset and the nadir of the δ 13 C excursion 28 , 29 . About two-thirds of the excursion occurred within two steps that each was less than 1 kyr in duration, assuming a constant sedimentation rate within each precession cycle 29 (but see ref. 56 ). The expanded hemipelagic Forada section (Italy) from the paleo-Tethys also records ~11 precession cycles (i.e., 231 ± 22 kyr) for the PETM 31 and the initial δ 13 C decline over 12.5 cm suggesting a ~5 kyr duration based on the approximately 50 cm precession cycle 57 . Reanalysis of sedimentary records at deep sea Site 690 and sites from ODP Leg 208 (southeastern Atlantic Ocean) showed the PETM duration was ~170 kyr 19 , which was supported by astrochronologic study of the Paleocene-Eocene boundary in Spitsbergen 17 , 58 . Cyclostratigraphy of the terrestrial Bighorn Basin site (Wyoming, USA) recognized ~7.5 precession cycles (~157 kyr) for the whole PETM 21 , but a subsequent study estimated the duration of the PETM in the Bighorn Basin to be ~200 kyr 18 . Both cyclostratigraphic studies in Bighorn Basin suggested that the PETM onset occurred in less than one precession cycle 18 , 21 . Similarly, the astrochronology of the shallow marine Zumaia section (Spain) indicates the PETM onset lasted less than 5 kyr 48 . In comparison, assuming a constant extraterrestrial 3 He flux, the independent 3 He age models for the PETM suggest the duration of the whole PETM is ~120 kyr 23 or 217 kyr (+44/−33 kyr) 22 . Nonetheless, deep sea cores 29 , the hemipelagic section in Italy 57 , and the shallow marine section in Spain 48 are condensed, hampering a credible estimation of the onset duration. Unlike all previous estimates based on the conventional cycle-ratio approach, which can be subjective and involve circular reasoning 59 , our study evaluates the null hypothesis of no orbital forcing and applies rigorous statistical tuning approaches to the chronology of the CIE onset. Here, our results suggest that the PETM record at Howards Tract spans no less than 110 kyr, though the main body of the event is truncated by an unconformity.
Our astrochronology from the same paleoshelf environment suggests that the PETM CIE onset is about 6 kyr in duration, challenging the “fast PETM onset” hypothesis 13 , 14 , 15 associated with the impact of a comet. Moreover, our estimate is generally consistent with those from Earth system modeling experiments that suggest the PETM CIE onset spanned at least 4 kyr 6 or less than 5 kyr 24 . The initial release of carbon at a rate of 0.6 Pg C/yr during the PETM, assuming an ~20 kyr duration of the onset, could be doubled when a 5 kyr duration is considered 4 , but anthropogenic carbon release rates at ~10 Pg C/yr 60 , which is one order of magnitude higher than that of the PETM. This study provides direct constraints on the carbon cycle and paleoclimate changes in the shelf environment, supporting the emerging consensus view of a few millennia for the onset interval.
Precession forced Ca oscillations
This study can improve our understanding of the linkage between orbital forcing and changes in paleoclimate proxies such as CaCO 3 content. Based on previous work using the cGENIE Earth-system model with transient orbital forcing (cf. ref. 61 ), we simulate the influence of transient astronomical forcing on paleoclimate to compare to our Howards Tract record. Modeling of the δ 13 C excursion using cGENIE has already been undertaken 2 , 4 , 48 , which forced the model to conform to observed isotope excursions, providing insightful constraints of the rate of carbon release and isotope fingerprint of the carbon source. Alternatively, we focus on astronomically forced climate change without simulating the effect of carbon release. In cGENIE model, variations of insolation are controlled by astronomical forcing 62 (Fig. 6a–c ). The upper envelope of mean daily insolation at HT was paced by 20 kyr precession cycles and modulated by eccentricity cycles (Fig. 6b, d ). The same is true for sea surface temperature (SST, Fig. 6e ) and [CO 3 2− ] ion concentration (Fig. 6f ). The upper envelope of mean daily CaCO 3 export fluxes of biological production (Fig. 6g ) or annual CaCO 3 fluxes (Fig. 6h ) at Howards Tract is dominated by precession cycles. The annual CaCO 3 export fluxes compare well with the filtered precession cycles of the Ca content in the Aquia Formation and the Marlboro Clay (Fig. 6i ). For example, the modeled CaCO 3 fluxes at ~110 kyr (i.e., 70 kyr after the PETM CIE onset) capture the minimal Ca content at 194 m (Fig. 6i ).
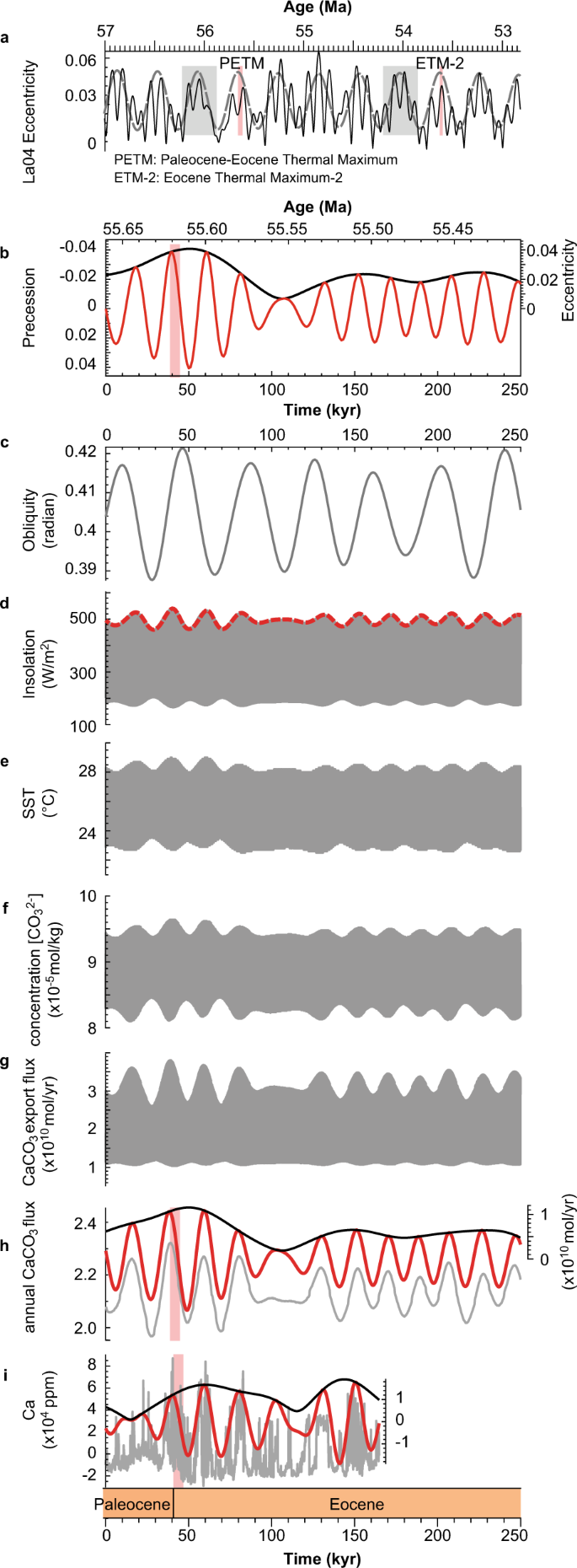
a Eccentricity of La2004 solution (black) 87 and filtered 405 kyr cycles (dashed gray) 12 . Gray bars in a denote long-term eccentricity minima following 12 . b Eccentricity (black) and precession (red) models of La2004 solution from 55.66 Ma to 55.41 Ma. c Obliquity model of La2004 solution from 55.66 Ma to 55.41 Ma. d Daily insolation (gray) at Howards Tract (HT) cores. Mean insolation on June 21 (dashed red) is also shown. The sampling rate is 1 kyr. e Daily sea surface temperature (SST) at HT cores. f Daily ocean surface [CO 3 2− ] concentration at HT cores. g Daily surface CaCO 3 export flux at HT cores. h Annual surface CaCO 3 export flux at HT cores (gray) shown with its Taner filtered output (red, cutoff frequency range: 0.032–0.068 kyr −1 ) and amplitude modulation (black) using Hilbert transform in Acycle. i Tuned Ca content (gray) derived from the HT cores shown with its filtered 20 kyr cycles (red, Taner filter with a cutoff frequency of 0.032–0.068 kyr −1 ) and amplitude modulation (black). Pink bars denote the Paleocene-Eocene Thermal Maximum carbon isotope excursion (CIE) onset (left bar in a , b , h , and i ) and Eocene Thermal Maximum 2 (right bar in a ). The frequency of daily time-series sampling is every 964 model time-steps, i.e., 20.083 year. Abbreviations: PETM, Paleocene-Eocene Thermal Maximum; ETM2, Eocene Thermal Maximum 2.
The above results can be explained by the fact that insolation forcing controls surface temperature and thus determines the rate of carbonate weathering 63 and silicate weathering 64 in cGENIE 65 , 66 and thus the alkalinity flux to the ocean. Subsequent variations in the ocean alkalinity drive changes in the [CO 3 2− ] ion concentration in the ocean, affecting the ocean calcite and aragonite saturation states and the preservation pattern of CaCO 3 in the sediments (cf. ref. 61 ). Here maxima of [CO 3 2− ] ion concentration are modulated by precession cycles and this modulation, along with variations of insolation and nutrients 67 , controls the CaCO 3 export flux (Fig. 6f, g ). Moreover, summer insolation is dominated by precession and eccentricity forcing (Supplementary Fig. 10 ), while the annual insolation intensity is controlled by obliquity forcing at HT (Supplementary Fig. 11 ). Therefore, CaCO 3 flux in HT cores could be paced by astronomically forced maximum insolation, in other words, the intensity of temperature in summer season or summer half-year. A positive swing in Ca time series at HT occurs when the northern summer occurs during perihelion, and vice versa. The amplitude of Ca concentration variations is higher at eccentricity maxima because of the modulation of eccentricity, during which Earth can be either particularly close to or away from the Sun in northern hemisphere summer on the 100−405 kyr time-scale (Fig. 6h, i ). Another scenario is that siliciclastic fluxes that control CaCO 3 content are modulated by astronomically forced changes in weathering, precipitation, runoff, and sediment discharge. In this scenario, siliciclastic dilution of CaCO 3 is driven partly by precipitation on the mid-Atlantic Coastal Plain which would impact sediment discharge and nutrient fluxes 68 . In addition, MS is considered to be a record of terrigenous material supplied to the depositional basin by runoff from the continent 42 , which suggests MS should be out of phase with the Ca concentration time series. Figure 2 shows more Ca generally corresponds to relatively less terrigenous material (thus drier summers), and vice versa. Therefore, the climate processes influencing the character of local sedimentation are not mutually exclusive and might enhance the lithologic cycle pattern.
Orbital trigger for the Paleocene-Eocene thermal maximum
Forcing the cGENIE model to conform to the boron isotope pH proxy and carbon isotope data indicates a mixed source of carbon release, i.e., volcanic outgassing plus methane hydrates and/or permafrost, during the PETM onset 4 , 48 . Both the astrochronology in the HT cores and model results demonstrate the PETM CIE onset occurred at an extreme in precession, favoring high temperature in summers (Fig. 6b, d, e ) and a maximum in the eccentricity cycles (Fig. 6a, b ), indicating an astronomical trigger. The possibility that volcanism pulsed at the maximum in the eccentricity cycles cannot be precluded; nonetheless, increased ocean temperatures could have triggered the release of methane hydrates and/or carbon ejection from permafrost (cf. refs. 9 , 11 ). This mechanism implies that hyperthermal warming events could have occurred at other times with similar orbital configurations. Time series analysis of the deep-sea records demonstrates both the PETM and Eocene Thermal Maximum 2 (ETM-2) occurred during the eccentricity maxima 10 that post-date the very long, Myr-scale eccentricity minima (Fig. 6a ) 11 , 12 . High-resolution paleoclimate proxy records (e.g., bulk carbonate and benthic δ 13 C and δ 18 O, Fe, and CaCO 3 ) reveal that the early Eocene global warmth was punctuated by recurrent, rapid hyperthermal events, which are mainly paced by cyclicities in Earth’s orbit eccentricity 69 , 70 , 71 , 72 . Moreover, coupled climate model simulations indicate that eccentricity-forced changes in ocean circulation and seawater temperature (through variations in seasonality) caused the destabilization of methane hydrates 73 , which could explain the increasing frequency and decreasing amplitude of hyperthermal warming events in the early Eocene. Therefore, the conjunction of 100 kyr, 405 kyr, and very long, Myr-scale eccentricity cycles may have facilitated the build-up of a major mobile reservoir of reduced carbon such as methane hydrates, marine dissolved organic carbon, and/or organic-rich peat before its release during the hyperthermal events 11 , 70 , 74 . Unlike those deep ocean records that are complicated by a major dissolution interval and bioturbation in the PETM interval 10 , 12 , 19 , 30 , high sedimentation rates and almost non-existent bioturbation in the HT cores allow for an unprecedented resolution for astrochronology of the PETM CIE onset that is supported by Earth system modeling, pointing to a possible orbital trigger for the PETM.
CaCO 3 preservation
The HT carbonate record exhibits signs of a carbonate saturation “overshoot” in the later recovery stage of the PETM. Theory, supported by recent observations, indicates that a large and rapid release of carbon into the Earth’s surface system induces a two-phase response in ocean carbonate saturation 75 . The first phase of carbon ejection will cause short-term ocean acidification lowering seawater ocean saturation (Ω), while the second phase could be characterized by carbonate oversaturation caused by elevated rates of silicate weathering and elevated carbonate deposition. This phenomenon, known as carbonate saturation overshoot, could have led to an over-deepening of the calcite compensation depth (CCD) relative to its pre-event depth 75 . Globally distributed PETM sites ranging from deep ocean to shelf support ocean acidification and the shoaling of the CCD possibly to even shallow shelf depths 38 . For example, multiple cores on the Atlantic paleo-shelf in Maryland and New Jersey record an interval devoid of carbonate during the onset of the PETM and the disappearance of nannofossils and planktic foraminifera 20 , 38 . The dissolution of calcareous material was considered to be syndepositional possibly due to the significant shoaling of the CCD, although there are other possible explanations involving local influences, including dilution coupled with euxinia 38 . In the second phase, the recovery and overshoot in carbonate saturation is best captured in hemi-pelagic and pelagic records 30 , 31 , 76 . The Forada section in particular, with a distinct clay layer indicates resumption of carbonate deposition roughly 20 kyrs after the acidification 31 . In the Atlantic, the CCD gradually deepened over several tens of thousand of years before a state of oversaturation was reached, resulting in carbonate deposition at depths previously below the CCD 75 , 77 . Collectively, these observations support carbon cycle models that include a silicate weathering feedback 3 , 75 , 77 . At HT, the Nanjemoy Formation preserves a high CaCO 3 content, i.e., up to 18% in the PETM late recovery phase versus 3.3% in the pre-PETM and 1.9% during the PETM body interval (Fig. 2 ), demonstrating the occurrence of the overshoot in carbonate saturation. This overshoot could explain the enhanced nannofossil preservation right above the dissolution interval in cores on the mid-Atlantic paleo-shelf 38 . The trend in the Atlantic paleoshelf demonstrates the carbon saturation overshoot impacted even the shallow ocean.
To review, a statistically significant astronomical signal in the Marlboro Clay has been detected, in this case from the Howards Tract cores in Maryland. The astrochronology suggests that the Marlboro Clay at this site preserves a 110-kyr record of the PETM and that the onset of the event lasted ~6 kyr. A combination of astrochronology and Earth system modeling suggests that the PETM CIE onset occurred at an extreme in precession favoring high temperature and at the maxima of 405 kyr and 100 kyr eccentricity cycles, indicating a possible orbital trigger. Astronomically paced siliciclastic and nutrient fluxes, along with precession-forced temperature-dependent changes in global weathering rates of carbonate and silicate rocks, could have contributed to oscillations of Ca content as exemplified at Howards Tract. Carbonate content data on the Atlantic paleo-shelf, along with other deep-sea records, suggest that carbonate saturation overshoot occurred not just in the deep sea but also in coastal regions during the PETM recovery.
Two cores were drilled at Howards Tract (HT1 and HT2, 5 m apart with offset coring intervals) to minimize loss due to coring gaps. Spliced data from HT1 and HT2 resulted in relatively complete coverage for the Aquia Formation, Marlboro Clay, and Nanjemoy Formation. The Aquia Formation-Marlboro Clay contact is located at 200.43 m (657.6 ft), and the Marlboro Clay-Nanjemoy Formation contact is at 187.5 m (615.2 ft). Observation of the HT cores suggests the contact between the Aquia Formation and the overlying Marlboro Clay is very gradational. The top Aquia Formation is greenish-black, laminated sandy clay, while the overlying Marlboro Clay is laminated and silty clay with a color change gradually from dark greenish gray to brownish gray. Therefore, this is no evidence of a disconformity at the base of the Marlboro Clay at HT 38 . In comparison, a transition between the Marlboro Clay and the underlying unit has been reported at other sites, including the Medford Auger Project (MAP) cores 36 , Millville 78 , CamDor, and Wilson Lake 38 on the mid-Atlantic Coastal Plain. Unlike cores at Millville and Wilson Lake 15 , Howards Tract cores show no evidence of couplets. Core photos are shown in Supplementary Figs. 12 – 15 .
Proxy data measurement
The elements in the Howards Tract cores (both HT1 and HT2) were measured using the XRF scanner of Geotek’s Multi-Sensor Core Logger at Pennsylvania State University. The measurement time for calcium is 30 seconds and the spatial resolution is 5 mm. To test the reliability of the XRF-scan calcium, we measured carbonate content on a UIC Inc. coulometrics Coulometer at the University of California Santa Cruz with a precision of ± 0.05%. δ 13 C and δ 18 O of bulk carbonate and benthic foraminifera (3-5 specimens from the 180-212 μm size fraction of Cibicidoides howelli prior to the CIE and Anomalinoides acutus following the CIE were analyzed on a Kiel/MAT253 at the University of California Santa Cruz. Analytical precision for δ 13 C and δ 18 O (i.e., ±0.1‰ and ±0.16‰, respectively; 2RSD) is based on the replicate analyses of standards (i.e., Carrara Marble). All data are reported relative to Vienna Pee Dee Belemnite. The sampling rate for bulk samples is ca. 0.1 m for the top of the Aquia Formation and increases to 0.03-0.05 m for the lower part of the Marlboro Clay. Nonetheless, the base of the Marlboro Clay is characterized by a prominent interval in which CaCO 3 content decreases to close to zero during the CIE onset, i.e., the low carbonate interval (LCI) on the New Jersey and Maryland paleoshelf 38 . This LCI can be further supported by a gap in the foraminifera and very poor coccolith shield preservation, due to a lack of calcareous material during the CIE onset at many mid-Atlantic paleoshelf sites, such as South Dover Bridge 79 , MCBR 20 , and HT 38 . The LCI and missing cores prevent a uniformly sampling strategy and are responsible for the sampling rate over 0.3 m in the bottom of the Marlboro Clay.
Changepoint analysis and the definition of the carbon isotope excursion onset
The changepoint analysis of δ 13 C data is able to provide the objective detection of changepoints at HT. Detailed search methods and test statistics of the changepoint analysis can be found in ref. 80 . We use the cpt.meanvar function of the changepoint R package 80 because the carbon isotope data show changes in both the mean and variance. Four changepoints are detected at depths of 186.61, 199.12, 200.47, and 202.92 m (Supplementary Text 1 and Supplementary Fig. 16 ). Among these, 200.47 m is used as the base of the CIE onset and coincides with the Aquia Formation-Marlboro Clay contact.
The changepoint analysis doesn’t provide direct constraints for the top of the CIE onset. We choose 199.89 m (5.8 kyr after the CIE onset) as the top of the onset because this position records the largest negative δ 13 C excursion, which is constrained by over one data point. The position at 199.34 m (11 kyr after the CIE onset) has the most negative δ 13 C value, however, this position is only constrained by one datapoint, which is thus not used in the main paper. Even if it is used as the top of the CIE onset, the comparison of the onset with the positions for the Marlboro Clay and the three nannoplankton datums shows the onset is no more abrupt at HT compared to Wilson Lake (Supplementary Fig. 17 ). The results do not contradict our conclusion on the sedimentation rate variation during the CIE onset.
Time series methods
The identification of astronomical cycles takes advantage of Acycle v2.4.1 software and follows typical procedures 58 . The Ca and magnetic susceptibility (MS) series carry a long-term trend that can be high amplitude and non-periodic, leading to power leakage from low-frequency components into the frequency band of interest 42 , therefore, both series were detrended after subtracting a 20-m “loess” (local regression using weighted linear least squares and a 2 nd degree polynomial model) trend for the MS series and a linear trend for the log 10 (Ca) series. Because regularly spaced time series is required for many powerful techniques in this study, the detrended log 10 (Ca) and MS series were interpolated using a “linear” method. To reveal the dominant wavelength of the proxy series and search for potential astronomical cycles, the Lomb-Scargle spectrum is calculated and shown with confidence levels test against robust AR(1) red noise models fitting to 30% median-smoothed power spectrum using the “Spectral Analysis” function in Acycle. Gauss and Taner bandpass filters were applied to isolate potential astronomical parameters 42 . Astronomical tuning is conducted using 20 kyr precession cycles and the “Age Scale” function in Acycle. In order to identify the sediment accumulation rate and test the null hypothesis that no astronomical forcing drove oscillations of the proxy series derived from the HT cores, we calculated the average spectral misfit (ASM) 43 using Astrochron package 81 and the correlation coefficient (COCO) spectra of the detrended log 10 (Ca) and detrended MS series and six astronomical target periodicities (i.e., 125, 95, 39.8, 23.3, 22.0, and 18.7 kyr), which is based on the power spectrum of astronomical target series (La2004 solution from 55 Ma to 57 Ma). Details of the parameters for the ASM calculation can be found in the Supplementary Information. In the COCO calculation, classic red noise models of both spectra were removed to suppress the very high amplitude for the low frequencies. Test sedimentation rates range from 0.13 cm/kyr to 30 cm/kyr with a step of 0.1 cm/kyr. The number of Monte Carlo simulations is 2000.
TimeOpt is a statistical method for the estimation of optimal sedimentation rate for a given paleoclimate proxy series 37 . At each test sedimentation rate, the proxy series was converted from depth domain to time domain. Then TimeOpt used the Taner filter and the Hilbert transform to isolate the potential precession cycles and the corresponding amplitude envelope. The envelope was linearly regressed on a synthetic time series that was generated using eccentricity frequencies retrieved from astronomical models (e.g., La2004 solution) for a given age. The correlation coefficient of the regression at the test sedimentation rate was recorded as r 2 envelope . Meanwhile, the data were linearly regressed to another synthetic dataset using frequencies of eccentricity and precession. And the regression was reported with the correlation coefficient r 2 power . The product r 2 opt = r 2 envelope * r 2 power was used to evaluate the most likely sedimentation rate. The test sedimentation rate with the highest r 2 opt might be the optimal sedimentation rate. The null hypothesis of no orbital forcing and the confidence of the optimal sedimentation rate can be evaluated using Monte Carlo simulations. The lag-1 correlation coefficient of the proxy series was calculated and used for the generation of many (e.g., 2000) red noise series. Then r 2 opt at each test sedimentation rate of these noise series were calculated and recorded. Consequently, the percentile of the r 2 opt using real proxy series indicates the chance of this r 2 opt that can occur randomly. The optimal sedimentation rate can be considered to be significant when the null hypothesis of r 2 opt is lower than 0.05. These calculations can be done either using astrochron package in R 81 or using Acycle software 58 .
Here, TimeOpt analysis for the detrended log 10 (Ca) series of the Aquia Formation and the Marlboro Clay using Acycle shows the most likely sedimentation rate is ca. 16.0 cm/kyr (Supplementary Fig. 3 ) at which the null hypothesis significance level of no orbital forcing is 0.001, that is, the confidence level of orbital forcing is 99.9% (Supplementary Fig. 4 ). The wide range of sedimentation rates at 10–17 cm/kyr demonstrates the sedimentation was variable at HT (Supplementary Fig. 4 ). This sedimentation rate is slightly higher than the COCO- and ASM-generated sedimentation of 8-16 cm/kyr (Fig. 4 ). In comparison, TimeOpt analysis for the MS series shows the most likely sedimentation rate is 8.1 cm/kyr (range of 6-10 cm/kyr; Supplementary Fig. 5 ) at which the null hypothesis significance level of no orbital forcing is 0.001 (Supplementary Fig. 6 ). This range is slightly lower than the COCO- and ASM-generated sedimentation rate of 8–16 cm/kyr. Nonetheless, all results point to the conclusion that variable 2–3 m wavelengths in our proxy records represent 20 kyr precession cycles. This is within expectation because multiple proxies and approaches can usually lead to different, but comparable within error, results 40 . Taken together, ASM, COCO and TimeOpt analyses indicate the PETM interval of the Marlboro Clay was paced by precession cycles, which were modulated by eccentricity cycles.
Variable sedimentation rate
In order to reveal the secular trend of dominant frequencies, the evolutionary fast Fourier transform (FFT) 42 were calculated with Acycle “Evolutionary Spectral Analysis” function 58 using a sliding window of 7 m and a step of 0.01 m. Because the window size is smaller than the reported eccentricity cycles (8–10 m), precession-related cycles (2–4 m) are the strongest signal in the evolutionary FFT result. The evolutionary FFT of both Ca and MS reveals a similar trend: the dominant ~2 m precession cycles at ~205–200 m increases upward smoothly to ~3 m cycles at ~185 m (Supplementary Fig. 7 ), indicating an increasing upward sedimentation rate from ca. 10 cm/kyr to ~15 cm/kyr. Wavelet analysis shows cyclicities of the data series are generally stable (Supplementary Fig. 8 ). The ca. 11 m wavelengths that are interpreted as 100 kyr short eccentricity cycles are mostly unchanged. The 2-3 m cycles (~20 kyr precession cycles) show a similar increase upward trend.
The Spectral Moments methods evaluate the first order change in sedimentation rate via investigating the analyzed series using a periodogram with two spectral moments: mean frequency (μf) and bandwidth (B) using a sliding window approach 44 . We detect shifts and changes in sedimentation rate using the Spectral Moments method in Acycle 2.4.1 58 . The edge of the data series is fulfilled using the zero-padding method. The bandwidth and mean frequency are calculated using a sliding window of 4 m with a running step of 0.1 m. The mean sedimentation rate, required by the Spectral Moments algorithm, is set to 10 cm/kyr based on the ASM and COCO analysis. We estimate the trend in sedimentation rate by taking the LOESS trend of the bandwidth.
Spectral moments of both series are shown in Supplementary Fig. 9 . The first-order changes in sedimentation rate using both Ca and MS series show an increasing upward trend. The minor discrepancies between the two estimated sedimentation rate maps indicate complex climate responses of different proxies 40 , and/or the ability of the spectral moments method in the estimation of fine-scale changes in sedimentation rate.
Earth system modeling
We used the cGENIE Earth system model to simulate the variability of the Ca content in the HT cores. The model consists of a 3D ocean circulation model 82 coupled to a 2D energy-moisture balance model (EMBM) of the atmosphere and a dynamic-thermodynamic sea-ice model 67 . It also includes a 3D module of marine biogeochemical cycling of major nutrients, trace elements, and isotopes in the ocean 83 , a 2D atmospheric chemistry module, and a module for interactions between sediments and ocean 84 and terrestrial weathering 66 .
The model used a Paleogene bathymetry and continental configuration and was initialized with a value of alkalinity (1975 umol.eq.kg −1 ) to produce a mean global CaCO 3 content of 47% 85 . Two-stage spin-up phases follow ref. 65 prior to the astronomical forcing experiment. In an initial spin-up phase, the ocean-atmosphere carbon cycle is set to ‘close’ with global weathering fluxes tracking sedimentary burial of CaCO 3 at all times and no bioturbation is allowed in the sediments 84 . This phase with a fixed p CO 2 value at 834 ppm and a prescribed δ 13 C value at −4.9% lasts 20 kyr and reaches steady state at the end of the simulation. In the second phase, we set the system to ‘open’ to allow for the temperature-controlled carbonate and silicate weathering and p CO 2 free to evolve. The bioturbation is allowed for the surface sediment layer 84 . This phase lasts 200 kyr with an acceleration ratio of 1:9 (10 yr model run in every 100 yr simulation). During this experiment, the p CO 2 drifts within 2 ppm over 200 kyr, similar to ref. 65 . In order to reproduce the astronomically forced Ca variations at HT core, the transient orbital forcing is enabled. Although some studies assign a ca. 56.0 Ma age for the PETM CIE onset 10 , 18 , we followed 12 , 37 , 86 , which indicates a ~55.6 Ma age for the PETM. Therefore, we used orbital parameters of 55.660-54.660 Ma in the La2004 solution to force cGENIE model 87 . The simulation results should not be largely affected by the choice of the onset age because both options occurred with similar orbital configurations, i.e., the peak of 405 kyr long eccentricity cycles 88 . The model was set to ‘open’ with bioturbation enabled and run for 300 kyr starting from 55.660 Ma, covering the entire PETM interval. The wall-clock time for one experiment is approximately 33 days.
Data availability
The proxy series of calcium content, magnetic susceptibility, and carbon and oxygen isotopes generated in this study are provided in Supplementary Data 1 .
Code availability
The Acycle 58 used in this study are available at https://doi.org/10.5281/zenodo.3955018 and can be obtained at: https://github.com/mingsongli/acycle . Astrochron 81 can be found at https://CRAN.R-project.org/package=astrochron . The cGENIE.muffin model used is tagged as release 0.9.4 and has been assigned a DOI ( https://doi.org/10.5281/zenodo.2654971 ). The code can be obtained at: https://github.com/derpycode/cgenie.muffin . Additional code and configuration files are provided in Supplementary Software 1 .
Dunkley Jones, T. et al. Climate model and proxy data constraints on ocean warming across the Paleocene–Eocene Thermal Maximum. Earth Sci. Rev. 125 , 123–145 (2013).
Article ADS CAS Google Scholar
Cui, Y. et al. Slow release of fossil carbon during the Palaeocene-Eocene Thermal Maximum. Nat. Geosci. 4 , 481–485 (2011).
Zeebe, R. E., Zachos, J. C. & Dickens, G. R. Carbon dioxide forcing alone insufficient to explain Palaeocene-Eocene Thermal Maximum warming. Nat. Geosci. 2 , 576–580 (2009).
Gutjahr, M. et al. Very large release of mostly volcanic carbon during the Palaeocene-Eocene Thermal Maximum. Nature 548 , 573 (2017).
Article ADS CAS PubMed PubMed Central Google Scholar
McGlade, C. & Ekins, P. The geographical distribution of fossil fuels unused when limiting global warming to 2 °C. Nature 517 , 187 (2015).
Article ADS CAS PubMed Google Scholar
Zeebe, R. E., Ridgwell, A. & Zachos, J. C. Anthropogenic carbon release rate unprecedented during the past 66 million years. Nat. Geosci. 9 , 325–329 (2016).
Svensen, H. et al. Release of methane from a volcanic basin as a mechanism for initial Eocene global warming. Nature 429 , 542–545 (2004).
Dickens, G. R., O’Neil, J. R., Rea, D. K. & Owen, R. M. Dissociation of oceanic methane hydrate as a cause of the carbon isotope excursion at the end of the Paleocene. Paleoceanography 10 , 965–971 (1995).
Article ADS Google Scholar
DeConto, R. M. et al. Past extreme warming events linked to massive carbon release from thawing permafrost. Nature 484 , 87–91 (2012).
Zeebe, R. E. & Lourens, L. J. Solar system chaos and the Paleocene–Eocene boundary age constrained by geology and astronomy. Science 365 , 926–929 (2019).
Article ADS MathSciNet CAS PubMed MATH Google Scholar
Lourens, L. J. et al. Astronomical pacing of late Palaeocene to early Eocene global warming events. Nature 435 , 1083–1087 (2005).
Li, M., Kump, L. R., Hinnov, L. A. & Mann, M. E. Tracking variable sedimentation rates and astronomical forcing in Phanerozoic paleoclimate proxy series with evolutionary correlation coefficients and hypothesis testing. Earth Planet. Sci. Lett. 501 , 165–179 (2018).
Schaller, M. F., Fung, M. K., Wright, J. D., Katz, M. E. & Kent, D. V. Impact ejecta at the Paleocene-Eocene boundary. Science 354 , 225–229 (2016).
Kent, D. V. et al. A case for a comet impact trigger for the Paleocene/Eocene thermal maximum and carbon isotope excursion. Earth Planet. Sci. Lett. 211 , 13–26 (2003).
Wright, J. D. & Schaller, M. F. Evidence for a rapid release of carbon at the Paleocene-Eocene thermal maximum. Proc. Natl Acad. Sci. U. S. A. 110 , 15908–15913 (2013).
Schaller, M. F & Fung, M. K. The extraterrestrial impact evidence at the Palaeocene–Eocene boundary and sequence of environmental change on the continental shelf. Philos. Trans. Royal Soc. A. 376 , 20170081 (2018).
Charles, A. J. et al. Constraints on the numerical age of the Paleocene-Eocene boundary. Geochem. Geophys . 12 , https://doi.org/10.1029/2010GC003426 (2011).
Westerhold, T. et al. Synchronizing early Eocene deep-sea and continental records – cyclostratigraphic age models for the Bighorn Basin Coring Project drill cores. Clim 14 , 303–319 (2018).
ADS Google Scholar
Röhl U., Westerhold T., Bralower, T. J. & Zachos, J. C. On the duration of the Paleocene‐Eocene thermal maximum (PETM). Geochem. Geophys . 8 , https://doi.org/10.1029/2007GC001784 (2007).
Self-Trail, J. M. et al. Shallow marine response to global climate change during the Paleocene-Eocene Thermal Maximum, Salisbury Embayment, USA. Paleoceanography , 32 , https://doi.org/10.1002/2017PA003096 (2017).
Aziz, H. A. et al. Astronomical climate control on paleosol stacking patterns in the upper Paleocene–lower Eocene Willwood Formation, Bighorn Basin, Wyoming. Geology 36 , 531–534 (2008).
Murphy, B. H., Farley, K. A. & Zachos, J. C. An extraterrestrial 3 He-based timescale for the Paleocene–Eocene thermal maximum (PETM) from Walvis Ridge, IODP Site 1266. Geochim. Cosmochim. Ac. 74 , 5098–5108 (2010).
Farley, K. & Eltgroth, S. An alternative age model for the Paleocene–Eocene thermal maximum using extraterrestrial 3 He. Earth Planet. Sci. Lett. 208 , 135–148 (2003).
Kirtland Turner, S., Hull, P. M., Kump, L. R. & Ridgwell, A. A probabilistic assessment of the rapidity of PETM onset. Nat. Commun. 8 , 353 (2017).
Article ADS PubMed PubMed Central Google Scholar
Pearson, P. N. & Nicholas, C. J. Layering in the Paleocene/Eocene boundary of the Millville core is drilling disturbance. Proc. Natl Acad. Sci. USA 111 , E1064–E1065 (2014).
Pearson, P. N. & Thomas, E. Drilling disturbance and constraints on the onset of the Paleocene-Eocene boundary carbon isotope excursion in New Jersey. Clim 11 , 95–104 (2015).
Zeebe, R. E., Dickens, G. R., Ridgwell, A., Sluijs, A. & Thomas, E. Onset of carbon isotope excursion at the Paleocene-Eocene thermal maximum took millennia, not 13 years. Proc. Natl Acad. Sci. USA. 111 , E1062–E1063 (2014).
Norris, R. D. & Rohl, U. Carbon cycling and chronology of climate warming during the Palaeocene/Eocene transition. Nature 401 , 775–778 (1999).
Röhl, U., Bralower, T., Norris, R. & Wefer, G. New chronology for the late Paleocene thermal maximum and its environmental implications. Geology 28 , 927–930 (2000).
Zachos, J. C. et al. Rapid acidification of the ocean during the Paleocene-Eocene thermal maximum. Science 308 , 1611–1615 (2005).
Giusberti, L. et al. Mode and tempo of the Paleocene-Eocene thermal maximum in an expanded section from the Venetian pre-Alps. Geol. Soc. Am. Bull. 119 , 391–412 (2007).
Jin, S. et al. Large-scale, astronomically paced sediment input to the North Sea Basin during the Paleocene Eocene Thermal Maximum. Earth Planet. Sci. Lett. 579 , 117340 (2022).
Article CAS Google Scholar
Hajek, E. A. & Straub, K. M. Autogenic sedimentation in clastic stratigraphy. Annu. Rev. Earth Pl. Sc. 45 , 681–709 (2017).
Li, M., Hinnov, L. A., Huang, C. & Ogg, J. G. Sedimentary noise and sea levels linked to land–ocean water exchange and obliquity forcing. Nat. Commun. 9 , 1004 (2018).
Kopp, R. E. et al. An Appalachian Amazon? Magnetofossil evidence for the development of a tropical river-like system in the mid-Atlantic United States during the Paleocene-Eocene thermal maximum. Paleoceanography 24 , https://doi.org/10.1029/2009PA001783 (2009).
Podrecca, L. G., Makarova, M., Miller, K. G., Browning, J. V. & Wright, J. D. Clear as mud: Clinoform progradation and expanded records of the Paleocene-Eocene Thermal Maximum. Geology 49 , 1441–1445 (2021).
Meyers, S. R. The evaluation of eccentricity-related amplitude modulation and bundling in paleoclimate data: An inverse approach for astrochronologic testing and time scale optimization. Paleoceanography , https://doi.org/10.1002/2015PA002850 (2015).
Bralower, T. J. et al. Evidence for Shelf Acidification during the Onset of the Paleocene-Eocene Thermal Maximum. Paleoceanogr. Paleoclimatol. 33 , 1408–1426 (2018).
Martinez, M., Aguado, R., Company, M., Sandoval, J. & O’Dogherty, L. Integrated astrochronology of the Barremian Stage (Early Cretaceous) and its biostratigraphic subdivisions. Glob. Planet. Change 195 , 103368 (2020).
Article Google Scholar
Li, M. et al. Paleoclimate proxies for cyclostratigraphy: Comparative analysis using a Lower Triassic marine section in South China. Earth-Sci. Rev. 189 , 125–146 (2019).
Herbert, T. D. & Fischer, A. G. Milankovitch climatic origin of mid-Cretaceous black shale rhythms in central Italy. Nature 321 , 739–743 (1986).
Kodama, K. P. & Hinnov, L. Rock Magnetic Cyclostratigraphy . Wiley-Blackwell (2015).
Meyers, S. R. & Sageman, B. B. Quantification of deep-time orbital forcing by average spectral misfit. Am. J. Sci. 307 , 773–792 (2007).
Sinnesael, M., Zivanovic, M., De Vleeschouwer, D. & Claeys, P. Spectral Moments in Cyclostratigraphy: Advantages and Disadvantages compared to more classic Approaches. Paleoceanogr. Paleoclimatol. 33 , 493–510 (2018).
Zhu, Z., Aller, R. C. & Mak, J. Stable carbon isotope cycling in mobile coastal muds of Amapá, Brazil. Continental Shelf Res. 22 , 2065–2079 (2002).
Aller, R. C., Hannides, A., Heilbrun, C. & Panzeca, C. Coupling of early diagenetic processes and sedimentary dynamics in tropical shelf environments: the Gulf of Papua deltaic complex. Cont. Shelf Res. 24 , 2455–2486 (2004).
John, C. M. et al. North American continental margin records of the Paleocene-Eocene thermal maximum: Implications for global carbon and hydrological cycling. Paleoceanography 23 , https://doi.org/10.1029/2007PA001465 (2008).
Dunkley Jones, T. et al. Dynamics of sediment flux to a bathyal continental margin section through the Paleocene–Eocene Thermal Maximum. Clim. 14 , 1035–1049 (2018).
Robert, C. & Kennett, J. P. Antarctic subtropical humid episode at the Paleocene-Eocene boundary: Clay-mineral evidence. Geology 22 , 211–214 (1994).
Pagani, M. et al. Arctic hydrology during global warming at the Palaeocene/Eocene thermal maximum. Nature 442 , 671–675 (2006).
Stassen, P., Dupuis, C., Steurbaut, E., Yans, J. & Speijer, R. P. Perturbation of a Tethyan coastal environment during the Paleocene–Eocene thermal maximum in Tunisia (Sidi Nasseur and Wadi Mezaz). Palaeogeogr. Palaeoclimatol. Palaeoecol. 317-318 , 66–92 (2012).
Egger, H., Homayoun, M., Huber, H., Rögl, F. & Schmitz, B. Early Eocene climatic, volcanic, and biotic events in the northwestern Tethyan Untersberg section, Austria. Palaeogeogr. Palaeoclimatol. Palaeoecol. 217 , 243–264 (2005).
Stassen, P., Thomas, E. & Speijer, R. P. Integrated stratigraphy of the Paleocene‐Eocene thermal maximum in the New Jersey Coastal Plain: Toward understanding the effects of global warming in a shelf environment. Paleoceanography 27 , PA4210 (2012).
Sluijs, A. et al. Arctic late Paleocene–early Eocene paleoenvironments with special emphasis on the Paleocene-Eocene thermal maximum (Lomonosov Ridge, Integrated Ocean Drilling Program Expedition 302). Paleoceanography 23 , https://doi.org/10.1029/2007PA001495 (2008).
Carmichael, M. J. et al. Hydrological and associated biogeochemical consequences of rapid global warming during the Paleocene-Eocene Thermal Maximum. Glob. Planet. Change 157 , 114–138 (2017).
Bralower, T. J. Evidence of surface water oligotrophy during the Paleocene-Eocene thermal maximum: Nannofossil assemblage data from Ocean Drilling Program Site 690, Maud Rise, Weddell Sea. Paleoceanography 17 , 13-11–13-12 (2002).
Google Scholar
Kirtland Turner S. Constraints on the onset duration of the Paleocene–Eocene Thermal Maximum. Philos. Trans. Royal Soc. A. 376 , https://doi.org/10.1098/rsta.2017.0082 (2018).
Li, M., Hinnov, L. & Kump, L. Acycle: time-series analysis software for paleoclimate research and education. Comput. Geosci. 127 , 12–22 (2019).
Puetz, S. J., Prokoph, A. & Borchardt, G. Evaluating alternatives to the Milankovitch theory. J. Stat. Plan. Infer. 170 , 158–165 (2016).
Article MathSciNet MATH Google Scholar
Le Quéré, C. et al. Trends in the sources and sinks of carbon dioxide. Nat. Geosci. 2 , 831–836 (2009).
Vervoort, P., Kirtland Turner, S., Rochholz, F. & Ridgwell, A. earth system model analysis of how astronomical forcing is imprinted onto the marine geological record: the role of the inorganic (carbonate) carbon cycle and feedbacks. Paleoceanogr. Paleoclimatol . 36 , https://doi.org/10.1029/2020PA004090 (2021).
Berner, R. A. & Kothavala, Z. GEOCARB III: a revised model of atmospheric CO 2 over Phanerozoic time. Am. J. Sci. 301 , 182–204 (2001).
Berner, R. A. GEOCARB III: a revised model of atmospheric CO 2 over Phanerozoic time. Am. J. Sci. 301 , 182–204 (2001).
Brady, P. V. The effect of silicate weathering on global temperature and atmospheric CO2. J. Geophys. Res. Solid Earth 96 , 18101–18106 (1991).
Greene, S. E. et al. Early Cenozoic decoupling of climate and carbonate compensation depth trends. Paleoceanogr. Paleoclimatol. 34 , 930–945 (2019).
Colbourn, G., Ridgwell, A. & Lenton, T. The rock geochemical model (RokGeM) v0. 9. Geosci. Model Dev. 6 , 1543–1573 (2013).
Ridgwell, A. et al. Marine geochemical data assimilation in an efficient Earth System Model of global biogeochemical cycling. Biogeosciences 4 , 87–104 (2007).
Rush, W. D., Kiehl, J. T., Shields, C. A. & Zachos, J. C. Increased frequency of extreme precipitation events in the North Atlantic during the PETM: Observations and theory. Palaeogeogr. Palaeoclimatol. Palaeoecol. 568 , 110289 (2021).
Kirtland Turner, S., Sexton, P. F., Charles, C. D. & Norris, R. D. Persistence of carbon release events through the peak of early Eocene global warmth. Nat. Geos. 7 , 748–751 (2014).
Zachos, J. C., McCarren, H., Murphy, B., Röhl, U. & Westerhold, T. Tempo and scale of late Paleocene and early Eocene carbon isotope cycles: Implications for the origin of hyperthermals. Earth Planet. Sci. Lett. 299 , 242–249 (2010).
Galeotti, S. et al. Orbital chronology of Early Eocene hyperthermals from the Contessa Road section, central Italy. Earth Planet. Sci. Lett. 290 , 192–200 (2010).
Lauretano, V., Zachos, J. C. & Lourens, L. J. Orbitally paced carbon and deep‐sea temperature changes at the peak of the early eocene climatic optimum. Paleoceanogr. Paleoclimatol. 34 , 1050–1065 (2018).
Lunt, D. J. et al. A model for orbital pacing of methane hydrate destabilization during the Palaeogene. Nat. Geosci. 4 , 775–778 (2011).
Sexton, P. F. et al. Eocene global warming events driven by ventilation of oceanic dissolved organic carbon. Nature 471 , 349 (2011).
Penman, D. E. et al. An abyssal carbonate compensation depth overshoot in the aftermath of the Palaeocene-Eocene Thermal Maximum. Nat. Geosci. 9 , 575–580 (2016).
Kelly, D. C., Nielsen, T. M. J., McCarren, H. K., Zachos, J. C. & Röhl, U. Spatiotemporal patterns of carbonate sedimentation in the South Atlantic: Implications for carbon cycling during the Paleocene–Eocene thermal maximum. Palaeogeogr. Palaeoclimatol. Palaeoecol. 293 , 30–40 (2010).
Dickens, G. R., Castillo, M. M. & Walker, J. C. G. A blast of gas in the latest Paleocene: Simulating first-order effects of massive dissociation of oceanic methane hydrate. Geology 25 , 259–262 (1997).
Makarova, M. et al. Hydrographic and ecologic implications of foraminiferal stable isotopic response across the U.S. mid-Atlantic continental shelf during the Paleocene-Eocene Thermal Maximum. Paleoceanography 32 , 56–73 (2017).
Self-Trail, J. M., Powars, D. S., Watkins, D. K. & Wandless, G. A. Calcareous nannofossil assemblage changes across the Paleocene–Eocene Thermal Maximum: Evidence from a shelf setting. Mar. Micropaleontol. 92 , 61–80 (2012).
Killick, R. & Eckley, I. changepoint: An R package for changepoint analysis. J. Stat. Softw. 58 , 1–19 (2014).
Meyers, S. R. astrochron: An R Package for Astrochronology. https://cran.r-project.org/package=astrochron (2014).
Edwards, N. R. & Marsh, R. Uncertainties due to transport-parameter sensitivity in an efficient 3-D ocean-climate model. Clim. Dyn. 24 , 415–433 (2005).
Ridgwell, A. Interpreting transient carbonate compensation depth changes by marine sediment core modeling. Paleoceanography 22 , https://doi.org/10.1029/2006PA001372 (2007).
Ridgwell, A. & Hargreaves, J. C. Regulation of atmospheric CO 2 by deep-sea sediments in an Earth system model. Global Biogeochem. Cy . 21 , https://doi.org/10.1029/2006GB002764 (2007).
Panchuk, K., Ridgwell, A. & Kump, L. R. Sedimentary response to Paleocene-Eocene thermal maximum carbon release: a model-data comparison. Geology 36 , 315–318 (2008).
Westerhold T. et al. On the duration of magnetochrons C24r and C25n and the timing of early Eocene global warming events: Implications from the Ocean Drilling Program Leg 208 Walvis Ridge depth transect. Paleoceanography 22 , https://doi.org/10.1029/2006PA001322 (2007).
Laskar, J. et al. A long-term numerical solution for the insolation quantities of the Earth. Astron. Astrophys. 428 , 261–285 (2004).
Westerhold, T., Röhl, U. & Laskar, J. Time scale controversy: accurate orbital calibration of the early Paleogene. Geochem. Geophys . 13 , https://doi.org/10.1029/2012gc004096 (2012).
Zachos, J. C. et al. Extreme warming of mid-latitude coastal ocean during the Paleocene-Eocene Thermal Maximum: Inferences from TEX86 and isotope data. Geology 34 , 737–740 (2006).
Sluijs, A. et al. Environmental precursors to rapid light carbon injection at the Palaeocene/Eocene boundary. Nature 450 , 1218–1221 (2007).
Download references
Acknowledgements
This project was funded by the National Key R&D Program of China (2021YFA0718200, M.L.) and the Heising-Simons Foundation, United States (2016–11, M.L. and L.R.K.). M.L. acknowledges the National Natural Science Foundation of China (42072040) and the Fundamental Research Funds for the Central Universities, Peking University (7100603368). T.J.B. acknowledges the National Science Foundation grant OCE-1416663. J.M.S. and M.M.R. acknowledge funding from the U.S. Geological Survey Climate Research and Development Program. The experiment was done on the Domino cluster at the University of California at Riverside. Andy Ridgwell, Sandra Kirtland Turner, and Pam Vervoort are acknowledged for their help in cGENIE modeling. We thank Debra Willard and Kristin McDougall-Reid for their review of an early draft of the manuscript. Any use of trade, firm, or product names is for descriptive purposes only and does not imply endorsement by the U.S. Government.
Author information
Authors and affiliations.
Key Laboratory of Orogenic Belts and Crustal Evolution, MOE, School of Earth and Space Sciences, Peking University, Beijing, 100871, China
Mingsong Li
Department of Geosciences, Pennsylvania State University, University Park, PA, 16802, USA
Mingsong Li, Timothy J. Bralower & Lee R. Kump
Florence Bascom Geoscience Center, U.S. Geological Survey, Reston, VA, 20192, USA
Jean M. Self-Trail & Marci M. Robinson
Department of Earth and Planetary Sciences, University of California Santa Cruz, Santa Cruz, CA, 95064, USA
James C. Zachos & William D. Rush
Department of Earth and Planetary Sciences, Yale University, New Haven, CT, 06511, USA
William D. Rush
Cooperative Institute for Research in Environmental Sciences (CIRES), University of Colorado Boulder, Boulder, CO, 80309, USA
You can also search for this author in PubMed Google Scholar
Contributions
M.L. and T.J.B. designed the study, T.J.B., J.M.S, and M.M.R collected and prepared cores, M.L. conducted XRF scanning, W.D.R. collected and identified the benthic foraminifera and carried out the isotope analyses, M.L., T.J.B., L.R.K, J.M.S., and J.C.Z interpreted data. M.L. wrote the paper, and all authors contributed to editing the paper.
Corresponding author
Correspondence to Mingsong Li .
Ethics declarations
Competing interests.
The authors declare no competing interests.
Peer review
Peer review information.
Nature Communications thanks Kenneth Kodama and Paul Pearson for their contribution to the peer review of this work. Peer reviewer reports are available.
Additional information
Publisher’s note Springer Nature remains neutral with regard to jurisdictional claims in published maps and institutional affiliations.
Supplementary information
Supplementary information, peer review file, description of additional supplementary files, supplementary data 1, supplementary software 1, rights and permissions.
Open Access This article is licensed under a Creative Commons Attribution 4.0 International License, which permits use, sharing, adaptation, distribution and reproduction in any medium or format, as long as you give appropriate credit to the original author(s) and the source, provide a link to the Creative Commons license, and indicate if changes were made. The images or other third party material in this article are included in the article’s Creative Commons license, unless indicated otherwise in a credit line to the material. If material is not included in the article’s Creative Commons license and your intended use is not permitted by statutory regulation or exceeds the permitted use, you will need to obtain permission directly from the copyright holder. To view a copy of this license, visit http://creativecommons.org/licenses/by/4.0/ .
Reprints and permissions
About this article
Cite this article.
Li, M., Bralower, T.J., Kump, L.R. et al. Astrochronology of the Paleocene-Eocene Thermal Maximum on the Atlantic Coastal Plain. Nat Commun 13 , 5618 (2022). https://doi.org/10.1038/s41467-022-33390-x
Download citation
Received : 14 February 2022
Accepted : 15 September 2022
Published : 24 September 2022
DOI : https://doi.org/10.1038/s41467-022-33390-x
Share this article
Anyone you share the following link with will be able to read this content:
Sorry, a shareable link is not currently available for this article.
Provided by the Springer Nature SharedIt content-sharing initiative
By submitting a comment you agree to abide by our Terms and Community Guidelines . If you find something abusive or that does not comply with our terms or guidelines please flag it as inappropriate.
Quick links
- Explore articles by subject
- Guide to authors
- Editorial policies
Sign up for the Nature Briefing newsletter — what matters in science, free to your inbox daily.

- More from M-W
- To save this word, you'll need to log in. Log In
Definition of excursion
Did you know.
In Latin, the prefix ex- means "out of" and the verb currere means "to run." When the two are put together, they form the verb excurrere , literally "to run out" or "to extend." Excurrere gave rise not only to excursion but also to excurrent (an adjective for things having channels or currents that run outward) and excursus (meaning "an appendix or digression that contains further exposition of some point or topic"). Other words deriving from currere include corridor , curriculum , and among newer words, parkour .
Examples of excursion in a Sentence
These examples are programmatically compiled from various online sources to illustrate current usage of the word 'excursion.' Any opinions expressed in the examples do not represent those of Merriam-Webster or its editors. Send us feedback about these examples.
Word History
Latin excursion-, excursio , from excurrere
circa 1587, in the meaning defined at sense 1a
Theme music by Joshua Stamper ©2006 New Jerusalem Music/ASCAP
Get Word of the Day delivered to your inbox!
Dictionary Entries Near excursion
excursional
Cite this Entry
“Excursion.” Merriam-Webster.com Dictionary , Merriam-Webster, https://www.merriam-webster.com/dictionary/excursion. Accessed 11 May. 2024.
Kids Definition
Kids definition of excursion.
from Latin excursio, excursion- "a going out," from excurrere "to run out, make an excursion, extend," from ex- "out, forth" and currere "to run" — related to current
Medical Definition
Medical definition of excursion, more from merriam-webster on excursion.
Nglish: Translation of excursion for Spanish Speakers
Britannica English: Translation of excursion for Arabic Speakers
Subscribe to America's largest dictionary and get thousands more definitions and advanced search—ad free!
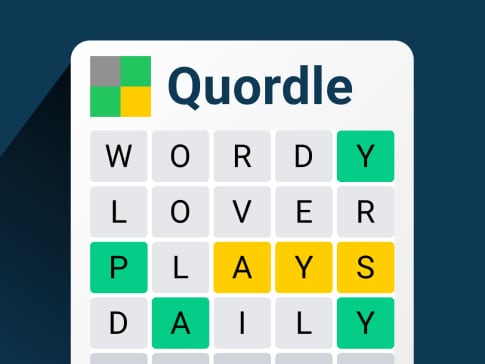
Can you solve 4 words at once?
Word of the day.
See Definitions and Examples »
Get Word of the Day daily email!
Popular in Grammar & Usage
More commonly misspelled words, your vs. you're: how to use them correctly, every letter is silent, sometimes: a-z list of examples, more commonly mispronounced words, how to use em dashes (—), en dashes (–) , and hyphens (-), popular in wordplay, the words of the week - may 10, a great big list of bread words, 10 scrabble words without any vowels, 8 uncommon words related to love, 9 superb owl words, games & quizzes.
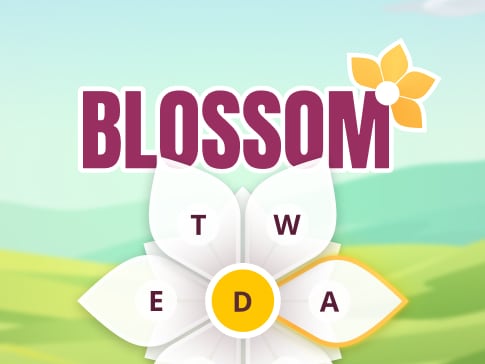
- Cambrex.com
- Client Login
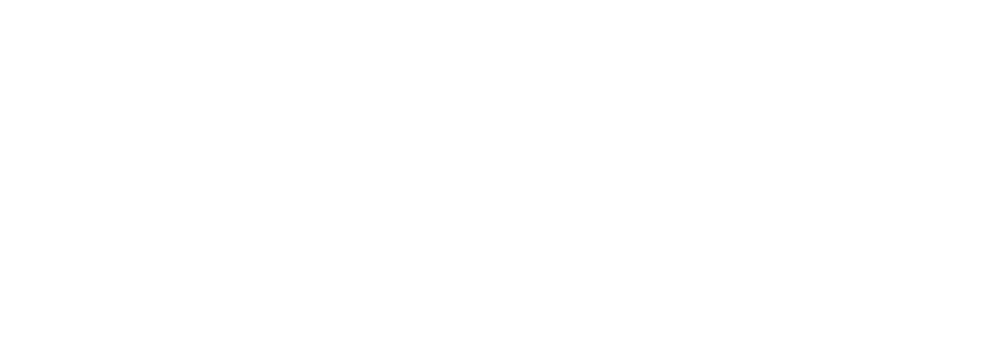
Temperature Excursion and Cycling Studies webinar
By Stephen Delaney
Register now for our live webinar: Temperature Excursion and Cycling Studies with guest speaker Dr. Mark Powell.
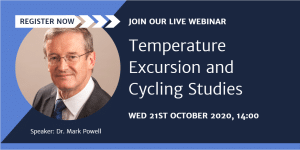
Temperature Excursion and Cycling Studies
Temperature excursions during shipment can cause the recall of drug product lots, leading to delays in supply and loss of revenue. Data from a well-conducted temperature excursion and/or cycling study can be used to justify such excursions. There is currently no harmonised guidance dealing with the impact of temperature excursions during distribution or the effect of freeze/thaw cycles on liquids and semi-solids. This webinar reviews best practice in these areas.
This webinar will cover:
- Overview of regulatory guidance
- Temperature excursion risks to physical and chemical product stability
- Evaluating possible excursions using supply chain knowledge
- Temperature excursion studies
- Thermal cycling studies
- Transportation control strategy

About the speaker Dr. Mark Powell

Mark has served as both Honorary Secretary and Honorary Treasurer of the RSC’s Analytical Division and led a working group on continuing professional development until July 2016. He has worked at a senior level in a number of companies with responsibility for analytical development and equipment qualification. In 2010 Mark was appointed Scientific Manager of a UK-based pharmaceutical CRO, with responsibility for guiding the direction of drug development programmes as well as establishing collaborations with academia and instrument manufacturers.
In 2013, he set up his own company to provide training and consultancy services to the pharmaceutical industry. His consultancy work has involved, amongst other things, managing the analytical aspects of pharmaceutical development programmes and conducting data integrity audits. He is in demand as a trainer in topics such as pharmaceutical development, chromatography, spectroscopy, dissolution testing, data integrity, control of impurities, technical writing, stability/stress studies and sample preparation.
Register now
To register for this webinar today, please visit our webinar registration page.
Is there anything additional that you would like us to cover during this webinar? If you have any questions, please contact us and we will answer your question during the Q&A session at the end of the webinar.
Did you miss our other webinars? Recordings are now available online for our previous webinars:
- Biopharmaceutical Stability Studies : Stability is a particular problem for biopharmaceutical products because the physical and chemical stability of peptides and proteins are often very sensitive to storage and transportation conditions. The purpose of this webinar is to explain the typical approach for the design of a stability study for biologics. The webinar also covers the specific challenges of these product types as well as typical degradation mechanisms.
- Stability Study Design – Bracketing and Matrixing : Stability programmes are a costly undertaking, especially for products that have multiple strengths and packaging configurations, where a full study design will require every configuration to be evaluated for three registration batches at each time point. The use of reduced study designs such as bracketing and matrixing can reduce the cost of formal stability studies. This webinar describes when the use of such approaches is allowable, together with their associated benefits and risks.
Privacy Overview
Necessary cookies are absolutely essential for the website to function properly. This category only includes cookies that ensures basic functionalities and security features of the website. These cookies do not store any personal information.
Any cookies that may not be particularly necessary for the website to function and is used specifically to collect user personal data via analytics, ads, other embedded contents are termed as non-necessary cookies. It is mandatory to procure user consent prior to running these cookies on your website.
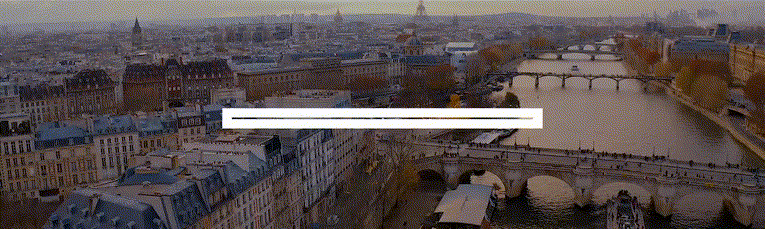
IMAGES
VIDEO
COMMENTS
Temperature excursion procedure should clearly define the situations that are covered by studies and in which a batch can be released and those where it cannot (see Figure 3). It should include a decision chart conducting the reader from the incoming temperature excursion information through the different questions that are relevant to the ...
Maintaining proper temperatures and avoiding excursions ensures that materials remain safe and effective. Meanwhile, according to the European Compliance Academy, a temperature excursion is defined as: "a deviation from the labeled storage condition of a product for any duration ". This includes excursions during transportation or distribution.
Patients use mail delivery as a convenient alternative to acquiring medications in person. Federal laws require nonspecialty oral medications to be stored at controlled room temperature during distribution; however, no laws or regulations govern temperature requirements for medication transport among patients, which may expose medications to harmful temperature excursions.
Thermal cycling studies should be conducted by taking several key factors into the consideration to assess the impact of the short-term exposure of drug product to conditions outside the intended storage temperature on product quality and stability and use this data to pre-define temperature excursion allowances and support potential ...
pectations related to the justification of temperature excursions, thermal cycling studies to assess and support potential tempera-ture excursions (including how/when thermal cycling study data is used to support temperature excursions), GDPs to minimize tem-perature excursions and use of theoretical methods/mathematical
Temperature excursion in pharmaceutical industry should be recorded and reported to the manufacturer for further investigation and risk analysis. The concept of temperature excursions, its reasons, consequences and handling mechanism should be well understood to ensure the concerted efforts under the aegis of Quality Management System.
RT was defined as 20 to 25 degrees Celsius (68-77 degrees Fahrenheit) based on the United States Pharmacopoeia's definition of "controlled RT." Refrigeration was defined as 2 to 8 degrees Celsius (36-46 degrees Fahrenheit). ... The data related to allowable RT excursions were completely unavailable for 32 products (or 13% of those approved ...
In this article, important aspects related to the key elements of a comprehensive temperature excursion management program are summarized, including standard stability testing, regulatory expectations related to the justification of temperature excursions, thermal cycling studies to assess and support potential temperature excursions (including ...
Download. The maintenance of cold chain product integrity across the entire supply chain demands rigorous processes and cold chain expertise of the highest caliber—from packaging, handling, storage and distribution of temperature sensitive Investigational Medicinal Products (IMP), all the way to the investigator site. Part 4 clearly outlines ...
The risk of temperature excursions cannot be ruled out, but it can be minimized through effective system. Alternative is to use thermal resistant packaging and stringent control measures during transit and shipment, to avert the undesired quality impact on pharmaceutical product. The systematic approach to handle the issues related temperature ...
when there is no control over the internal environment. MKT should not be used to justify regular excursions due to inadequate thermal control or poor operating procedures (e.g. load capacity of HVAC/refrigeration inadequate for the room or frequent excursions due to personnel mishandling of equipment). While USP makes these points in <1079.2>,
The Paleocene-Eocene Thermal Maximum (PETM) at 56 million years before present is arguably the best ancient analog of modern climate change. ... in fact, the excursion is now the formal definition of the boundary between the Paleocene and Eocene eras. Moreover, the excursion in terrestrial sediments allows us to correlate the changes that ...
The excursion study is followed by storage at the long-term storage condition • Patient convenience period needs to be included at the long-term storage condition, if applicable. A cycling approach (versus a block excursion) reduces the risk if multiple temperature excursions are experienced during the same shipment. Study Setup •
Multiple cycles may be included if desired. The duration of the cycle should mimic or exceed the maximum likely duration of a shipping excursion. The thermal cycling should be conducted at the beginning of shelf-life and the samples should be stored at the intended long-term storage conditions for the remainder of the shelf life.
Paleocene-Eocene Thermal Maximum. Part of the book series: Encyclopedia of Earth Sciences Series ( (EESS)) Approximately 55.5 Ma ago in marine and terrestrial sections all around the globe, there was an exceptionally large carbon isotope ( δ 13 C) excursion (CIE) which is unique in the Tertiary for its size and rapidity of its onset. This ...
Thermal profiling is the act of measuring several points on a circuit board to determine the thermal excursion it takes through the soldering process. In the electronics manufacturing industry, SPC (Statistical Process Control) helps determine if the process is in control, measured against the reflow parameters defined by the soldering ...
Pressurized Thermal Shock. Personnel need to be aware how pressure combined with thermal stress can cause failure of plant materials. This chapter addresses thermal shock (stress) with pressure excursions. Definition. One safety issue that is a long-term problem brought on by the aging of nuclear facilities is pressurized thermal shock (PTS).
A thermal cycling study is done to determine the impact of temperature excursion on the edge and outside of a product's labeled storage conditions. The excursion is simulated below and above the product's labeled storage conditions for multiple cycles, and the impact on the product quality will be evaluated because of thermal cycling.
Thermal excursion accelerating factors [GaAs reliability testing] Abstract: For more than a decade, the focus of GaAs reliability testing has been on high temperature life testing. Several failure mechanisms are highly accelerated by temperature, so this methodology has produced data that is easy to analyze and straightforward to predict ...
Changepoint analysis and the definition of the carbon isotope excursion onset. ... Ridgwell, A., Sluijs, A. & Thomas, E. Onset of carbon isotope excursion at the Paleocene-Eocene thermal maximum ...
excursion where the product is exposed to multiple temperature stress conditions. Since international distribution of drug product may include multiple distribution legs, thermal-cycling studies should be designed with a minimum of three cycles to support cumulative exposure periods from the entire distribution process.
thermal. adj. 1 (Also) thermic of, relating to, caused by, or generating heat or increased temperature. 2 hot or warm. thermal baths, thermal spring. 3 (of garments or fabrics) specially designed so as to have exceptional heat-retaining properties. n.
excursion: [noun] a going out or forth : expedition. a usually brief pleasure trip. a trip at special reduced rates.
Temperature excursions during shipment can cause the recall of drug product lots, leading to delays in supply and loss of revenue. Data from a well-conducted temperature excursion and/or cycling study can be used to justify such excursions. ... Temperature excursion studies; Thermal cycling studies; Transportation control strategy; About the ...